Background
Clinical two-dimensional (2D) and clinical three-dimensional echocardiography are validated against cardiac magnetic resonance imaging (CMR), the gold standard for left ventricular (LV) volume measurement. In rodents, there is no widely accepted echocardiographic measure of whole LV volumes, and CMR measurements vary among studies. The aim of this study was to compare LV volumes by 2D echocardiography (using a hemisphere-cylinder [HC] model) with HC and full-volume (FV) CMR in normal and diseased rats to measure the impact of geometric models and imaging modalities.
Methods
Rats ( n = 27) underwent ascending aortic banding, myocardial infarction induction by either permanent left anterior descending coronary artery ligation or ischemia-reperfusion, and sham thoracotomy. Subsequently, end-diastolic volume, end-systolic volume, and ejection fraction were measured using an HC 2D echocardiographic model combining parasternal short-axis and long-axis measurements, and these were compared with HC and FV CMR.
Results
Diseased groups showed LV dilatation and dysfunction. HC echocardiographic and FV CMR measures of end-diastolic volume, end-systolic volume, and ejection fraction were correlated. On Bland-Altman plots, end-diastolic volumes were concordant between both methods, while HC echocardiography underestimated end-systolic volumes, resulting in a modest overestimation of ejection fractions compared with FV CMR. Other 2D echocardiographic geometric models offered less concordance with FV CMR than HC. HC CMR overestimated LV volumes compared with FV CMR, while HC echocardiography underestimated HC CMR volumes. Echocardiography underestimated corresponding LV dimensions by CMR, particularly short axis.
Conclusions
Concordant measures of LV volume and function were obtained using (1) a relatively simple HC model of the left ventricle inclusive of two orthogonal 2D echocardiographic planes and (2) FV CMR in normal and diseased rats. The HC model appeared to compensate for the underestimation of LV dimensions by echocardiography.
Echocardiography and cardiac magnetic resonance imaging (CMR) measure left ventricular (LV) volumes and function. In humans, full-volume (FV) CMR is the gold standard for LV size and function because of its ability to obtain three-dimensional (3D) images of the left ventricle and thus measure LV volumes without geometric assumptions. In small animals, FV CMR is gaining momentum for similar reasons.
Both echocardiography and CMR are complementary techniques with advantages and disadvantages, but little is known about the comparability of their volume measurements in rodents and their comparative ability to detect small and large variations in LV size and function.
On the other hand, several published echocardiographic indicators of LV dimension used in rodents rely exclusively on a single parasternal short-axis or long-axis view, with or without M-mode imaging, and often derive LV volumes from unidimensional measures.
The need for 3D measurements of LV size and function using rodent echocardiography has been understood for a long time, but no approach is recognized as standard and optimal in that regard. Real-time 3D echocardiography is now widely used in humans ; 3D echocardiography has been successfully performed in rodents but remains of limited availability and applicability, with some technical challenges such as the need for gated imaging. Besides, real-time 3D echocardiography requires matrix-array transducers, which, to our knowledge, are not developed for use in small animals. Real-time 3D echocardiography is still constrained by lower spatial resolution and frame rate compared with two-dimensional (2D) echocardiography, which further limits its application to small animals. Thus, for imaging in small animals, it remains useful to determine the most suitable 2D echocardiography-based geometric model to determine LV volumes.
Picard et al. reviewed and discussed the importance of 3D echocardiography and the limitations of geometric models of LV volumes from 2D echocardiographic views. One such measure of LV volumes uses the “bullet formula,” or hemisphere-cylinder (HC) model, as reported in rat studies using echocardiography and CMR. This geometric model has the theoretical advantage of including three dimensions of the left ventricle by combining cross-sectional area of the parasternal short-axis view (two dimensions) with cavity length from the parasternal long-axis view (a third perpendicular dimension); it was validated in dogs and recommended for echocardiographic LV volume and LV mass calculation in humans. Another advantage of the HC model is its entire reliance on parasternal views, which are easier to obtain with the high-resolution linear-array probes commonly used in rodents and less subject to foreshortening than apical views in rodents.
We compared LV volumes by 2D echocardiography in rats using an HC model with HC and FV CMR in two disease models of rat LV dysfunction and dilatation of graded severity.
Methods
Animal Use and Care
Animals were obtained and handled as approved by the Institutional Animal Care and Use Committee of the Icahn School of Medicine at Mount Sinai in accordance with the “Principles of Laboratory Animal Care by the National Society for Medical Research and the Guide for the Care and Use of Laboratory Animals” (National Institutes of Health Publication No. 86-23, revised 1996).
Surgical Animal Disease Models
We studied 27 rats. Male Sprague-Dawley rats (200–250 g) underwent surgery as previously described. Pressure-overload LV hypertrophy and failure was induced by ascending aortic banding ( n = 4), and myocardial infarction (MI) was created by permanent left anterior descending coronary artery ligation ( n = 9) or left anterior descending coronary artery ligation for 30 min followed by reperfusion ( n = 5). Sham animals underwent thoracotomy without MI or aortic constriction ( n = 4), and normal animals ( n = 5) of similar age and weight at the time of imaging were used.
One to 2 months after surgery, animals underwent consecutive echocardiography and CMR by double-blinded operators in random order. The time interval between CMR and echocardiography on the same animal was 0 ± 5 days.
Echocardiography
Echocardiography was performed under sedation by intraperitoneal ketamine up to 80 mg/kg, with starting doses as low as 10 mg given to diseased rats and supplemented by additional injections until optimal sedation was obtained. Sedation was optimized by giving the lowest dose of ketamine needed to (1) restrain the animal and prevent motion artifacts and (2) maintain the heart rate in the range of 350 to 450 beats/min. Ketamine was chosen on the basis of our previous experience and considering that alternative agents had long durations of action (pentobarbital), were potentially unsafe for heart failure animals, or had bradycardic effects (isoflurane, ketamine/xylazine), as demonstrated elsewhere. The chest was shaved.
Short-axis parasternal views of the left ventricle at the midpapillary level and long-axis parasternal views of the left ventricle were obtained using a Vivid i echocardiographic apparatus with a 13-MHz linear-array probe (GE, New York, NY) at a frame rate ranging from 25 to 28 frames/sec for 2D echocardiography. Volumes of the LV cavity at end-diastole and end-systole were calculated using an area-length HC formula that assumes a bullet-shaped left ventricle, as previously recommended and described. LV end-diastolic volume (EDV) and end-systolic volume (ESV) were thus calculated as V = 5/6 × A × L , where V is the volume of the LV cavity in milliliters, A is the cross-sectional area of the LV cavity in square centimeters obtained from a parasternal short-axis image at the midpapillary level, and L is the length of the LV cavity in centimeters measured as the distance from the endocardial LV apex to the mitral-aortic junction on the parasternal long-axis image, as previously described. Additional planimetry was performed on the parasternal long-axis view to obtain LV volumes (1) by application of a modified Simpson’s rule (programmed in the software package of the ultrasound device) and (2) by measurement of the area A and length L of the echocardiographic long-axis modeled as a single plane ellipsoid in which LV volume = (8 A 2 )/(3π L ), as previously published.
M-mode echocardiography was performed on short-axis parasternal views as previously recommended to obtain LV end-diastolic and end-systolic diameters. The frame rate for M-mode echocardiography was 48 frames/sec.
For 2D and M-mode LV cavity measurements, care was taken to exclude papillary muscles from the LV wall.
CMR
Rats were sedated with 1.5% to 2% isoflurane in oxygen (0.6 L/min), consistent with previous reports. The respiratory rate was monitored using a magnetic resonance–compatible monitoring system (SA Instruments, New York, NY) and maintained in the range of 55 to 70 breaths/min.
A 7-T horizontal-bore animal magnetic resonance scanner (Bruker BioSpin GmbH, Ettlingen, Germany) with an 86-mm radiofrequency volume coil (Bruker BioSpin GmbH) and Paravision 5.1 software was used. After axial, sagittal, and coronal localizer sequences were obtained, the coil was tuned and matched. Then, bright-blood cine images of two-chamber, three-chamber, and four-chamber views of the left ventricle were obtained using a retrospectively self-gated protocol (IntraGate; Bruker BioSpin GmbH). Acquisition parameters were as follow: echo time, 2.96 msec; repetition time, 9.8 msec; flip angle, 15∞; field of view, 50 × 50 mm 2 ; matrix size, 256 × 256; number of repetitions, 200; cardiac frames reconstructed, 20; slice thickness, 1 mm; and scan time, approximately 3 min.
FV imaging was also acquired using a retrospectively self-gated protocol with a 3D imaging volume (3D IntraGate; Bruker BioSpin GmbH) and the additional acquisition of navigator echoes. The short-axis orientation was defined using the three-chamber and four-chamber views, as shown in Figure 1 . The navigator slice was placed above the 3D imaging package that covered the LV cavity from base to apex. Sequence parameters were as follows: radiofrequency pulse, 1-msec sinc pulse (10 lobes; bandwidth, 20 kHz); slab thickness, 10 mm, 10 mm; field of view, 50 × 50 × (18−14) mm 3 ; Nx × Ny × Nz, 128 × 128 × (12−9); spatial resolution, 0.312 × 0.312 × 1.5 mm/pixel; echo time, 2 msec; repetition time, 8 msec; number of repetitions, 50; flip angle α, 14∞; on the navigator slice: radiofrequency pulse, 1-ms Gauss pulse (bandwidth, 2.740 kHz); slice thickness, 3.9 mm; flip angle α nav , 8∞. No more than 70% of all k-lines were used for the reconstruction of 16 cardiac time frames to avoid artifacts from respiratory movement. Total scan time was about 8 to 10 min (Ny × Nz × repetition time/number of repetitions). The effective number of averages was approximately 2.5 (70% × [number of repetitions]/6).

Analysis of global LV function was calculated using Segment version 1.9 (Medviso, Stockholm, Sweden). Myocardium segmentation was performed among at least seven LV short-axis slices outlining both endocardial and epicardial borders in all cardiac frames ( Figure 1 ). EDV and ESV were calculated from the largest and smallest areas, respectively, of the LV cavity in each slice. Apical and basal slices without semicircular muscular ring at either end-systole or end-diastole were disregarded. Papillary muscles were not included in the LV wall, and the LV outflow tract was not included in the LV cavity in FV measurements.
Additional analysis of the CMR images included measurements similar to the ones performed by echocardiography on the long-axis and midpapillary short-axis views, obtained using the CMR localizer sequences, and used to derive CMR volumes by the HC formula, as well as LV diameters from the short-axis midpapillary view (see the “Echocardiography” section for details).
Animal Group Assignment
Animals were divided into five groups: normal rats, sham-operated rats (thoracotomy, no MI, and no pressure-overload LV hypertrophy), pressure-overload LV hypertrophy rats, rats with apical MI visible on all echocardiographic and CMR views, and rats with small apical MI (visible on some but not all echocardiographic and/or CMR views).
Statistical Analysis
Comparison of continuous variables between animal groups used analysis of variance (ANOVA) followed by post hoc pairwise comparisons using Student’s t test with Bonferroni’s correction for multiple testing. Concordance between LV volumes and ejection fraction (EF) by echocardiography versus CMR was measured using paired t tests, Pearson’s correlation on scatterplots, and Bland-Altman plots. Linear regression of between-methods difference versus among-methods average was performed on the Bland-Altman plot to test the hypothesis that the difference between the two methods did not vary across the range of measured values. Concordance between methods was quantified separately in diseased ( n = 18) and nondiseased (normal and sham, n = 9) animals. Repeated-measures ANOVA was used to compare sequential echocardiography and CMR-derived parameters across groups, for the purpose of testing a method-group interaction. This interaction tests the differential ability of the two methods to distinguish groups. Results are expressed as mean ± SD. P values <.05 were considered statistically significant. Stata version 10.1 (StataCorp LP, College Station, TX) was used for statistical analyses.
Results
Heart Rate During Imaging
Heart rate per animal group is shown in Table 1 . It was significantly higher on echocardiography compared with CMR (394 ± 53 vs 294 ± 54 beats/min; P < .0001, paired t test).
Group | EDV (μL) | ESV (μL) | EF (%) | HR (beats/min) | |||||||
---|---|---|---|---|---|---|---|---|---|---|---|
HC echo | HC CMR | FV CMR | HC echo | HC CMR | FV CMR | HC echo | HC CMR | FV CMR | Echo | CMR | |
Apical MI ( n = 11) | 706 ± 160 ∗ | 1,250 ± 228 | 729 ± 94 ‡||# | 262 ± 129 ∗§ | 491 ± 80 ‡¶∗∗‡‡ | 328 ± 77 ‡¶#†† | 64 ± 13 †§ | 60 ± 6 †¶∗∗ | 55 ± 10 ∗§ | 403 ± 53 | 284 ± 53 |
POH ( n = 4) | 666 ± 149 | 1,031 ± 137 ( n = 3) | 553 ± 52 | 158 ± 109 | 195 ± 26 ( n = 3) | 197 ± 87 | 78 ± 12 | 81 ± 4 ( n = 3) | 65 ± 13 | 324 ± 44 | 309 ± 41 |
Small apical MI ( n = 3) | 560 ± 187 | 1,022 ± 108 | 601 ± 54 | 165 ± 75 | 318 ± 17 | 178 ± 41 | 71 ± 3 | 69 ± 3 | 71 ± 4 | 404 ± 38 | 298 ± 78 |
Normal ( n = 5) | 509 ± 119 | 1,045 ± 179 | 532 ± 92 | 82 ± 23 | 244 ± 60 | 134 ± 62 | 84 ± 3 | 77 ± 3 | 75 ± 12 | 404 ± 50 | 271 ± 59 |
Sham ( n = 4) | 409 ± 41 | 953 ± 36 ( n = 3) | 453 ± 89 | 44 ± 18 | 249 ± 5 ( n = 3) | 119 ± 37 | 89 ± 5 | 74 ± 1 ( n = 3) | 74 ± 9 | 417 ± 36 | 332 ± 47 |
P (ANOVA) | .013 | .079 | .0001 | .005 | <.0001 | <.0001 | .002 | <.0001 | .006 | .069 | .50 |
Concordance and Correlation of LV Volumes and EF by HC Echocardiography and CMR (HC CMR and FV CMR)
Representative images of long-axis and short-axis views of the left ventricle by echocardiography and CMR are shown in Figure 2 , from the same sham and post-MI animals, respectively. LV volumes (EDV and ESV) and EF measured by HC echocardiography, HC CMR, and FV CMR are shown in Table 1 . Diseased groups demonstrated variable LV dilatation and dysfunction, most significant in the apical MI group ( Table 1 ).

Agreement between HC echocardiography and FV CMR for the measurement of EDV, ESV, and EF was assessed using paired t tests ( Table 2 ), Bland-Altman plots ( Figure 3 ), and linear regression (scatterplots; Figure 4 ).
Group | EDV (μL) | ESV (μL) | EF (%) | ||||||
---|---|---|---|---|---|---|---|---|---|
HC echo | HC CMR | FV CMR | HC echo | HC CMR | FV CMR | HC echo | HC CMR | FV CMR | |
Diseased ( n = 18) | 673 ± 161 † | 1,171 ± 220 ( n = 17) ‡ | 669 ± 111 | 223 ± 123 † | 408 ± 137 ( n = 17) ‡ | 274 ± 100 | 68 ± 13 ‡ | 65 ± 9 ( n =17) ¶ | 60 ± 11 |
Normal and sham ( n = 9) | 464 ± 102 † | 1,011 ± 144 ( n = 8) ‡ | 497 ± 94 | 65 ± 28 ||† | 246 ± 45 ( n = 8) ‡ | 127 ± 50 | 86 ± 5 ∗§ | 76 ± 3 ( n = 8) | 74 ± 10 |
Overall ( n = 27) | 603 ± 174 † | 1,120 ± 210 ( n = 25) ‡ | 611 ± 133 | 170 ± 126 †§ | 356 ± 138 ( n = 25) ‡ | 225 ± 111 | 74 ± 14 ∗|| | 69 ± 9 ( n = 25) ¶ | 65 ± 13 |
Overall, HC echocardiography and FV CMR gave concordant measures of EDV ( Table 2 , Figure 3 ). The average difference in EDV between HC echocardiography and FV CMR was −8 ± 123 μL.
Compared with FV CMR, HC echocardiography underestimated ESV across its entire range, with an average difference between HC echocardiography and FV CMR of −55 ± 88 μL ( Table 2 , Figure 3 ). This resulted in echocardiography overestimating EF by 10 ± 12% ( Table 2 , Figure 3 ).
Agreement between Volume Measurement: Differential Impact of Imaging Modalities and Geometric Models
Compared with FV CMR, HC CMR overestimated EDV and ESV ( Table 2 ). Moreover, HC echocardiography underestimated volumes compared with HC CMR, indicating that the HC model per se overestimates LV volumes compared with the FV model used as a reference, while echocardiography per se may underestimate LV volumes compared with CMR. EF was significantly different among the three methods ( Table 2 , Figure 3 ).
LV Dimensions by Echocardiography Underestimated Corresponding CMR Dimensions
This underestimation was major for the short-axis area ( Table 3 ) and for LV diameters of the midpapillary short-axis view (data not shown). Short-axis diameters were consistently and severely underestimated by M-mode echocardiography compared with CMR short-axis diameters as well (data not shown).
Group | Short-axis area (cm 2 ) | Long-axis length (cm) | ||||||
---|---|---|---|---|---|---|---|---|
End-diastole | End-systole | End-diastole | End-systole | |||||
Echo | CMR | Echo | CMR | Echo | CMR | Echo | CMR | |
Diseased ( n = 18) | 0.46 ± 0.10 † | 0.78 ± 0.11 | 0.17 ± 0.09 † | 0.31 ± 0.09 | 1.77 ± 0.11 | 1.80 ± 0.13 ( n = 17) | 1.57 ± 0.17 | 1.59 ± 0.19 ( n = 17) |
Normal and sham ( n = 9) | 0.35 ± 0.06 † | 0.68 ± 0.08 | 0.06 ± 0.03 † | 0.23 ± 0.05 | 1.56 ± 0.13 † | 1.73 ± 0.13 ( n = 8) | 1.20 ± 0.10 | 1.20 ± 0.09 ( n = 8) |
Overall ( n = 27) | 0.42 ± 0.10 † | 0.75 ± 0.11 | 0.13 ± 0.09 † | 0.28 ± 0.09 | 1.70 ± 0.15 ∗ | 1.77 ± 0.13 ( n = 25) | 1.45 ± 0.23 | 1.46 ± 0.25 ( n = 25) |
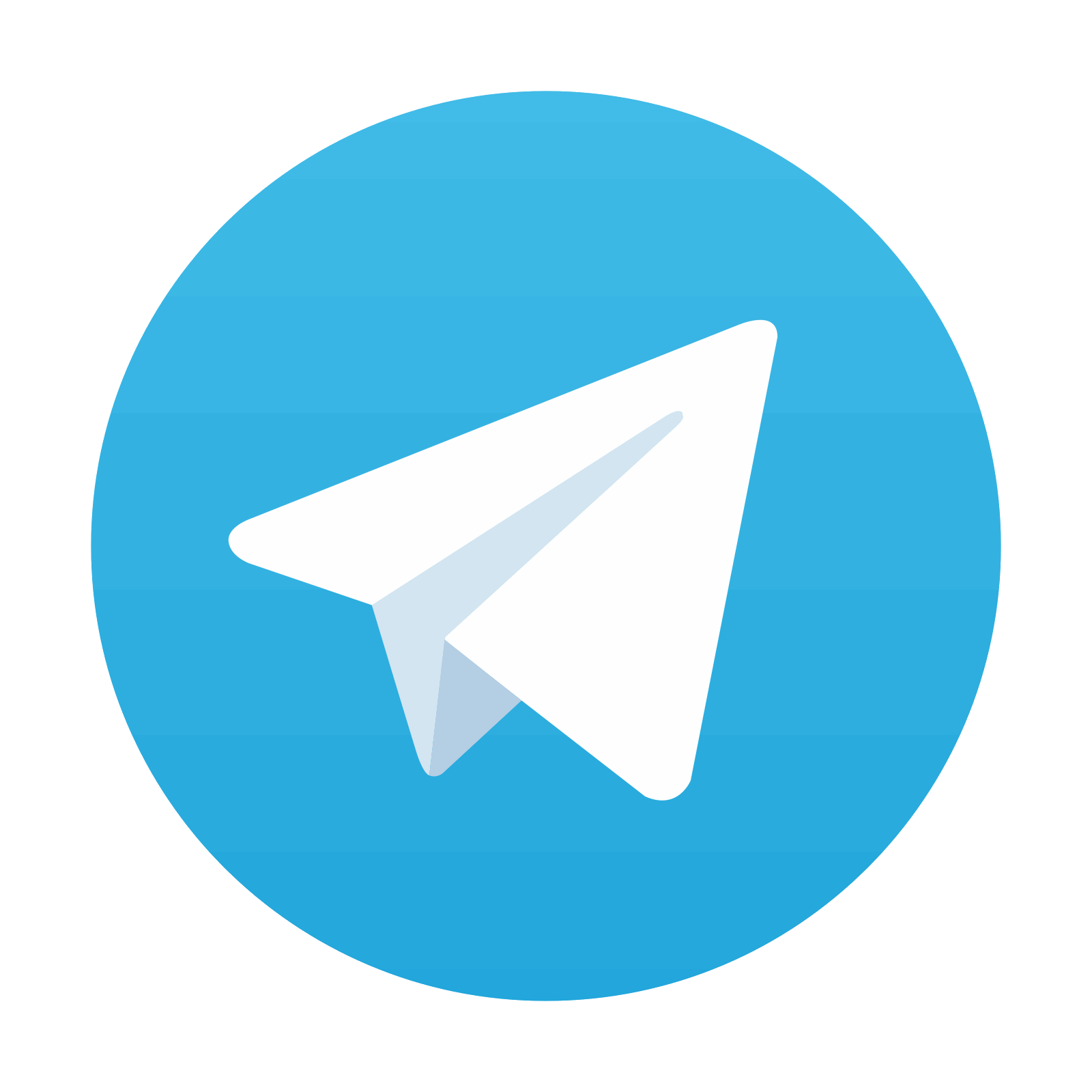
Stay updated, free articles. Join our Telegram channel

Full access? Get Clinical Tree
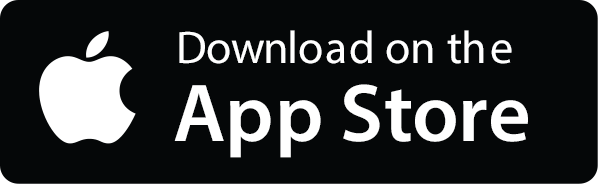
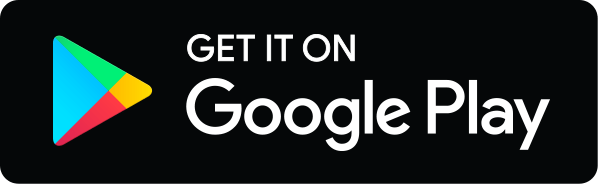
