Hemostasis is the physiologic response to bleeding that occurs when a vessel wall is disrupted and is regulated through several processes. The first response is the activation, adhesion, and aggregation of platelets. The platelet plug that forms is stabilized by the deposition of fibrin, which is generated on the surface of the platelet membrane and on nearby disrupted vessel surfaces. The extent of thrombosis is limited by endogenous anticoagulant systems and by the fibrinolytic system, which precludes the development of thrombosis beyond the site of injury.1
Thrombosis is a pathologic process characterized by an inappropriate or exaggerated generation of clot. It may occur if there is vessel wall injury without disruption (i.e., no bleeding) or in the setting of excess activation of platelets or coagulation factors. Inadequate endogenous anticoagulant or fibrinolytic function may also contribute to the development of thrombosis.
In the arterial circulation, hemostasis and thrombosis are platelet dependent, where high shear stresses and potentially turbulent flow stimulate this mechanism and where the fibrinolytic system regulates secondary fibrin formation.2,3 By contrast, the venous system is characterized by low flow and stasis, permitting the development of hemostasis and thrombosis through activation of the coagulation cascade and regulation by endogenous anticoagulant proteins.1 Thrombosis may occur when the quantity of platelets is low or their function is inhibited. This distinction is important when considering defined hypercoagulable states and the role they may play in venous thrombosis.
Virchow’s triad has been the basis of our understanding of the mechanisms that lead to thrombus formation in the venous system. Virchow postulated that changes in the vessel wall, venous stasis, and hypercoagulability of the blood lead to thrombus formation.4 This underlying principle has been applied to many clinical situations.
A healthy endothelium is nonthrombogenic. However, changes in the endothelial surface brought on by inflammation, injury, or damage lead to initiation of thrombus formation by expression of tissue factor and subsequent activation and aggregation of platelets. Endothelial damage may be caused by direct trauma, exposure to endotoxins, inflammatory cytokines, thrombin, or low oxygen tension.5 Injured endothelial cells release tissue factor and plasminogen activator inhibitor-1 (PAI-1) and internalize thrombomodulin, which leads to thrombus formation. They also produce less tissue plasminogen activator (tPA), the principal activator of fibrinolysis, which further promotes thrombosis.6 The presence of functional platelets is not necessary for the initiation or propagation of thrombosis on these damaged surfaces.
Venous flow is supported by the calf muscles, and venous pooling is prevented by venous valves. Venous stasis can be produced by immobility (hospitalization, surgery, stroke), increased venous pressure (varicose veins, venous insufficiency, heart failure), and medical conditions that increase blood viscosity (paraproteinemia, cryoglobulinemia, myeloproliferative disorders).
Normally, there is a balance between prothrombotic aspects of the coagulation system, including activatable platelets and clotting factors, and antithrombotic systems, including endogenous anticoagulants and the fibrinolytic system. However, clotting factors that are usually finely regulated can escape regulation if the level or function of inhibitors is reduced or activators are increased. During normal hemostasis, clot formation intrinsically leads to activation of endogenous anticoagulants and fibrinolysis to limit clot extension. Alteration of this balance may lead to thrombosis.
The clotting cascade (Figure 4-1) as we understand it today can be activated by contact of factor XII with collagen or other surfaces (contact pathway) but is more often triggered by exposure to tissue factor, which is released by injured endothelium (tissue factor pathway). In venous thrombosis, factor X is usually activated by tissue factor bound to factor VIIa. Activated factor X (factor Xa) then stimulates the generation of thrombin. Thrombin feeds back into the cascade, activating factors V and VIII, which increase generation of factor Xa, creating an amplification of clot formation. To control this amplification, thrombin also stimulates the activation of protein C. Activated protein C (aPC), using protein S as a cofactor, breaks down activated factors V and VIII, limiting the amount of thrombus formation. Antithrombin binds directly to activated factors, especially factors Xa and thrombin, to counterbalance the thrombotic process.
In most venous hypercoagulable states, thrombosis occurs when there is an increase in prothrombotic factors or reduced activity of counterregulatory systems. However, direct activation of factor X by malignant cells has been described; this may be one of the factors of increased thrombosis in cancer patients.7
Clinical conditions leading to activation of the clotting system include trauma, surgery, systemic infections, malignancies, and decreased mobility. Individuals with underlying hypercoagulable states are more vulnerable in these clinical settings and may manifest with venous thrombosis.
Hypercoagulable states are defined as an imbalance between prothrombotic and antithrombotic processes. Traditionally, hypercoagulable states can be divided into acquired and inherited disease states. Common risk factors include trauma, surgery, immobility, hormonal changes (pregnancy, use of oral contraceptives, hormone replacement), inflammatory or autoimmune conditions (antiphospholipid antibody syndrome [APLS]), myeloproliferative disorders, and cancer. Identifiable and well-defined inherited hypercoagulable states include factor V Leiden (FVL), prothrombin gene mutation, and deficiencies of protein S and C and antithrombin.
Specific inherited and acquired hypercoagulable states can be identified by laboratory testing; common disorders are listed in Table 4-1. Inherited and long-standing acquired hypercoagulable states have the potential to augment the hypercoagulability induced by pathophysiologic processes and traditional risk factors in venous thromboembolism.
Incidence | ||||||
---|---|---|---|---|---|---|
Hypercoagulable State | Mechanism | Testing | Diagnostic Result | General Population | Patients with VTE | References |
Genetic Polymorphisms | ||||||
Factor V Leiden (aPC resistance) | Point mutation in factor V → resistance to anticoagulant activity of aPC | aPCR assay PCR for mutation | Low aPCR ratio Presence of mutation | 4.8% (whites) | 18.8% | 8, 9 |
Prothrombin G20210A mutation | Unknown; point mutation in Factor II (prothrombin) gene associated with modest increase in factor II levels | PCR for mutation | Presence of mutation | 2.7% (whites) | 7.1% | 9–11 |
Endogenous Anticoagulant Deficiency | ||||||
Antithrombin | Diminished capacity to bind activated coagulation factors | Antithrombin activity assay | Activity <50% | 0.02% | 1.9% | 10,12 |
Protein C | Diminished capacity to inactivate factors Va and VIIIa | Protein C activity assay | Classical deficiency Activity <30%–40% | 0.2%–0.4% | 3.7% | 10,13 |
Protein S | Diminished capacity to inactivate factors Va and VIIIa | Free protein S antigen | Antigen <30%–40% | 0.003% | 2.3% | 10,14 |
Hyperhomocysteinemia | Injury to endothelial surface; may lead to accelerated atherosclerosis Role in venous thrombosis controversial; mechanism unclear | Fasting serum homocysteine | Elevated | 5%–7% | 10% | 15,16 |
Fibrinolytic Defects | ||||||
Dysfibrinogenemia | Inherited mutation of fibrinogen resulting in resistance to fibrinolytic factors | Nonspecific testing includes fibrinogen level and euglobulin lysis time | Fibrinogen level may be normal or high ELT prolonged | <0.01% | 0.8% | 17,18 |
Hypofibrinolysis | Endothelial dysfunction or rare genetic defects resulting in underproduction or release of tPA or excess PAI-1 | tPA antigen and activity assays PAI-1 antigen and activity assays | Decreased tPA antigen or activity Elevated PAI-1 antigen or activity | |||
Multifactorial | ||||||
APS | Anticardiolipid antibody titers (IgG, IgM) Anti-β2 GP-1 antibody titers (IgG, IgM) Lupus anticoagulant panel (at least two types of tests) | Moderately positive Moderately positive Present | 2%–5% | 12% | 19,20 | |
Myeloproliferative disorders | CBC | Elevated Hb or Hct Elevated platelet count |
Activated Protein C Resistance and Factor V Leiden. Resistance to aPC is characterized by reduced plasma protein C anticoagulant activity.21 This is most commonly caused by FVL, a point mutation that renders factor V more resistance to cleavage and inactivation by aPC.21 FVL affects 5% to 6% of persons of European descent and is associated with venous thrombosis, usually in high-risk settings. It is not a deficiency state, and more than 90% of heterozygous carriers will not experience thrombosis.21,22 Diagnosis is made by determination of the genetic status of the individual by polymerase chain reaction (PCR) methodology. FVL occurs as heterozygous and homozygous state. Heterozygote individuals have a fivefold increased risk of venous thrombosis; the risk among homozygotes is thought to be as high as 50% to 80%.23
The condition usually comes to an individual’s attention after a triggered event. Classic situations are a first thrombosis in young women on oral contraceptives or during pregnancy. Venous thrombosis and pulmonary embolism (PE) are the most common clinical manifestations. FVL is not a risk factor for arterial events.24
This genetic polymorphism is created by a point mutation in an untranslated portion of the prothrombin or factor II gene, which results in a modest increase in basal levels of functionally normal prothrombin.21 Between 2% and 5% of the white population carries the polymorphism in the heterozygous state, which imparts a modestly increased risk of venous thrombosis.25 The risk is estimated at three- to fourfold. As with FVL, the prevalence of this mutation is very low in African, Asian, and indigenous populations of Australia and America.26 Interestingly, numerous studies have shown that the prothrombin gene mutation is frequently co-inherited with FVL in symptomatic carriers of the FVL mutation.27
Endogenous Anticoagulant Deficiencies. A number of studies have been conducted to screen for the presence of endogenous anticoagulant deficiencies in patients with venous thrombosis. Classical, inherited antithrombin, protein C and protein S deficiencies are characterized by diminished activity levels of less than 50%, less than 40% to 50%, and 40% to 50%, respectively, and are generally associated with venous thrombosis.28,29 Accurate diagnosis of these disorders is compromised when testing is performed during an acute thrombotic episode, during pregnancy, in the setting of severe medical illness or inflammation, in the presence of antiphospholipid antibodies, or during anticoagulant therapy.21
Antithrombin is a multifunctional inhibitor of all active enzymes in the clotting cascade. By itself, it is a slow inhibitor, but glycosaminoglycans increase the inhibitory activity. Binding to antithrombin occurs via a pentasaccharide sequence, which is the basis for the anticoagulant activity of heparin.30
Antithrombin deficiency has not been described in the homozygous form type I (decreased protein concentration), suggesting that complete antithrombin deficiency is not compatible with life. Several type II (decreased protein activity) homozygotes have been described with mutations in the heparin-binding region of antithrombin.
Antithrombin deficiency should be suspected when patients present with thrombosis at a young age, with recurrent thrombosis, or with a family history of recurrent thrombosis.31 The initial event presents often after exposure to thrombotic risk factors such as surgery, pregnancy, postpartum state, trauma, immobilization, or oral contraceptive use. Thrombosis at unusual sites may occur. The severity of disease manifestation varies and is not related to the level of the deficient protein.32 Patients with congenital antithrombin deficiency have levels around 50% with little change during pregnancy or use of oral contraceptives. The lifetime risk of thrombosis has been estimated at 50% to 85%, and there is a clear age-dependent risk, with few thromboses seen before the third decade in life.33 Antithrombin deficiency is inherited in an autosomal dominant manner, and both genders are affected. The prevalence in the general population is one in 2000 to one in 3000.34 The prevalence of antithrombin deficiency in a large group of patients with venous thrombosis was 2.8%.31
Acquired antithrombin deficiency has been described in numerous clinical settings, including disseminated intravascular coagulation (DIC), liver disease, nephrotic syndrome, L-asparaginase chemotherapy. and oral contraceptive use.35
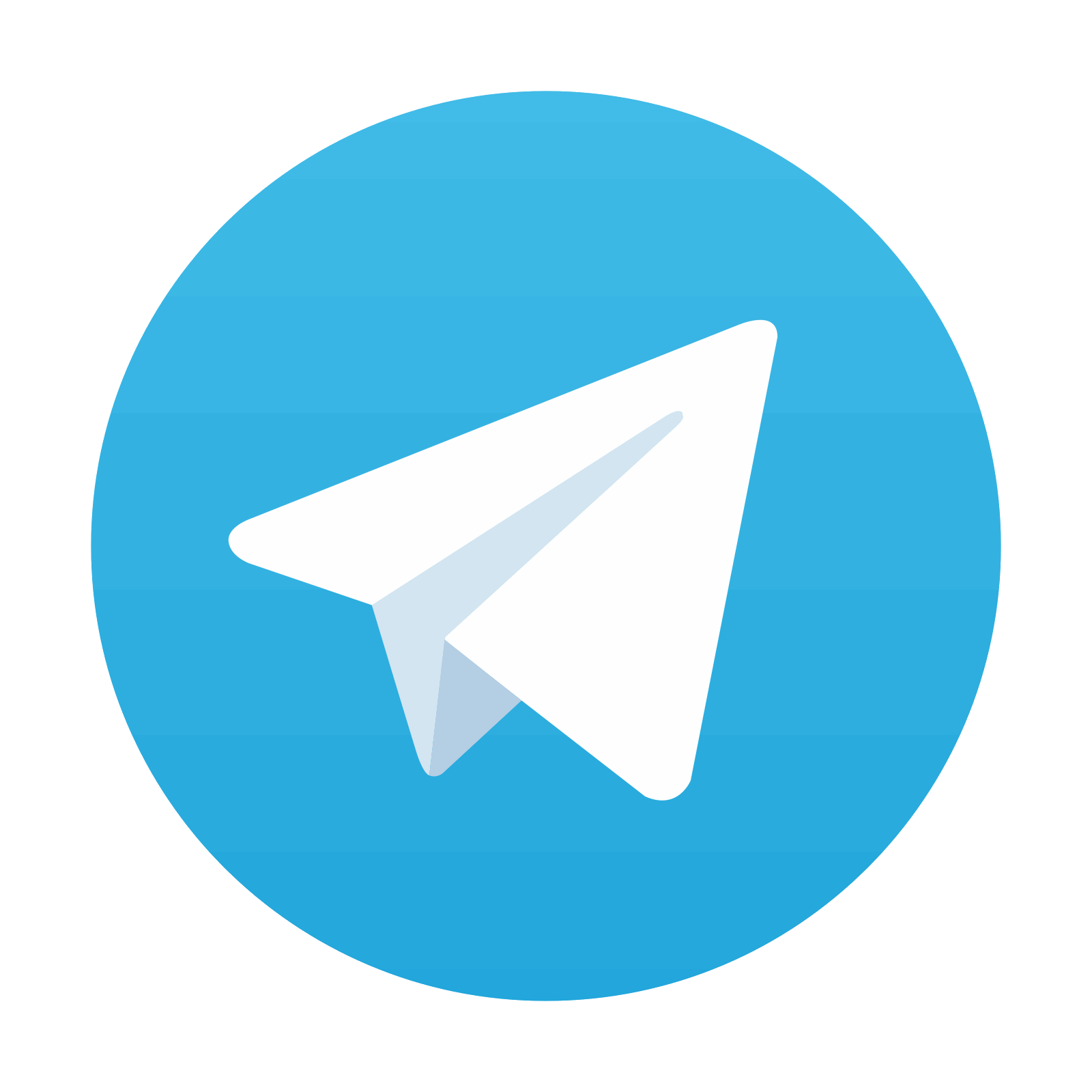
Stay updated, free articles. Join our Telegram channel

Full access? Get Clinical Tree
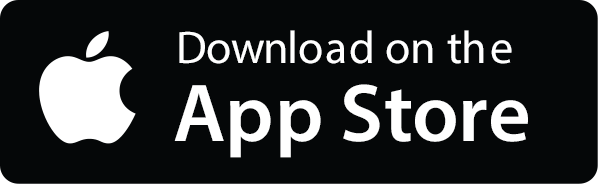
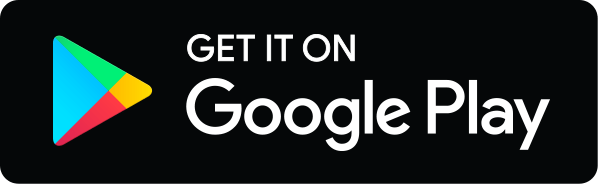