Direct planimetry of anatomic regurgitation orifice area (AROA) using 3-dimensional transesophageal echocardiography (TEE) has been described. This study sought to (1) compare mitral valve regurgitant volume (RV) derived by AROA using 3-dimensional TEE with RV obtained by cardiac magnetic resonance (CMR) imaging and (2) determine the impact of AROA and flow velocity changes throughout systole on the dynamic variation in mitral regurgitation. In 43 patients (71 ± 11 years old) with mild to severe mitral regurgitation, 3-dimensional TEE and CMR were performed. Mitral valve RV was determined based on (1) AROA at 5 subintervals of systole and analysis of the regurgitant continuous-wave Doppler signal at equal durations of systole, (2) effective regurgitation orifice area (EROA) using the proximal isovelocity surface area method, (3) CMR with subtraction of aortic outflow volume from left ventricular stroke volume. RV calculated by AROA tended to overestimate RV less than RV calculated by EROA compared to RV by CMR (average bias +20 ml, 95% confidence interval [CI] −41 to +81, vs +13 ml, 95% CI −22 to 47). In patients with RV >30 ml by CMR, overestimation of RV using the AROA method was less than using the EROA method (difference in means +18 ml, 95% CI 4 to 32, p <0.001). AROA determined by 3-dimensional TEE varied by only 18% among the 5 subintervals of systole, and the velocity time integral of the subinterval with the highest flow was 120% of the subinterval with the lowest flow. In conclusion, 3-dimensional TEE allows accurate analysis of mitral valve RV. In the clinically relevant group of patients with RV >30 ml as defined by CMR, the AROA method results in less overestimation of RV than the EROA method. Changes in AROA during systole contribute much less to dynamic variation in mitral regurgitation severity than changes in regurgitant flow velocity.
This study sought to (1) compare regurgitant volume (RV) derived from anatomic regurgitant orifice area (AROA) determined by real-time 3-dimensional transesophageal echocardiography (TEE) and RV derived from effective regurgitation orifice area (EROA) using the proximal isovelocity surface area method with RV derived from cardiac magnetic resonance (CMR) used as a reference method and (2) obtain a better understanding on the impact of AROA and regurgitant flow velocity changes throughout systole on the dynamic variation in mitral regurgitation.
Methods
We enrolled 46 consecutive patients with 1 to 4+ mitral regurgitation defined by routine color Doppler echocardiography. Patients with significant mitral stenosis (mitral valve area <2.0 cm 2 ), mitral prosthesis, irregular rhythm or aortic regurgitation grade >1 were not included. Because of impaired 2-dimensional transesophageal echocardiographic image quality, 3 patients were excluded. In the remaining 43 patients (95%) color Doppler TEE, real-time 3-dimensional TEE, and CMR were performed within a short interval on the same day. This study was approved by the institutional review board of the University Clinic Aachen and all patients provided written informed consent.
Echocardiographic studies were performed with a commercially available echocardiographic system (iE 33, Philips Medical Systems, Andover, Massachusetts) and a transesophageal echocardiographic probe (X7-2 t), allowing real-time 3-dimensional TEE. Color Doppler flow TEE was performed using a multiplane transesophageal echocardiographic probe in the midesophageal position with a scanning plane of 0° to achieve a 4-chamber view of the mitral valve. The narrowest sector angle that allowed visualization of the mitral regurgitation proximal convergence field was used to maximize color flow imaging frame rate. Nyquist velocity ranged from 39 to 70 cm/s. In each patient, a sweep of the mitral valve coaptation line was performed with the transesophageal echocardiographic probe to find the location with the largest regurgitation jet and optimize visualization of the area of proximal flow acceleration and the downstream expansion of the jet.
Zoom mode was used to optimize visualization. Appearance of the proximal convergence field was optimized by baseline shifting of the color Doppler aliasing velocity to 30 to 39 cm/s. The radial distance from the first aliasing contour (red/blue interface) to the center of the regurgitant orifice was measured on the image with the largest convergence zone. For patients with nonflail mitral leaflets, the orifice was assumed to be at the plane passing through the tips of the mitral leaflets; for flail mitral leaflets, the orifice was assumed to lie in the plane of the nonflail leaflet. Maximal instantaneous regurgitant flow was calculated as 2r 2 v a , where r is the maximal radial distance to the contour of the aliasing velocity, v a , with a hemispheric contour assumed. For calculation of mitral RV the regurgitant continuous-wave Doppler signal was obtained. EROA was obtained by dividing maximal flow by peak regurgitant velocity obtained by continuous-wave Doppler (EROA = maximal instantaneous regurgitant flow/peak regurgitant velocity). RV was calculated by multiplying EROA by the velocity time integral obtained by continuous-wave Doppler (RV = EROA × velocity time integral).
Real-time 3-dimensional TEE was based on full-volume wide-angle acquisition of the mitral valve. Three-dimensional transesophageal echocardiographic data acquisition was performed over 4 heart cycles during a short period of breath-hold to avoid stitching artifacts with typical volume rates of 24 to 28 per second. Analysis was done off-line with commercially available software (3DQ, QLAB 7.0, Philips Medical Systems). The cropping tool allowed adjustment of 2 known views to help with orientation. These 2 planes were cropped to an intercommissural view and a left ventricular outflow tract view. Orthogonal to these 2 helping views, a third plane was placed in short-axis view at the level of the commissural line of the mitral valve. AROA was visualized in this cross-sectional view where direct planimetry of the regurgitant orifice could be performed ( Figure 1 ).
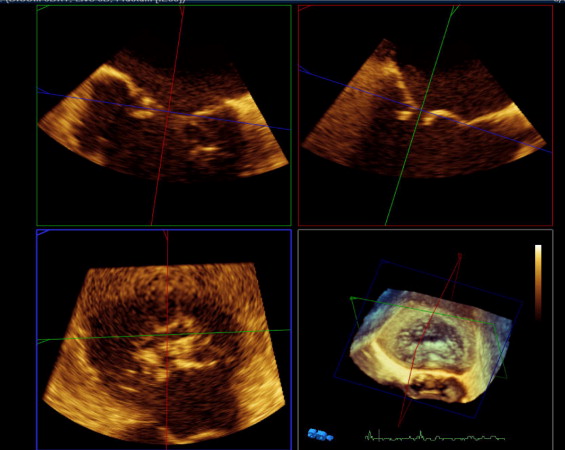
To adjust for possible changes in AROA during systole, AROA was determined 5 times during systole with the duration of systole being divided into 5 equally long subintervals. For calculation of mitral RV, the regurgitant continuous-wave Doppler signal was obtained. Five subintervals of equal duration of the regurgitant continuous-wave Doppler signal were defined. For each subinterval, the velocity time integral was determined. RV was calculated as the sum of the 5 products of AROA and corresponding velocity time integral (VTI) as Σ 5 i = 1 (AROA i × VTI i ) ( Figure 2 ).
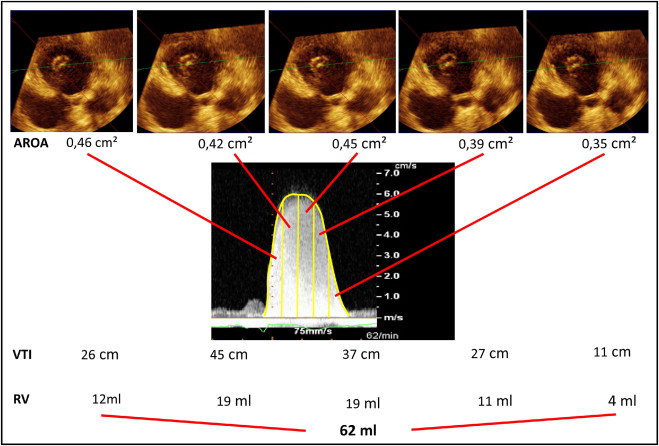
CMR imaging was performed using a 1.5-T MR scanner (Achieva, Philips Healthcare, Best, The Netherlands) equipped with a 5-element cardiac synergy coil for signal reception and a vector electrocardiograph for cardiac synchronization. Image acquisition was performed during short repetitive end-expiratory breath-holding. Left ventricular function was assessed using balanced turbo field echocardiographic cine imaging with retrospective gating (repetition time 3.3 ms, echocardiographic time 1.6 ms, flip angle 60°, 50 phases per cardiac cycle, spatial resolution 1.5 × 1.5 × 8.0 mm 3 ) in short-axis orientation with full ventricular coverage. Aortic outflow volume was assessed using quantitative flow measurements (repetition time 4.1 ms, echocardiographic time 2.5 ms, flip angle 15°, 35 phases per cardiac cycle, spatial resolution 1.4 × 1.4 × 10 mm 3 ), which were performed 10 mm above the tip of the aortic valve perpendicular to the aorta. Velocity encoding was adapted individually to avoid aliasing.
Image analysis was performed using an off-line workstation (Extended Workspace, Philips Healthcare). Left ventricular end-diastolic and end-systolic volumes were determined from short-axis cine images according to the disk summation method (Simpson rule) and left ventricular stroke volume was calculated by subtracting left ventricular end-systolic from left ventricular end-diastolic volume. Aortic outflow volume was derived from quantitative flow measurements using the semiautomatic built-in tool. Mitral regurgitation volume was calculated by subtracting systolic aortic outflow volume from left ventricular stroke volume.
Statistical analysis was performed with a special statistical analysis program (MedCalc 9.5.1.0, MedCalc, Mariakerke, Belgium). Continuous data are presented as mean ± SD and categorical data are presented as frequency. RVs determined by AROA and by EROA were compared to mitral RV determined by CMR using linear regression analysis and Bland–Altman analysis of agreement for the entire population and a subgroup of patients with RV >30 ml. Pearson correlation coefficient (r) with p value and 95% confidence interval (CI) for radial distance was calculated. A p value <0.05 was considered statistically significant.
Results
Clinical characteristics of the 46 patients with 1 to 4+ mitral regurgitation are presented in Table 1 . Most mitral regurgitation was due to functional mitral regurgitation, although prolapse or flail leaflet of the mitral valve and rheumatic disease were other common causes. RVs determined by the 3 methods were as follows: RV by EROA 51.5 ± 36.3 ml, RV by AROA 44.7 ± 17.7 ml, and RV by CMR 32.0 ± 15.3 ml. Using only the largest AROA instead of the 5 subinterval AROAs for calculation of RV based on 3-dimensional TEE resulted in an RV of 52 ± 21 ml. Therefore, subintervals of systole were analyzed and RVs were calculated for 5 subintervals of velocity time integral and AROA. Although RVs determined by EROA and AROA were larger than those obtained by CMR, RVs determined by EROA tended to overestimate reference RVs even more than those obtained by the AROA method. Average bias was +20 ml (95% CI −41 to 81 for EROA-derived RVs compared to +13 ml, 95% CI −22 to 47 for AROA-derived RVs; Figure 3 ). Considering only patients with mitral regurgitation because of flail or rheumatic cause, RVs were 81 ± 41 ml using the EROA and 58 ± 18 ml using the AROA method. Considering only patients with functional mitral regurgitation, RVs were 34 ± 16 ml by EROA and 36 ± 12 ml by AROA.
Age (years) | 71 ± 13 |
Men/women | 17/26 |
New York Heart Association class | |
I | 3 (7%) |
II | 15 (35%) |
III | 21 (49%) |
IV | 4 (9%) |
Ejection fraction by cardiac magnetic resonance (%) | 52 ± 16 |
Diastolic left ventricular volume by cardiac magnetic resonance (ml) | 182 ± 52 |
4-Chamber left atrial area (cm 2 ) | 27.9 ± 6.4 |
4-Chamber color Doppler jet area (cm 2 ) | 8.9 ± 2.8 |
Mitral valve regurgitation severity | |
Mild | 12 (26%) |
Moderate | 19 (41%) |
Severe | 12 (26%) |
Cause of mitral regurgitation | |
Functional ischemic heart disease/dilated cardiomyopathy | 17 (40%) |
Mitral valve prolapse/flail leaflet | 15 (35%) |
Rheumatic heart disease/others | 11 (25%) |
Mild aortic regurgitation (grade 1) | 11 (24%) |
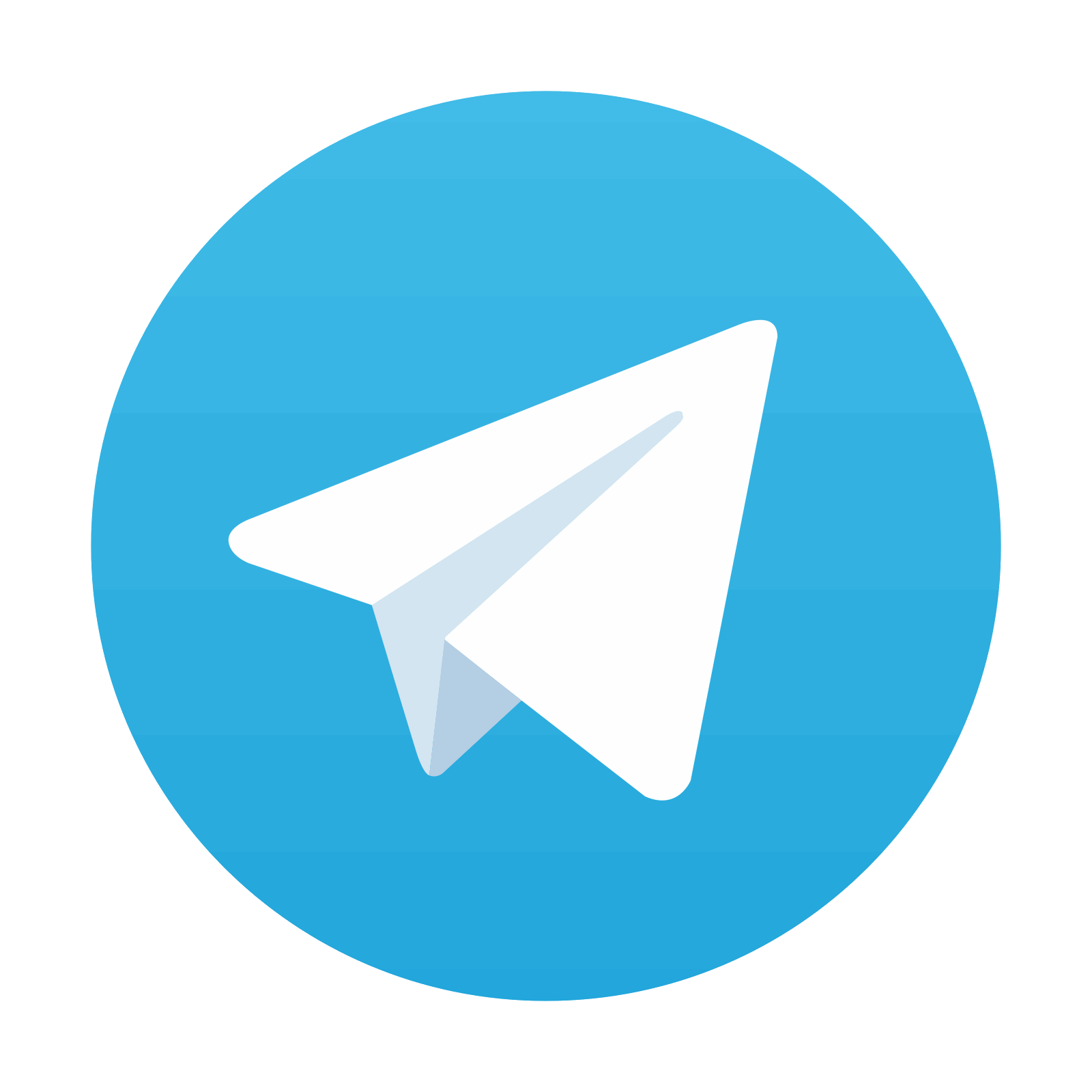
Stay updated, free articles. Join our Telegram channel

Full access? Get Clinical Tree
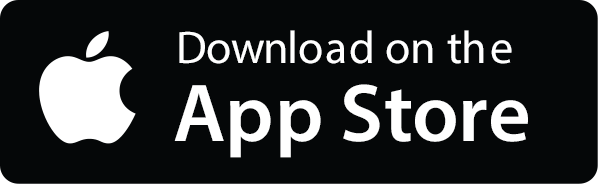
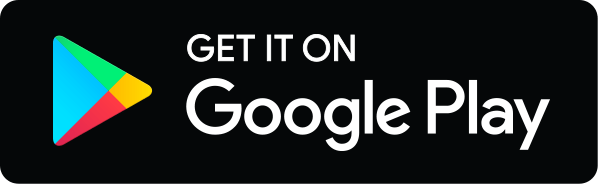
