Background
Color Doppler jet area (CDJA) is an important measure used to classify mitral regurgitation (MR) severity. The investigators hypothesized that the presence and configuration of multiple regurgitant jets can alter CDJA quantification for fixed regurgitant volumes. This has relevance to MR assessment prior to the treatment of valves with multiple regurgitant orifices or after surgical or percutaneous double-orifice mitral valve repair.
Methods
An in vitro model was developed to create jets flowing through a simulated mitral orifice into an imaging chamber. The flow loop was driven with a pulsatile pump at 60 beats/min containing a water-glycerol solution approximating the viscosity of blood. At the orifice, simulated regurgitant stroke volumes of 2.5 to 25 mL were created through plates having either single openings with orifice areas from 0.125 to 0.50 cm 2 or two to four openings with total orifice area of 0.25 cm 2 and varied linear spacing. An 8-MHz transthoracic two-dimensional ultrasound probe was used to acquire jet velocities by continuous-wave Doppler as well as color Doppler for offline analysis. CDJA values were obtained with custom automated pixel-counting software.
Results
Peak jet velocities ranged from 30 to 550 cm/sec. For single jets, normalized average CDJA values increased nonlinearly as a function of average Reynolds number. Peak CDJA values were up to 62% higher for multiple jets compared with single jets with similar total orifice areas and simulated regurgitant stroke volumes. The presence or absence of multiple jets, rather than the total number of jets, appeared to have a greater effect on maximum CDJA. In addition, peak CDJA values for multiple jets increased with increased linear spacing.
Conclusions
A fixed regurgitant volume involving multiple jets will have a larger CDJA value than the same total volume from a single jet. The source of this discrepancy appears to be increased ambient fluid entrainment from adjacent regurgitant jets. This potential overestimation of MR severity using color Doppler flow jets should be taken into consideration when assessing MR prior to treatment or when assessing residual MR after double-orifice mitral valve repair.
Because each of the surrogate measures of mitral regurgitation (MR) severity has limitations, the American Society of Echocardiography suggests using an integrative approach for classifying MR. One of the recommended metrics (the easiest to acquire and most commonly used) is the size of the color Doppler jet area (CDJA) in the left atrium created by the regurgitant jet. The challenge in assessing MR severity using CDJA is estimating volumetric flow from the spatial distribution of velocity-encoded pixels. This becomes much more difficult when flow is turbulent and fast enough to cause multiple aliasing artifacts, as is the case with MR. CDJA estimates of MR severity are often confounded by several factors independent of the regurgitant volume. These factors include instrument settings, the shape and size of the regurgitant orifice, blood viscosity, jet momentum, jet velocity, jet direction, and driving pressure.
To the best of our knowledge, studies demonstrating the limitations of CDJA measurements have reported only on flow through a single regurgitant orifice. However, given the complex three-dimensional geometry of the mitral valve coaptation surface, it is common for diseased mitral valves to have multiple regurgitant lesions (e.g., in Barlow’s disease or endocarditis). In addition, mitral valve repair or replacement techniques that alter mitral valve geometry may also alter the spatial distribution of residual regurgitation at the mitral valve orifice. In particular, double-orifice repair, in which the scallops of the anterior and posterior leaflets are approximated, converts a single-orifice valve into a double-orifice valve. The creation of a double-orifice valve may result in multiple, smaller residual regurgitant jets. A number of computational and experimental studies have examined fluid dynamics after double-orifice repair. These efforts have focused on forward flow through the mitral valve during diastole. In the current study, we were interested in understanding how CDJA measurements are influenced by the number and spacing of multiple regurgitant jets. These factors may be important in determining residual MR severity after double-orifice mitral repair.
It has previously been reported that the CDJA for a single constrained jet grows nonlinearly with increasing jet momentum. We hypothesized that the number and configuration of multiple regurgitant jets can also cause increased CDJA measurements relative to single jets of similar total regurgitant volume. This seems likely given that the amount of ambient fluid entrainment into the jets increases when multiple jets are present. An inherent limitation of color Doppler recordings is the inability to differentiate between moving blood in the regurgitant jet and the entrained left atrial blood. As a result of this limitation, a fixed regurgitant volume should have a maximum CDJA when the mixing between the regurgitant flow and the atrial blood is maximized (and when the imaging plane intersects all of the jets). To test these hypotheses, we created an experimental setup in which various configurations of jets with regard to number and spacing could be analyzed. Direct comparisons were facilitated by keeping the total mass flux (i.e., the rate of mass flow across an area), total momentum flux (i.e., the rate of momentum flow across an area), peak velocities, and driving pressure constant (see Appendix ).
Methods
Data Acquisition
A schematic of the flow loop is shown in Figure 1 A. Unidirectional, pulsatile flow with an adjustable simulated regurgitant stroke volume (SRSV) and systolic ratio was ejected from the pump (Harvard Apparatus Pulsatile Blood Pump Model 1423; Harvard Apparatus, Holliston, MA) and traveled through flexible plastic tubing and a one-way valve to a clear acrylic imaging chamber. The blood-analogue fluid (with viscosity of approximately 3 cP, composed of 70% water, 30% glycerol, and 80 μm ultrasound scattering particles) flowed through the interchangeable orifice into the imaging chamber, where echocardiographic data were recorded. The dimensions of the imaging chamber were approximately 20 × 20 cm, with a height of 17 cm. A large foam plate with an imaging window cutout was placed at the top of the chamber to prevent imaging artifacts from movement of the air-water interface. A schematic of the interchangeable circular orifices is shown in Figure 1 B. We varied SRSV from 2.5 to 25 mL and measured instantaneous and time-averaged bulk flow rates within the tubing using an ultrasonic flow probe positioned between the pulsatile pump and regurgitant orifice ( Figure 1 C). The systolic ratio was approximately 50% for each case. Average and peak Reynolds numbers ( Re ; representing the ratio between inertial and viscous forces) were calculated for the single-orifice cases using the following formulas, respectively:
R e avg = ρ U avg sys D / μ
R e peak = ρ U peak sys D / μ ,

Ultrasound Acquisitions
Two-dimensional images were collected from the top of the imaging chamber using a GE Vingmed System V ultrasound machine (GE Vingmed Ultrasound AS, Horten, Norway) with an 8-MHz transthoracic ultrasound probe. Color Doppler data were acquired at 15.3 frames/sec with a depth of 14 cm and Nyquist limits between −27 and 63 cm/sec. Continuous-wave Doppler recordings were used to acquire the peak transorifice velocity ( Figure 1 D). Continuous-wave Doppler recordings were also used to confirm that the ultrasound probe was aligned parallel to the main axis of the jet such that waveforms and peak velocities matched through the most distant orifices in each plate.
Color Doppler Data Processing
Automated colored pixel–counting code was written using MATLAB (The MathWorks, Inc., Natick, MA) to consistently quantify and compare CDJA ( Figure 1 E). For each cine loop, all frames over three successive cycles were imported into the program. For each frame, every pixel within the region of interest was converted to black or white by comparing its 8-bit red, green, and blue values to a threshold gray level in the red-green-blue color space. This allowed pixels comprising each jet to be converted to white (and counted as colored pixels), while all other pixels were converted to black. The program allows the user to count the total number of colored pixels in every recorded frame ( Figure 1 F, Video 1 ) and plot the jet area as a function of time ( Figure 1 G), giving maximum and average CDJAs for each cycle. Because the units of our quantitative analysis (ie, the number of colored pixels) are not the conventional metric clinicians use to evaluate MR severity, we provide examples illustrating the differences in appearance of three regurgitant jets with various jet areas in Figure 2 .

Results
Peak centerline jet velocities of flow through the orifices as measured by continuous-wave Doppler are shown in Table 1 . These values ranged between 30 and 550 cm/sec.
Peak velocity (m/sec) | |||||||
---|---|---|---|---|---|---|---|
Average flow rate (mL/sec) | One orifice | Two orifice (near) | Two orifice (far) | Three orifice (near) | Three orifice (far) | Four orifice (near) | Four orifice (far) |
2.5 | 0.3 | 0.3 | 0.3 | 0.3 | 0.4 | 0.4 | 0.4 |
5 | 0.9 | 0.9 | 0.9 | 0.9 | 0.9 | 0.9 | 0.9 |
7.5 | 1.4 | 1.4 | 1.4 | 1.4 | 1.4 | 1.4 | 1.4 |
10 | 2 | 2 | 2.1 | 2 | 2.1 | 2.1 | 2 |
12.5 | 3.3 | 3.5 | 3.5 | 3.3 | 3.5 | 3.5 | 3.3 |
15 | 4 | 4 | 4 | 3.8 | 4 | 3.8 | 3.8 |
20 | 4.5 | 4.5 | 4.7 | 4.5 | 4.5 | 4.5 | 4.5 |
25 | 5 | 5 | 5.5 | 5.3 | 5.3 | 5.3 | 5.3 |
Results for the various single-orifice sizes were compared as functions of Re . Figure 3 A shows normalized average CDJA measurements as functions of average Re . Jet areas were normalized by the largest average jet area measured to allow comparison of dimensionless variables. It appears that the data collapse onto a single curve that is a function of the corresponding Re .

Peak measured jet areas for multiple-orifice configurations with far-spacing (30 mm) are compared with the single-orifice case in Figure 3 B. The total orifice area was 0.25 cm 2 for each of these cases. It can be seen that for any given SRSV above a threshold volume of about 5 mL, peak measured jet areas were significantly higher if multiple orifices were present. Additional regurgitant jets beyond two did not seem to significantly affect the measurements. At the threshold SRSV, the curve for the single-orifice case appears to separate from those for the multiple-orifice cases. The curves eventually return to having similar slopes, though at different absolute values of maximum jet area. At SRSVs ≥ 12.5 mL, jet areas for two orifices with far-spacing were 44% to 62% greater relative to single orifices with the same SRSVs.
Peak measured jet areas for multiple-orifice configurations with near spacing (15 mm) are compared with the single-orifice case in Figure 3 C. The total orifice area was 0.25 cm 2 for each of these cases. Again, it can be seen that for any given SRSV above about 5 mL, peak measured jet areas were typically higher if multiple orifices were present. Similar to the cases shown in Figure 3 B, the curve for the single-orifice case separates from those for the multiple-orifice cases, and the curves eventually return to having similar slopes at different absolute values of jet area (although the differences are not as large as for the far-spacing cases). At SRSVs ≥ 12.5 mL, jet areas for two orifices with near spacing were 18% to 33% greater relative to single orifices with the same SRSVs.
The effect of spacing distance was also directly compared. Figure 3 D shows a comparison between peak measured jet areas for far and near spacing with two jets. A similar relationship between maximum jet area and spacing was seen for conditions with three and four jets (not shown). In each case, a given number of jets would produce similar measurements irrespective of spacing distance until a threshold SRSV between 5 and 10 mL was reached. After that point, the curves separate, and the far-spacing cases reach higher maximum jet area values. In Figure 3 D, at SRSVs ≥ 12.5 mL, jet areas for two far-spaced orifices were 19% to 32% greater relative to two near-spaced orifices with the same SRSVs.
Results
Peak centerline jet velocities of flow through the orifices as measured by continuous-wave Doppler are shown in Table 1 . These values ranged between 30 and 550 cm/sec.
Peak velocity (m/sec) | |||||||
---|---|---|---|---|---|---|---|
Average flow rate (mL/sec) | One orifice | Two orifice (near) | Two orifice (far) | Three orifice (near) | Three orifice (far) | Four orifice (near) | Four orifice (far) |
2.5 | 0.3 | 0.3 | 0.3 | 0.3 | 0.4 | 0.4 | 0.4 |
5 | 0.9 | 0.9 | 0.9 | 0.9 | 0.9 | 0.9 | 0.9 |
7.5 | 1.4 | 1.4 | 1.4 | 1.4 | 1.4 | 1.4 | 1.4 |
10 | 2 | 2 | 2.1 | 2 | 2.1 | 2.1 | 2 |
12.5 | 3.3 | 3.5 | 3.5 | 3.3 | 3.5 | 3.5 | 3.3 |
15 | 4 | 4 | 4 | 3.8 | 4 | 3.8 | 3.8 |
20 | 4.5 | 4.5 | 4.7 | 4.5 | 4.5 | 4.5 | 4.5 |
25 | 5 | 5 | 5.5 | 5.3 | 5.3 | 5.3 | 5.3 |
Results for the various single-orifice sizes were compared as functions of Re . Figure 3 A shows normalized average CDJA measurements as functions of average Re . Jet areas were normalized by the largest average jet area measured to allow comparison of dimensionless variables. It appears that the data collapse onto a single curve that is a function of the corresponding Re .

Peak measured jet areas for multiple-orifice configurations with far-spacing (30 mm) are compared with the single-orifice case in Figure 3 B. The total orifice area was 0.25 cm 2 for each of these cases. It can be seen that for any given SRSV above a threshold volume of about 5 mL, peak measured jet areas were significantly higher if multiple orifices were present. Additional regurgitant jets beyond two did not seem to significantly affect the measurements. At the threshold SRSV, the curve for the single-orifice case appears to separate from those for the multiple-orifice cases. The curves eventually return to having similar slopes, though at different absolute values of maximum jet area. At SRSVs ≥ 12.5 mL, jet areas for two orifices with far-spacing were 44% to 62% greater relative to single orifices with the same SRSVs.
Peak measured jet areas for multiple-orifice configurations with near spacing (15 mm) are compared with the single-orifice case in Figure 3 C. The total orifice area was 0.25 cm 2 for each of these cases. Again, it can be seen that for any given SRSV above about 5 mL, peak measured jet areas were typically higher if multiple orifices were present. Similar to the cases shown in Figure 3 B, the curve for the single-orifice case separates from those for the multiple-orifice cases, and the curves eventually return to having similar slopes at different absolute values of jet area (although the differences are not as large as for the far-spacing cases). At SRSVs ≥ 12.5 mL, jet areas for two orifices with near spacing were 18% to 33% greater relative to single orifices with the same SRSVs.
The effect of spacing distance was also directly compared. Figure 3 D shows a comparison between peak measured jet areas for far and near spacing with two jets. A similar relationship between maximum jet area and spacing was seen for conditions with three and four jets (not shown). In each case, a given number of jets would produce similar measurements irrespective of spacing distance until a threshold SRSV between 5 and 10 mL was reached. After that point, the curves separate, and the far-spacing cases reach higher maximum jet area values. In Figure 3 D, at SRSVs ≥ 12.5 mL, jet areas for two far-spaced orifices were 19% to 32% greater relative to two near-spaced orifices with the same SRSVs.
Discussion
Although color Doppler imaging has been used to measure MR severity for decades, efforts to better understand and improve the clinical utility of this diagnostic tool are ongoing. Much work has focused on quantitative measures such as effective regurgitant orifice area (by the proximal isovelocity surface area method) and vena contracta width. Recent studies have explored the use of three-dimensional color Doppler imaging. Two-dimensional CDJA measurements, however, are still widely relied on to estimate MR severity. A number of factors have been reported to influence the accuracy of these measurements. As mentioned previously, these include instrument settings, geometric orifice characteristics, blood viscosity, and jet properties such as momentum, velocity, pressure gradient, and eccentricity. Another limitation is the use of an instantaneous measurement of jet area to approximate a volume that arises from the integration of unsteady flow over the duration of systole. The left atrial chamber walls and pulmonary venous inflow also affect the accuracy of measurements. Refinements such as normalization by left atrial area have been suggested to improve the quantitative accuracy of the technique. Practical applications such as simply increasing MR severity by one grade for eccentric jets have been used. Regardless, there is agreement that CDJA measurements should be used only as a qualitative approximation of MR severity.
This study was performed to determine how the presence of multiple regurgitant jets might affect CDJA measurements. From our results, the presence of more than one regurgitant jet leads to an increase in peak measured jet area for a given regurgitant volume. This is not surprising considering the fluid dynamics of regurgitant jets. MR involves the persistent cyclic discharge of transient, or starting, jets from the left ventricle into the left atrium. When a starting jet is discharged into an ambient fluid, a leading vortex ring is formed, and a shear layer develops at the interface between the jet and the ambient fluid. This shear layer is inherently unstable and can easily develop into multiple vortical structures in the trailing portion of the jet. The growth of shear layer instabilities into vortical structures is dictated by the magnitude of the velocity difference between the jet and the ambient fluid. The leading and trailing vortex rings all entrain fluid from the surrounding ambient fluid, and therefore, the total jet volume increases with distance from the orifice. Accordingly, the visualization of CDJA does not just involve the regurgitant volume but also reflects the amount of entrained ambient (or atrial) fluid. Processes that tend to alter the degree of entrainment will then also affect CDJA measurements, and this fact explains the most significant results of this study.
Examination of Figure 3 A shows that for a single orifice, there appears to be a dependence of normalized average CDJA measurements on average Re . The data for all of the single-orifice sizes collapse together when analyzed as functions of Re . There is initially a region with a steep linear relationship between CDJA and Re . This is followed later by a region where a flatter linear relationship exists. The transition between these regions is gradual and appears to be centered on an average Re of approximately 1500. Thomas et al. demonstrated similar findings that CDJA grows nonlinearly with momentum flux and that the flatter sloped region of the curve is caused by chamber constraints upon the jet. Here we have shown the same behavior with respect to Re , which is not surprising given that momentum flux is proportional to the square of Re . This emphasizes the fact that the ratio of inertial and viscous forces (i.e., Re ) is an important determinant of jet behavior and resultant CDJA measurements. The average Re for a single jet is directly proportional to the orifice diameter and average velocity. It should be noted that most of the flows imaged in this study likely involved turbulent jets, so the dominant trends in different Re regimes were not likely the results of laminar to turbulent flow transitions. Instead, the varied behavior of turbulent jets at different Re values led to the results that were obtained.
For each case shown in Figure 3 A, it is likely that the steeper linear region reflects increased entrainment by vortical structures as Re increases. The flatter linear region results from finite chamber constraints that limit how much the jet can continue to grow once the threshold Re is reached. At higher values of Re , the jets spend a large percentage of time impinging on the top of the imaging chamber. This constrains additional growth to be mainly in the radial direction, and these increases are smaller for a given increase in Re than if the jets could also continue to grow in the axial direction. This phenomenon is likely to occur in vivo as well, because the left atrium is a confined chamber.
Whether multiple jets were spaced nearer or farther from each other, the CDJA values were typically higher than the corresponding single-orifice values at matched SRSV. For these comparisons, SRSV was plotted along the abscissa rather than Re because the geometries were variable between cases, and there was no consistently meaningful length scale with which to define and compare Re . In comparing the far-spaced multiple-jet cases versus single-jet case ( Figure 3 B), we observed that multiple jets significantly increased the maximum CDJA at SRSVs > 10 mL. It appears that the presence of more than one jet, not necessarily the number of multiple jets, has the most significant effect on CDJA. At higher SRSV, the curves all reach a point at which finite chamber effects cause them to level off and increase at a reduced slope, but the multiple-orifice cases do so at higher absolute values of maximum jet area. This can be explained by the fact that when the multiple jets coalesce, they coalesce into one jet with a much larger effective diameter and shear layer surface area ( Figures 4 A and 4 B). This will lead to increased entrainment, especially before the finite chamber exerts an influence. The curves start to separate at SRSVs of about 5 mL, which is the threshold at which adjacent jets start to have significant interactions with each other.
The near-spaced multiple-jet cases are compared with the single jet-case in Figure 3 C. As before, the single-jet cases typically have smaller CDJA measurements than the multiple-jet cases at each SRSV. There also appears to be a threshold SRSV at about 5 mL at which the curves begin to diverge. The separations between the single-orifice curves and near-spaced multiple-orifice curves are not as pronounced as for the far-spaced cases, because the effective coalesced diameter and surface area for multiple jets become smaller with tighter spacing.
The direct effect of spacing distance is shown in Figure 3 D. Any given number of jets produces similar measurements whether near spaced or far spaced until SRSV passes 5 to 10 mL. After that point, the far-spaced jets have consistently larger CDJA values. Again, this is reasonable, because the far-spaced jets eventually coalesce into single jets with larger effective diameters ( Figure 4 ).
The effect of multiple jets on CDJA has important clinical implications for evaluating MR severity. In our studies, peak CDJA values increased by up to 62% compared with single jets with the same SRSV. Although the volumes tested in our studies are consistent with mild to mild-to-moderate regurgitation, increases of the observed magnitudes could make the total CDJA more consistent with moderate to moderate-to-severe regurgitation.
It is important to note that we conducted our studies under conditions in which we attempted to isolate the contributions of the number and configuration of multiple regurgitant orifices. To do this, we kept the total momentum flux, peak velocities, pressure gradients, and instrument settings constant. These and other physiologic and technical factors will independently affect CDJA in the setting of multiple jets. Whether the magnitude of these effects changes with the number of jets likely depends on how strongly related each variable is to the entrainment process. For instance, adherence of regurgitant jets to the left atrial chamber (because of the Coanda effect) will reduce the ability of multiple jets to increase entrainment because of the bound surfaces. Separately, the impingement of a jet on the concave left atrial wall will also reduce CDJA, (because of momentum transfer and three-dimensional distortion) but this process may not be altered greatly by having multiple parallel jets. In contrast, whether the decrease in CDJA related to acute severe MR (with increased atrial pressure and decreased atrial compliance ) is altered with multiple jets is harder to predict. Nevertheless, for any given physiologic situation, we do expect some degree of increased entrainment and CDJA due to the presence of multiple jets.
Limitations
Limitations of this study included the idealization of certain physiologic factors to facilitate imaging and data analysis. For instance, the imaging chamber was much larger than a typical left atrium. This means that some jets were allowed to become much larger than they would under physiologic constraints. Transorifice pressure gradient values were not explicitly matched to physiologic values in our study. Because the pressure gradients were kept constant, however, the trends seen between direct comparisons remain valid. In addition, many of the measured peak jet velocities were lower than those usually seen clinically in patients with moderate-to-severe or severe MR. Including lower velocity jets, however, did allow for a more complete analysis of the relevant fluid dynamics. Although the specific jet properties we studied are representative of the mild to mild-to-moderate MR severity range, we believe that the fundamental findings can be directly applied to more severe cases of MR. Our colored pixel–counting software relies on exported Digital Imaging and Communications in Medicine color Doppler images and, like CDJA, is directly influenced by instrument settings that affect encoding (such as pulse repetition frequency, frame rate, color gain, and Nyquist limit). These settings were kept constant for all acquisitions. No jet border detection or interpolation was implemented, so some cineloops had small pockets of missing pixels within the jet core (mainly at low SRSVs). We do not expect any of these limitations to affect the primary conclusions of this study.
Conclusions
We have shown that peak CDJA values are higher for multiple jets compared with single jets with matched total orifice areas and regurgitant volumes. For a given total orifice area, the presence of multiple jets appears to be more important than the exact number of jets. With multiple jets, increased linear spacing will lead to larger peak CDJA values. In addition, we have confirmed the expectation for single jets that normalized CDJA values grow nonlinearly as a function of Re . These findings have relevance to assessing MR in situations in which multiple jets are present, as can occur with Barlow’s disease or endocarditis. Our findings are also important after procedures that reduce MR (such as double-orifice mitral valve repair), in which multiple small residual jets may be present and confound the assessment of procedural efficacy. CDJA measurements in such settings should be evaluated carefully to avoid an overestimation of residual MR severity. Future work examining the accuracy of alternative MR severity assessment techniques in the setting of multiple jets should also provide useful information.
Appendix
We illustrate here how conditions were created with constant total mass flux, momentum flux, peak velocity, and driving pressure using different orifice configurations. First, we consider a jet of mass flux m 0 , density ρ, and uniform cross-sectional velocity U 0 ejected through a circular orifice with area A 0 , where
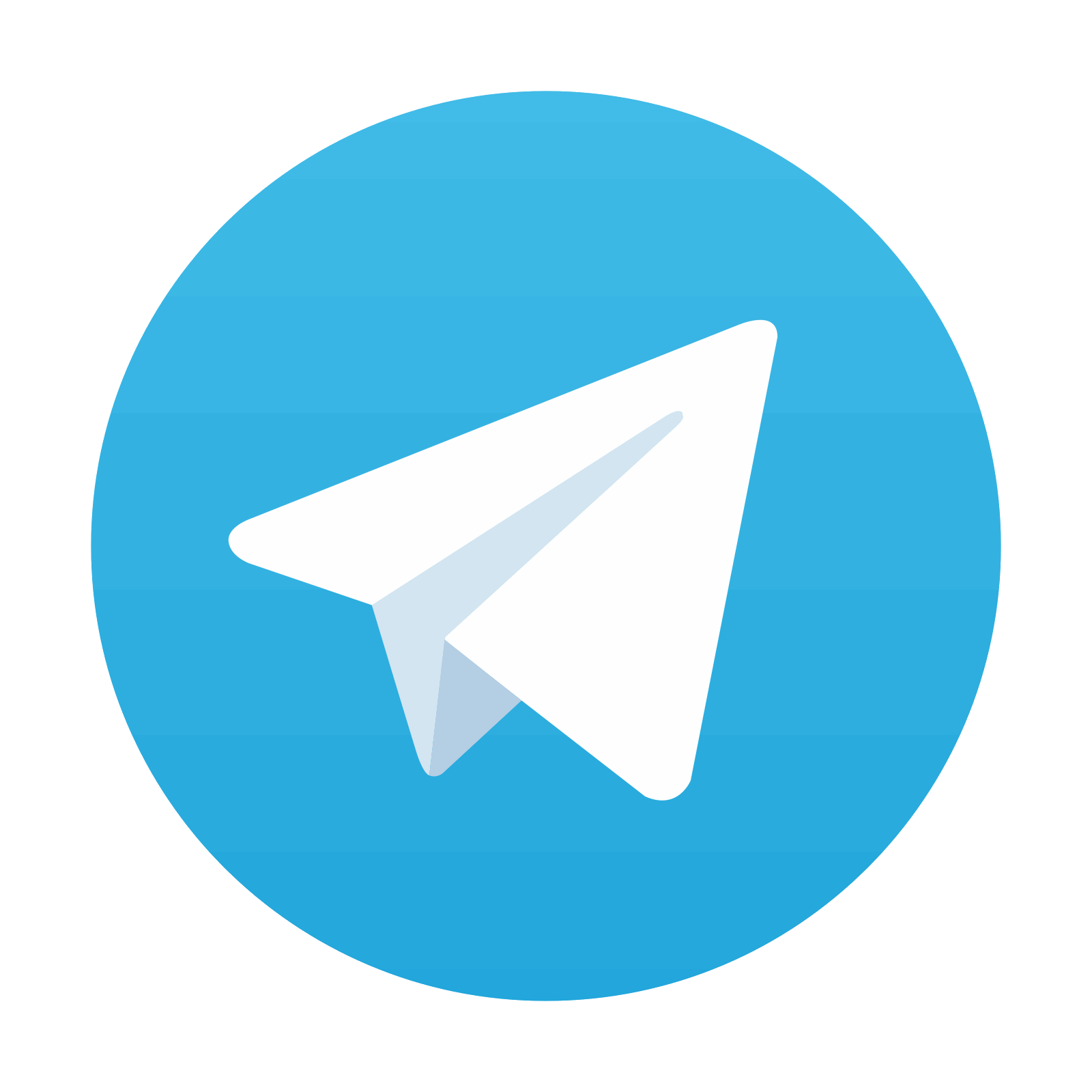
Stay updated, free articles. Join our Telegram channel

Full access? Get Clinical Tree
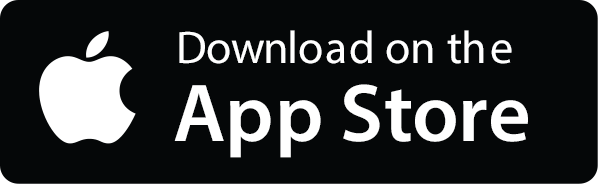
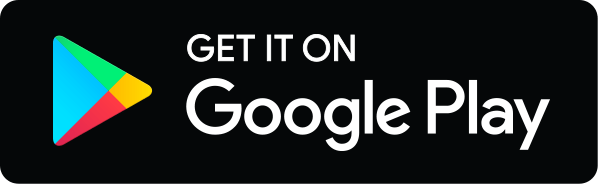