Common Genetic Issues in Cardiovascular Disease
Mary T. Quinn Griffin
Saif Anwaruddin
Genetic abnormalities have been associated with all types of cardiovascular disease, including coronary atherosclerosis, rhythm disorders, aortic disease, and structural heart disease. Since the initial descriptions of familial hyperlipidemia (FH) and the mutations in the low-densitylipoprotein (LDL) receptor (LDL-R), genetic studies have fostered an improved understanding of the underlying pathophysiology of various cardiovascular disease states. Furthermore, the sequencing of the human genome has ushered in the era of cardiovascular genomics. The ability to efficiently scour through the massive amount of genomic information will ultimately result in an improved understanding of the contributions of genetics to cardiovascular disease.
This understanding would potentially lead to an improved ability to accurately diagnose disease, prevent progression, and risk stratify at the individual level. Furthermore, this information will add to our understanding of the relationship between DNA variants and the response to drugs or other treatment modalities. The pharmacogenomic profiles developed may provide a refined approach to treatment with less toxicity. A more comprehensive assessment of future risk for both patient and potentially affected family members would also be feasible.
Although there are some examples within cardiovascular disease of simple monogenic disorders explained by principles of Mendelian inheritance, many of the entities, such as coronary atherosclerosis, acute myocardial infarction (MI), and atrial fibrillation (AF), are complex traits with multiple genes contributing to the phenotype. As opposed to Mendelian disorders, which are deterministic, complex traits are probabilistic.
It will require extensive time and effort to be able to define all the variations in all the genes that contribute to the susceptibility to or protection from a complex trait. Furthermore, the simple identification of genes involved does not address the issue of gene—gene and gene-environment interactions influencing complex traits. There have been extensive recent reports of genomic variants associated with risk of diseases. These variants are common, often accounting for 20% to 30% of the population attributable risk, but with an odds ratio of 1.2 or 1.3, for example, only 20% to 30% excess risk. These are common variants for common diseases. The hunt to find rare variants that induce susceptibility to common diseases with high risk (or protection) will be more challenging, but eminently feasible with sequencing technology and ultra high-throughput genotyping. At some point in the future, the major genomic underpinnings for most cardiovascular diseases will be known. Furthermore, the integration of all of the genomic variants for any cardiovascular disease has not been undertaken. What follows is a brief overview of what is known today about the genetic basis for a sampling of disease entities within cardiovascular medicine.
METHODOLOGY.
The process of discovering relevant genetic underpinnings of generally complex traits requires an extensive analysis of genetic information in large populations. Complex traits without simple Mendelian patterns of inheritance are difficult to analyze, given that there are often multiple genes involved, with many gene interactions being important. However, even before attempting this task, perhaps the single most significant goal is to accurately and concisely define the phenotype in question. In addition, many variations
often exist within a single category of cardiovascular diseases such as hypertrophic cardiomyopathy (HCM) and acute MI. The ability to clearly define cases and controls is paramount to obtaining accurate and reproducible information.
often exist within a single category of cardiovascular diseases such as hypertrophic cardiomyopathy (HCM) and acute MI. The ability to clearly define cases and controls is paramount to obtaining accurate and reproducible information.
There are three major methods of identifying DNA variants associated with cardiovascular disease. Of note, some of the single nucleotide polymorphism (SNP) variants that have been found are not in a gene, but actually in areas of the genome not associated with gene expression. It is not yet known how these variants exert their effect. Of note, the major variants for MI (at 9p21) and AF (at 4q25) fall into this category of intergene (i.e., not in a gene).
I. GENOME-WIDE ASSOCIATION STUDIES.
Gene association studies utilize the concept that multiple SNPs, where a single nucleic acid substitution results in a different allele, can affect the risk of developing a disease in question. This is especially true in diseases of complex traits such as coronary artery disease (CAD) and MI. Using genes of interest in a particular disease phenotype, scans are conducted in areas of interest in both cases and controls to compare haplotype frequency to determine if a statistically significant difference between the two groups in the region of interest exists. One of the limitations of this technique is the inability to know whether the SNP of interest is involved in the pathogenesis or simply associated (in linkage disequilibrium) with another SNP that may actually be involved in the process. Utilizing this process of gene association across the entire genome is now possible with high-throughput sequencing technology of up to 1 million SNPs assessed per individual and the term “genome-wide” association has been coined. The SNPs assessed are tagging tiny bins of the genome, such that in a genome of an individual of European ancestry, there are only about 250,000 bins, most of which are sampled by current high-throughput genotyping. Each bin is typically inherited as a block (haplotype). The breakdown of the genome into bins via the International Haplotype map was critical in making current genome-wide association studies possible.
II. LINKAGE ANALYSIS.
Linkage analysis is another tool used to identify genes that are possibly involved in the pathogenesis of complex traits. The use of linkage analysis begins without any assumptions as to the potential involvement of various genes. It is based on the idea that during the process of meiosis when recombination events occur, they tend to involve loci on a particular chromosome that are closer together than farther apart. By following the inheritance of certain known loci, assumptions can be made about the presence of alleles that cosegregate with them. Using linkage analysis, the potential exists to identify these known loci as markers and determine the transmission through a pedigree and its relationship to the phenotype in question. In doing so, it may be possible to suggest that an allele in proximity to known loci may be associated with a particular phenotype. The logarithm of odds ratio (LOD score) is used to estimate the (distance) relationship between the known locus of interest and an unknown locus thought to be associated with the disease phenotype. A LOD score of 3 is used to classify such a statistically meaningful linkage.
III. GENE EXPRESSION PROFILING.
The identification of certain disease alleles or loci associated with disease-causing genes provides valuable information but remains limited in its scope. The statistical association of genes and disease does not prove causation or even involvement in disease. Gene expression profiling takes this concept one step forward in trying to delineate gene expression. The presence of transcription profiles may provide more useful information in terms of relevance of findings made in gene association studies or linkage analysis. Technology now permits the evaluation of large genomes in a rapid fashion to derive expression profiles, which can then be compared between diseased and healthy individuals to draw conclusions about which genes are transcriptionally active in certain phenotypes.
A. Coronary atherosclerosis and atherothrombosis.
Coronary atherothrombosis and atherosclerosis remain significant causes of morbidity and mortality in the population as a whole. There is a great deal of variation in presentation in atherosclerosis and in acute MI. The presence of atherosclerosis is necessary but not sufficient
for atherothrombosis. There are separate factors involved that predispose to plaque rupture and thrombosis. Even within the category of plaque rupture, the clinical phenotypes vary significantly, as reflected by the spectrum of diseases that constitute the acute coronary syndromes. These entities are complex traits and are influenced by multiple pathways. Inflammation, endothelial dysfunction, and dyslipidemias are only a few of the pathways influencing the development of atherothrombosis and atherosclerosis. Delineating the genetic basis, in most cases, is a work in progress, but it may help to broaden our understanding of the disease.
for atherothrombosis. There are separate factors involved that predispose to plaque rupture and thrombosis. Even within the category of plaque rupture, the clinical phenotypes vary significantly, as reflected by the spectrum of diseases that constitute the acute coronary syndromes. These entities are complex traits and are influenced by multiple pathways. Inflammation, endothelial dysfunction, and dyslipidemias are only a few of the pathways influencing the development of atherothrombosis and atherosclerosis. Delineating the genetic basis, in most cases, is a work in progress, but it may help to broaden our understanding of the disease.
1. Dyslipidemia: familial hyperlipidemia and other genetic variants.
Dyslipidemia is a known risk factor for the development of atherosclerosis. Familial hyperlipidemia (FH) is a well-characterized, albeit relatively uncommon, entity defined by elevated levels of serum LDL levels, which predispose to coronary atherosclerosis. Often, these patients can develop atherosclerotic disease between 20 and 30 years of age. In FH, the dyslipidemia is often severe and not responsive to standard lifestyle and pharmacologic interventions.
a. Autosomal dominant.
The underlying problem in this form of FH has to do with an abnormality in the clearance of the LDLR gene, and, as a consequence, elevated levels of LDL persist. The LDLR gene located at 19p13.2 has approximately 1,600 known mutations, and these appear to affect many aspects of LDLR function. Alterations in the gene range from point mutations to gene rearrangements. homozygotes and heterozygotes for FH vary in terms of the severity of lipid levels. The majority of individuals are heterozygous, and those with homozygous patterns of inheritance are more severely affected. Typically, these patients and affected family members develop premature coronary atherosclerosis and MI.
b. Autosomal recessive.
This occurs as a result of a mutation in the low-densitylipoprotein receptor adaptor protein 1 (LDLRAP1) gene at 1p36-p35. The phenotype of this form of FH is milder than the autosomal dominant form of the disease and more amenable to treatment with lipid-lowering agents.
c. Familial defective apolipoprotein B.
The cellular mechanisms involved in cholesterol metabolism are complex, and there are many potential targets where mutations can significantly affect phenotype. One such example is a point mutation in the apolipoprotein B (apo B) component of the LDL molecule. The Arg3500Gln is the most common substitution in the apo B (APOB) gene and results in the inability of LDL to bind to its LDLR. This mutant allele is more common in Northern European populations as a cause of hyperlipidemia, but the phenotype appears to be less severe than in the familial hyperlipidemia caused by LDLR mutations.
d. Proprotein convertase subtilisin/kexin type 9 (PCSK9).
Less than 5% of FH involves PCSK9 gene, located at 1p32.3, responsible for increasing deg radation of the LDL receptor. Mutations in PCSK9, such as D374Y, F216L, S127R, D129N, D374H, N425S, and R496W, confer gain in function and cause FH. Much rarer PCSK9 mutations are those that result in loss of func tion and act to reduce serum cholesterol levels, thereby conferring a protec tion against coronary atherosclerosis. Many new PCSK9 mutations have been identified, but their effect on PCSK9 functions requires further investigation.
In addition to the FH syndromes, there is a multitude of genes involved in more frequently occurring types of dyslipidemia. Phenotypically, these forms of dyslipidemia are less severe and more amenable to treatment than those observed in the FH population.
2. Endothelial function.
MEF2a is a transcription factor that localizes to the endo thelial cell of coronary arteries and is believed to be important in the function of endothelial cells. In 2003, a mutation in the MEF2a gene was described in a large family with autosomal dominant transmission of coronary atherothrombosis.
A 21-bp deletion in exon 11 was thought to result in loss of function. Three additional variants in the MEF2A thought to be associated with MI and CAD have also been reported. The findings from a large Spanish case-controlled study provided supporting evidence for only one of these variants, P279L. Further studies have failed to replicate these mutations in association with MI. The 21-bp deletion has not been found in any family apart from that in the original study. This particular mutation may be a “private mutation” for the family in the original study and therefore extremely difficult to replicate. In 2008, Lieb et al. (1) conducted a large German study, with 23 MI families, > 1,700 MI patients, and 2 large control populations, and did not find any evidence that the MEF2A gene had any linkage or association with MI/CAD.
A 21-bp deletion in exon 11 was thought to result in loss of function. Three additional variants in the MEF2A thought to be associated with MI and CAD have also been reported. The findings from a large Spanish case-controlled study provided supporting evidence for only one of these variants, P279L. Further studies have failed to replicate these mutations in association with MI. The 21-bp deletion has not been found in any family apart from that in the original study. This particular mutation may be a “private mutation” for the family in the original study and therefore extremely difficult to replicate. In 2008, Lieb et al. (1) conducted a large German study, with 23 MI families, > 1,700 MI patients, and 2 large control populations, and did not find any evidence that the MEF2A gene had any linkage or association with MI/CAD.
3. Inflammation.
The genes ALOX5AP (arachidonate 5-lipoxygenase—activating protein) and LTA4H (leukotriene A4 hydrolase) encode proteins involved in the leukotriene pathway, particularly in the synthesis of the proinflammatory leukotriene B mediators. The case for inflammation as a significant participant in acute MI has been made stronger by the discovery, through linkage analysis to a locus at 13q12-13, of a four SNP haplotype (Hap A) in the gene ALOX5AP. Carriers of the variant had higher levels of leukotriene B4. This variant was found to double the risk of MI and to almost double the risk of stroke. Another haplotype variant (Hap B) of the ALOX5AP gene was found to confer a doubling of the risk of MI in a British cohort. In Italy, researchers identified an increased risk for CAD with Hap B only. In a large German study, presence of the haplotype B indicated an association with an increased risk of MI. These studies provide strong evidence of the role of ALOX5AP gene for increased CAD risk in Europeans. In a case-control study of a US Midwestern population with European American ancestry, seven SNPs in the ALOX5AP gene along with two haplotypes (Hap A and Hap B) were genotyped. The results indicated that Hap B and one of the SNPs (SG13S377) were significantly associated with increased risk of premature CAD. In a meta-analysis conducted in 2010, the Hap B and SNP (rs1722842) variants in the ALOX5AP gene were associated with coronary heart disease, while Hap A was associated with the risk of MI.
The LTA4H gene encodes leukotriene A4 hydrolase and is also involved in the inflammation pathway. A haplotype (HapK) in the LTA4H gene moderately increased the risk of MI in a cohort from Iceland. A moderate risk was also found in studies of three US cohorts with European Americans, but HapK, although rarer in African Americans, tripled their risk of MI. The results of these studies with the ALOX5AP and the LTA4H variants provide the basis for further research to develop new drugs targeting the leukotriene pathway, thereby preventing or reducing the risk of MI and CAD in the future.
B. Connective tissue abnormalities and disease of the aorta.
Genetic mutations affecting the connective tissue and extracellular matrix typically affect multiple organ systems, but often the most devastating and lethal effects arise from those upon the cardiovascular system. Aortic dissection and rupture are often the consequences of such abnormalities, and what follows is a brief description of three such disorders.
1. Marfan syndrome.
This disorder is inherited in an autosomal dominant fashion with variable penetrance, and it affects the connective tissue, leading to abnormalities of organs of the cardiovascular, skeletal, and ocular systems. The genetic defect is in the fibrillin-1 (FBN1) gene on chromosome 15q21. Often, the diagnosis is made on clinical grounds alone. The classic features of tall stature, arachnodactyly, dolichostenomelia, pectus excavatum, ectopia lentis, and a positive family history all support a diagnosis of Marfan syndrome. The cardiovascular system is affected, and the most common cause of death in these patients is from aortic dissection and aortic rupture. Other common related problems include aortic dilation, aortic valve regurgitation, mitral valve prolapse (MVP), tricuspid valve prolapse, and arrhythmias. When patients with Marfan syndrome present with dissection, they are typically younger and do not have hypertension.
The FBN1 gene is responsible for producing a key constituent of microfibrils, which are important in the extracellular matrix. Microfibrils add to the elastic properties of extracellular tissue. Over 1,000 mutations in the FBN1 gene have been recognized and appear to affect different aspects of cellular processing of fibrillin-1. These mutations can vary from a SNP to a premature stop codon. There are no specific relationships between mutations and phenotypes as of yet. Family members with Marfan syndrome and the same FBNI gene mutation may show wide variation in onset and severity of cardiac symptoms. The diagnosis of Marfan syndrome is generally made on a clinical basis. However, genetic testing for Marfan syndrome is available.
2. Ehlers-Danlos syndrome.
Ehlers-Danlos syndrome is a group of connective tissue disorders caused by defects in proteins that are involved in the formation of collagen. It is uncommon and has an autosomal dominant pattern of inheritance. There are multiple types of Ehlers-Danlos syndrome based on the collagen type affected; however, the cardiovascular system is involved prominently in type IV, the vascular type of Ehlers-Danlos syndrome. The gene, COL3A1, involved in this subtype is localized to chromosome 2q24.3-q31 and encodes for type III procollagen. Vascular complications include dissections of the carotids and the vertebral arteries. Aortic dissections are the primary cause of death and often involve both the thoracic and the abdominal aortas. Diagnosis is usually ascertained clinically along with genetic testing for evidence of the mutation in the COL3A1 gene.
3. Loeys-Dietz syndrome.
Loeys-Dietz syndrome is a connective tissue disorder characterized by hypertelorism, cleft palate, and vascular disease in the form of arterial aneurysms and dissection. It is inherited in an autosomal dominant pattern. Clinically, these patients are at high risk for aortic dissection. Loeys-Dietz syndrome is caused by mutations in the transforming growth factor beta (TGF-β) receptors I and II genes (TGFBR1 and TGFBR2) on chromosome 3. Phenotypically, the characteristics are like those in Marfan syndrome, and also these patients appear similar (with the exception of the craniofacial abnormalities) to Ehlers-Danlos patients with vascular involvement (type IV). The relevance of this distinction is that those with Loeys-Dietz appear to have much lower intraoperative mortality during corrective vascular surgery. Genetic testing to identify the mutation is needed for definitive diagnosis, but it is not available in all laboratories.
C. Cardiomyopathies.
Primary disease of the myocardium affects both systolic and diastolic function and often results in heart failure or other adverse events over time. Many of the nonischemic cardiomyopathies have a strong genetic component to explain their phenotype. Perhaps, the most clinically relevant entities include dilated cardiomyopathy (DCM) and HCM. Cardiomyopathies can also occur as a secondary process in response to a separate unrelated factor (i.e., hypertension), and it is unknown to what degree genetic susceptibility determines the myocardial response/remodeling over time. Until recently, the classification of cardiomyopathies has been based on the phenotype and morphologic characteristics. However, with an improved knowledge of the genetics of these disorders, a new understanding and appreciation for the underlying mechanisms of disease in these disorders will undoubtedly influence how these entities are treated in the future.
1. Dilated cardiomyopathy.
DCM is characterized by dilation of one or both of the ventricular chambers resulting in severe systolic dysfunction and characterized by congestive heart failure. A genetic cause is thought to account for 20% to 50% of DCM cases, and in many cases, the pattern of inheritance is autosomal dominant. However, recessive, X-linked, and mitochondrial patterns of inheritance are also seen. Many cases of DCM are secondary to other etiologies. Mutations in more than 30 genes have been associated with this phenotype, and although the products of most of these genes are important structural proteins, there are others involved in the handling of calcium and regulation of energy within the myocytes. Again, however, there are often subtle differences in the
various types of DCM, such as age of onset and degree of clinical symptoms, all of which may suggest separate genetic abnormalities are at play. Mutations in the sarcomere protein genes account for approximately 10% of DCM. Mutations in LMNA gene, which encodes lamin A and lamin C, are seen in DCM with prominent conduction system disease. Although mutations in SCN5A are also associated with this type of DCM, the clinical picture differs as ventricular dysfunction is usually present. Dystrophin gene mutations are seen in X-linked DCM. Mutations in the ALMS1 gene are transmitted in a recessive pattern, resulting in DCM with hearing loss. Genetic testing is available for many of the gene mutations that have been identified. Table 42.1 lists some of the genetic variants that are believed to be associated with various DCM phenotypes.
various types of DCM, such as age of onset and degree of clinical symptoms, all of which may suggest separate genetic abnormalities are at play. Mutations in the sarcomere protein genes account for approximately 10% of DCM. Mutations in LMNA gene, which encodes lamin A and lamin C, are seen in DCM with prominent conduction system disease. Although mutations in SCN5A are also associated with this type of DCM, the clinical picture differs as ventricular dysfunction is usually present. Dystrophin gene mutations are seen in X-linked DCM. Mutations in the ALMS1 gene are transmitted in a recessive pattern, resulting in DCM with hearing loss. Genetic testing is available for many of the gene mutations that have been identified. Table 42.1 lists some of the genetic variants that are believed to be associated with various DCM phenotypes.
2. Hypertrophic cardiomyopathy.
HCM (see Chapter 10) is a highly variable and heterogeneous disease process that affects the myocardium, with clinical manifestations varying from completely asymptomatic to severe (sudden cardiac death). The
broad range of phenotypes and significant selection bias resulted in an overestimation of the mortality rate associated with this disease. The clinical spectrum of the disease is wide, and the ability to accurately predict outcomes remains challenging. The clinical variability of HCM is not only limited to the presenting phenotype but also related to age of presentation, clinical course, and eventual outcomes, making it very challenging to properly identify a phenotype of interest. The characteristic phenotype is an asymmetrically hypertrophied myocardium with a small ventricular chamber and occasionally a left ventricular (LV) outflow tract (with systolic anterior motion of the mitral valve) and/or an intracavitary gradient.
broad range of phenotypes and significant selection bias resulted in an overestimation of the mortality rate associated with this disease. The clinical spectrum of the disease is wide, and the ability to accurately predict outcomes remains challenging. The clinical variability of HCM is not only limited to the presenting phenotype but also related to age of presentation, clinical course, and eventual outcomes, making it very challenging to properly identify a phenotype of interest. The characteristic phenotype is an asymmetrically hypertrophied myocardium with a small ventricular chamber and occasionally a left ventricular (LV) outflow tract (with systolic anterior motion of the mitral valve) and/or an intracavitary gradient.
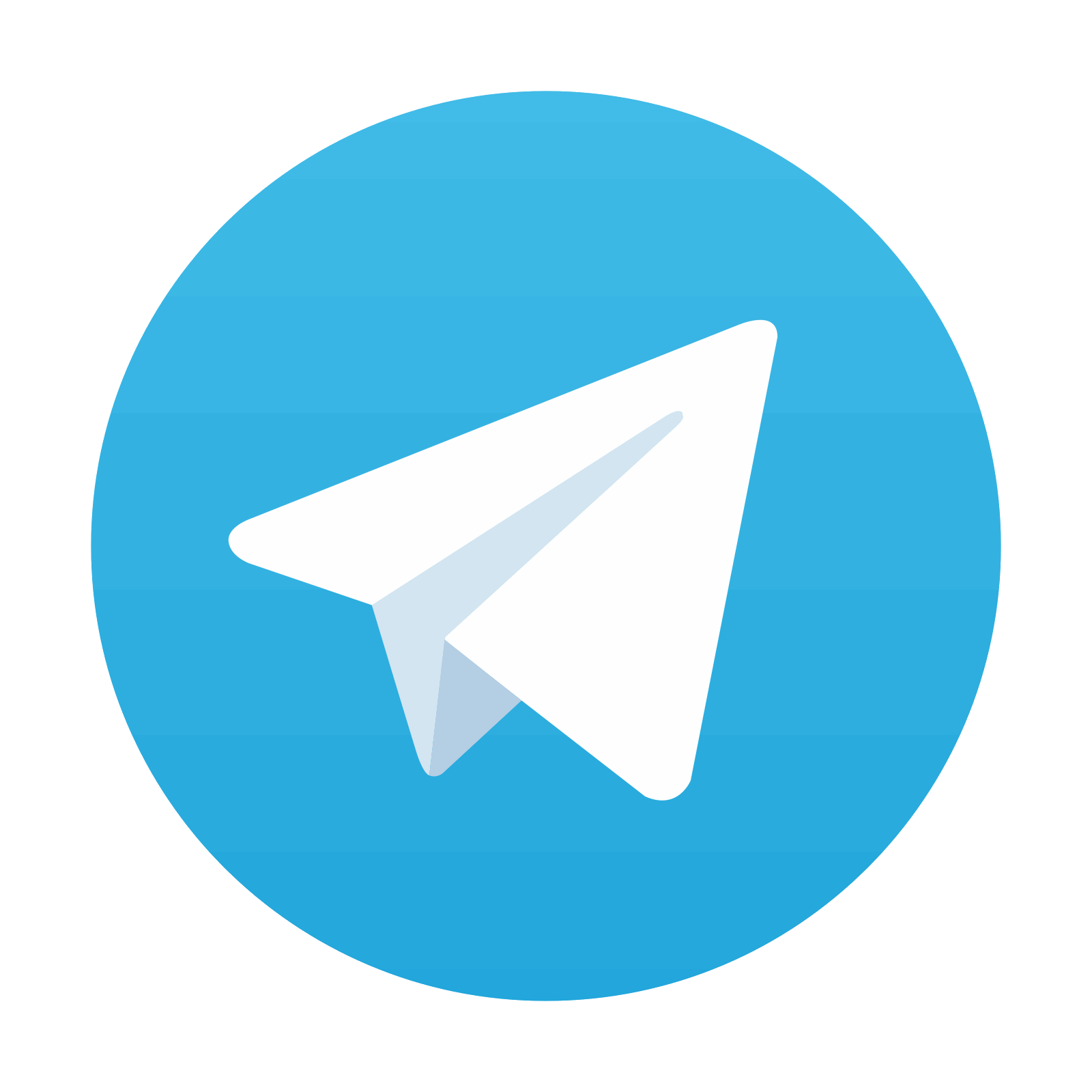
Stay updated, free articles. Join our Telegram channel

Full access? Get Clinical Tree
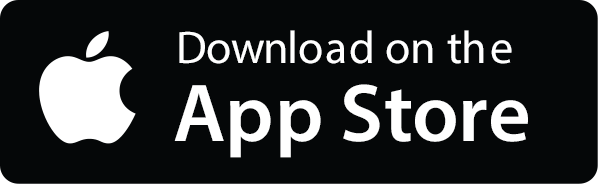
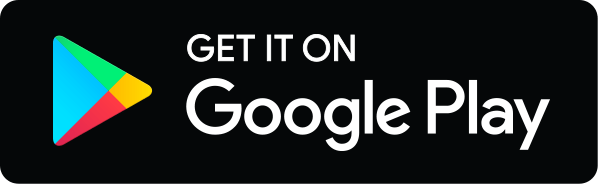
