Abstract
Purpose
CAAS IntraVascular (CAAS-IV) is a recently released software that provides options for sophisticated quantitative coronary ultrasound (QCU) analysis. The aim of this study was to validate CAAS-IV for QCU in diseased human coronaries.
Methods
Ten preprocedural and 5 postprocedural IVUS studies were derived from daily practice. Intraobserver, interobserver, intersoftware (CAAS-IV vs. Curad) and interplatform (CAAS-IV vs. Volcano console) variability were assessed for cross-sectional area (CSA) measurements. Interobserver and intersoftware comparisons were made for volume measurements.
Results
Measurements of lumen, EEM, plaque and stent CSA demonstrated small differences in the intraobserver (0.0 ± 3.7%, − 0.7 ± 2.8%, − 0.5 ± 7.0% and − 0.9 ± 3.4%), interobserver (0.1 ± 4.4%, 0.1 ± 3.4%, − 0.5 ± 8.2% and − 0.8 ± 4.3%), intersoftware (− 0.3 ± 4.5%, 0.2 ± 2.4%, 0.4 ± 6.8% and − 0.5 ± 3.2%) and interplatform (0.7 ± 7.9%, 0.9 ± 4.0%, − 1.1 ± 12%, − 1.8 ± 3.6%) comparisons. For lumen, EEM, plaque and stent volume, the interobserver (− 2.1 ± 9.3%, 0.9 ± 5.6%, 3.4 ± 7.2% and − 0.2 ± 3.6%) and intersoftware (− 2.2 ± 6.2%, − 2.6 ± 6.1%, − 2.7 ± 12% and − 4.1 ± 3.2%) differences were substantially larger. Excluding large side-branches and calcifications, post-hoc measurements of lumen, EEM, plaque and stent volume showed small differences in the interobserver (− 0.3 ± 3.2%, 0.9 ± 2.4%, 2.9 ± 4.4% and − 1.3 ± 1.8%) and intersoftware (0.5 ± 2.5%, − 1.2 ± 1.7%, − 3.4 ± 5.1% and − 1.5 ± 2.2%) comparisons. Analysis time for entire pullbacks was reduced by 19.2 [14.9–30.0]% using CAAS-IV (p < 0.01).
Conclusions
CAAS-IV demonstrated reliable QCU with excellent agreement with previously validated software and the IVUS imaging console. Precision and reproducibility of measurements were high, proving CAAS-IV to be a valid option for QCU analysis in clinical practice and research. Interactive contour editing reduced analysis time by 20%.
1
Introduction
Intravascular ultrasound (IVUS) is a catheter-based invasive imaging technique that produces tomographic cross-sectional images of coronary artery lumen and wall, providing details on plaque burden and vascular dimensions . IVUS is being increasingly used in the catheterization laboratory for assessment of coronary artery disease and guidance of percutaneous coronary intervention (PCI). In cases where coronary angiography fails to identify the hemodynamic significance of coronary lesions, e.g. in left main coronary artery (LMCA) disease, IVUS can provide guidance in decision making . Moreover, IVUS can be performed to optimize percutaneous treatment strategy (e.g. adequate lesion preparation), device selection (e.g. sizing) and stent deployment, and to reduce complication rates such as suboptimal stent apposition and expansion which are known risk factors for stent thrombosis . In addition, IVUS is frequently used for research purposes to evaluate the performance of new devices and the progression/regression of coronary atherosclerotic burden in patients on investigational medical treatment .
As the options for quantitative coronary ultrasound (QCU) analysis are often limited on the imaging consoles, several software packages are commercially available that enable more sophisticated off line analysis of imaging data. These software packages provide semi-automatic contour detection of vessel compartments, reducing the time needed for pullback analysis and enabling volume calculations of coronary artery segments. Semi-automatic means that after initial manual input (i.e. a seed point) a contour tracing is performed which subsequently is fine-tuned manually by the user again. Recently, new QCU software has been launched under the name of ‘CAAS IntraVascular’ (Pie Medical Imaging, Maastricht, The Netherlands).
The aim of this study is to validate CAAS IntraVascular (CAAS-IV) in the clinical setting through assessment of intraobserver and interobserver variability for grey scale QCU measurements in diseased human coronary arteries, and comparing these measurements with those obtained by established and already validated QCU software (intersoftware variability) and the IVUS imaging console (interplatform variability).
2
Material and methods
2.1
IVUS image acquisition
All IVUS images were provided by the University Medical Center Utrecht and retrospectively selected after review for sufficient image quality. The IVUS studies were derived from daily clinical practice and were performed at discretion of the operators (mainly to guide LMCA in our center). Fifteen random IVUS pullbacks, obtained in 10 individual patients, were selected for this study. The IVUS investigations comprised 10 pullbacks prior to PCI and 5 after PCI (all including stent implantation).
Each IVUS study was conducted using the mechanical Volcano s5 console (Volcano Corp., CA, USA) equipped with a 45-MHz Revolution transducer catheter. Image acquisition was performed without electrocardiographic (ECG) gating, following intracoronary administration of 200 μg nitroglycerine at an automated pullback speed of 0.5 mm/s. All images were stored on the console and transferred to CD-ROM for offline-analysis.
2.2
IVUS analysis software
CAAS IntraVascular is a recently released software package that provides offline semi-automatic quantitative analysis of IVUS images. The user interface renders one cross-sectional and two orthogonal longitudinal views of the coronary artery that allow for delineation of three compartment outlines: lumen, external elastic membrane (EEM) and stent. After initial automatic contour tracing by the software, manual adjustments can be made in both longitudinal and cross-sectional views. In the longitudinal view, an infinite number of points can be added to correct for errors in the automatic contour analysis. In the cross-sectional view, 8 points are used to delineate compartment contours by default ( Fig. 1 A ), which can be relocated exclusively along the radius of the vessel. Freely movable points can be added on a per frame basis, if required for delineation of complex contours (e.g. in the case of erosions, ruptured plaques or dissections). Manual correction of irregularities in the cross-sectional and longitudinal contours is facilitated by interactive contour editing. This software feature provides the propagation of single manual contour adjustments to a modifiable number of neighboring frames through local reiteration of the automatic contour detection algorithm, reducing the need for frame by frame correction of erroneous initial tracings.

Curad Vessel Analysis (Curad, Curad BV, Amsterdam, The Netherlands) is established and validated software for quantitative analysis IVUS analysis , that has been previously used in several studies . This software package offers semi-automatic contour delineation that is comparable with CAAS-IV. Manual corrections can also be applied in both cross-sectional ( Fig. 1 B) and longitudinal views, but once adjustments to the cross-sectional contours have been made, the longitudinal contours will be disabled to further changes. This restriction is imposed to allow more freedom in cross-sectional contour delineation, as certain complex contours (e.g. in case of dissections) cannot be comprehensibly displayed in the longitudinal view. Consequentially, all points added for cross-sectional contour delineation are freely repositionable in Curad, providing high grade precision for tracing vessel contours. Drawback to this approach is the undesirable visual discrepancy between cross-sectional and longitudinal contours that will arise during vessel analysis. In addition, corrections involving many consecutive cross-sectionals, most easily performed on the longitudinal view, might not be possible when required. Furthermore, each cross-sectional contour has to be manually adjusted in this approach, as changes in the cross-sectional view are not transmitted in longitudinal direction to the next cross-sectional, potentially leading to an increase in analysis time.
Both CAAS-IV and Curad produce comprehensive reports with calculated compartment diameters, areas and volumes for selective frames and/or vessel segments. Cross-sectional areas are calculated by means of the pixel count within the contour. Longitudinal distances are derived from the automated pullback speed and the duration of pullback acquisition.
2.3
IVUS quantitative analysis
Quantitative analysis of the grey scale IVUS images was performed in compliance with the current American College of Cardiology Clinical Expert Document . Initially, two independent sessions of QCU analysis were performed: a single frame analysis to assess the validity of cross-sectional area (CSA) measurements and a pullback analysis to evaluate volumetric measurements.
For the single frame analysis, 4 random frames of each preprocedural IVUS were analyzed for lumen, EEM and plaque CSA, and 8 random frames of each postprocedural IVUS for stent CSA, resulting in a total of 40 analyzed frames for both preprocedural and postprocedural IVUS studies. All frames had to be separated by at least 100 frames. Alternative frames were randomly selected when reliable analysis was impeded by the presence of bifurcations, artifacts or extensive calcifications that caused severe signal attenuation. The single frame lumen, EEM and stent CSA were measured by semi-automatic tracing of contours. After automatic detection of compartment contours in four longitudinal views, equally divided across the vessel radius, precise manual adjustments were made in the cross-sectional views of the frames selected for single frame analysis. Recognition of lumen boundaries was facilitated during manual corrections by cinematic looping of pullback images around the frame of interest, taking advantage of the speckling of blood to distinguish blood from tissue. Plaque CSA was derived from subtraction of EEM and lumen CSA, and actually represents the media and plaque complex.
For the pullback analysis, one predefined vessel segment in each preprocedural IVUS was analyzed for lumen, EEM and plaque volume. In each postprocedural IVUS the stent was analyzed for stent volume. The requirements for the predefined vessel segments were: a minimum length of 1250 frames (20.0 mm) and inclusion of the target lesion. The stent was identified by the first and last cross-sections of the pullback in which the stent struts could be circumferentially designated. Delineation of contours was performed using both longitudinal and cross-sectional views, taking full advantage of the software’s abilities of semi-automatic contour detection, limiting manual adjustments to a minimum. Frame skipping, the repetitive omission of a pre-set number of frames, was enabled during analysis and set to 5 frames (0.08 mm). Side-branches were excluded from the pullback analysis by visual interpolation of the lumen and EEM contours distal and proximal to the side-branch. On completion of contour tracing, compartment volumes were documented as reported by the software. The frame numbers and values of the minimum lumen area (MLA) and minimal stent area (MSA) were automatically derived from lumen and stent contour data by the software. The difference in reported MLA frame number amongst observers and software was defined as ‘geographic miss’, and reported without accounting for frame skipping. The time required for image analysis, excluding the time needed for loading the imaging data, was measured for comparison purposes.
Hereafter, a post-hoc volumetric QCU analysis was performed to assess measurement reproducibility in selected pullback segments in which the lumen and vessel wall contours are clearly distinguishable without uncertainty in contour definition or shadowing effects because of large amounts of calcium or large side branches. The post-hoc analysis was performed for 2 selected vessel segments (n = 20) of each preprocedural pullback and 2 selected stent segments (n = 10) for each postprocedural pullback. Care has been taken to select segments without severe calcifications, large side-branches or artifacts importantly hampering automatic image analysis, while accommodating a length of approximately 250 frames (4.0 mm). The analysis was performed with frame skipping enabled at 5 frames. Manual adjustment of contours on a per frame basis was mandatory.
The single frame QCU analyses were performed in CAAS-IV by two independent observers (observer 1a and 2). The exact same analyses were thereafter repeated by observer 1b in CAAS-IV, Curad and on the Volcano S5 console. Each analysis session was followed by an interval of 4 weeks to avoid measurement bias. The pullback analysis was performed by two observers (observer 1a and 2) and repeated after the aforementioned interval in Curad (observer 1a). The post-hoc analysis was performed according to the same routine as the pullback analysis.
2.4
Statistical analysis
Data were analyzed using IBM SPSS Statistics software version 20 (IBM Corp., Armonk, NY). Results for continuous variables are presented as means ± SD or medians [interquartile range] as considered appropriate. Categorical data are presented as counts and percentages. Intraobserver (observer 1a vs. observer 1b), interobserver (observer 1a vs. observer 2), intersoftware (CAAS-IV vs. Curad) and interplatform (CAAS-IV vs. the Volcano s5 Console) variability were evaluated by calculating the mean difference ± SD and the percentage difference (using the formula ((measurement 1-measurement 2)/measurement 1)*100) for repeated measurements. Statistical significance of the difference between two measurements was assessed with the paired sample T-test or the Wilcoxon signed ranks test, depending on normality and sample size. Agreement of repeated measurements was further assessed by two way random intraclass correlation coefficient (ICC), simple linear regression and construction of Bland–Altman plots with limits of agreement (mean difference + 1.96*SD for the upper limit and mean difference –1.96*SD for the lower limit). A p-value < 0.05 was regarded statistically significant.
2
Material and methods
2.1
IVUS image acquisition
All IVUS images were provided by the University Medical Center Utrecht and retrospectively selected after review for sufficient image quality. The IVUS studies were derived from daily clinical practice and were performed at discretion of the operators (mainly to guide LMCA in our center). Fifteen random IVUS pullbacks, obtained in 10 individual patients, were selected for this study. The IVUS investigations comprised 10 pullbacks prior to PCI and 5 after PCI (all including stent implantation).
Each IVUS study was conducted using the mechanical Volcano s5 console (Volcano Corp., CA, USA) equipped with a 45-MHz Revolution transducer catheter. Image acquisition was performed without electrocardiographic (ECG) gating, following intracoronary administration of 200 μg nitroglycerine at an automated pullback speed of 0.5 mm/s. All images were stored on the console and transferred to CD-ROM for offline-analysis.
2.2
IVUS analysis software
CAAS IntraVascular is a recently released software package that provides offline semi-automatic quantitative analysis of IVUS images. The user interface renders one cross-sectional and two orthogonal longitudinal views of the coronary artery that allow for delineation of three compartment outlines: lumen, external elastic membrane (EEM) and stent. After initial automatic contour tracing by the software, manual adjustments can be made in both longitudinal and cross-sectional views. In the longitudinal view, an infinite number of points can be added to correct for errors in the automatic contour analysis. In the cross-sectional view, 8 points are used to delineate compartment contours by default ( Fig. 1 A ), which can be relocated exclusively along the radius of the vessel. Freely movable points can be added on a per frame basis, if required for delineation of complex contours (e.g. in the case of erosions, ruptured plaques or dissections). Manual correction of irregularities in the cross-sectional and longitudinal contours is facilitated by interactive contour editing. This software feature provides the propagation of single manual contour adjustments to a modifiable number of neighboring frames through local reiteration of the automatic contour detection algorithm, reducing the need for frame by frame correction of erroneous initial tracings.

Curad Vessel Analysis (Curad, Curad BV, Amsterdam, The Netherlands) is established and validated software for quantitative analysis IVUS analysis , that has been previously used in several studies . This software package offers semi-automatic contour delineation that is comparable with CAAS-IV. Manual corrections can also be applied in both cross-sectional ( Fig. 1 B) and longitudinal views, but once adjustments to the cross-sectional contours have been made, the longitudinal contours will be disabled to further changes. This restriction is imposed to allow more freedom in cross-sectional contour delineation, as certain complex contours (e.g. in case of dissections) cannot be comprehensibly displayed in the longitudinal view. Consequentially, all points added for cross-sectional contour delineation are freely repositionable in Curad, providing high grade precision for tracing vessel contours. Drawback to this approach is the undesirable visual discrepancy between cross-sectional and longitudinal contours that will arise during vessel analysis. In addition, corrections involving many consecutive cross-sectionals, most easily performed on the longitudinal view, might not be possible when required. Furthermore, each cross-sectional contour has to be manually adjusted in this approach, as changes in the cross-sectional view are not transmitted in longitudinal direction to the next cross-sectional, potentially leading to an increase in analysis time.
Both CAAS-IV and Curad produce comprehensive reports with calculated compartment diameters, areas and volumes for selective frames and/or vessel segments. Cross-sectional areas are calculated by means of the pixel count within the contour. Longitudinal distances are derived from the automated pullback speed and the duration of pullback acquisition.
2.3
IVUS quantitative analysis
Quantitative analysis of the grey scale IVUS images was performed in compliance with the current American College of Cardiology Clinical Expert Document . Initially, two independent sessions of QCU analysis were performed: a single frame analysis to assess the validity of cross-sectional area (CSA) measurements and a pullback analysis to evaluate volumetric measurements.
For the single frame analysis, 4 random frames of each preprocedural IVUS were analyzed for lumen, EEM and plaque CSA, and 8 random frames of each postprocedural IVUS for stent CSA, resulting in a total of 40 analyzed frames for both preprocedural and postprocedural IVUS studies. All frames had to be separated by at least 100 frames. Alternative frames were randomly selected when reliable analysis was impeded by the presence of bifurcations, artifacts or extensive calcifications that caused severe signal attenuation. The single frame lumen, EEM and stent CSA were measured by semi-automatic tracing of contours. After automatic detection of compartment contours in four longitudinal views, equally divided across the vessel radius, precise manual adjustments were made in the cross-sectional views of the frames selected for single frame analysis. Recognition of lumen boundaries was facilitated during manual corrections by cinematic looping of pullback images around the frame of interest, taking advantage of the speckling of blood to distinguish blood from tissue. Plaque CSA was derived from subtraction of EEM and lumen CSA, and actually represents the media and plaque complex.
For the pullback analysis, one predefined vessel segment in each preprocedural IVUS was analyzed for lumen, EEM and plaque volume. In each postprocedural IVUS the stent was analyzed for stent volume. The requirements for the predefined vessel segments were: a minimum length of 1250 frames (20.0 mm) and inclusion of the target lesion. The stent was identified by the first and last cross-sections of the pullback in which the stent struts could be circumferentially designated. Delineation of contours was performed using both longitudinal and cross-sectional views, taking full advantage of the software’s abilities of semi-automatic contour detection, limiting manual adjustments to a minimum. Frame skipping, the repetitive omission of a pre-set number of frames, was enabled during analysis and set to 5 frames (0.08 mm). Side-branches were excluded from the pullback analysis by visual interpolation of the lumen and EEM contours distal and proximal to the side-branch. On completion of contour tracing, compartment volumes were documented as reported by the software. The frame numbers and values of the minimum lumen area (MLA) and minimal stent area (MSA) were automatically derived from lumen and stent contour data by the software. The difference in reported MLA frame number amongst observers and software was defined as ‘geographic miss’, and reported without accounting for frame skipping. The time required for image analysis, excluding the time needed for loading the imaging data, was measured for comparison purposes.
Hereafter, a post-hoc volumetric QCU analysis was performed to assess measurement reproducibility in selected pullback segments in which the lumen and vessel wall contours are clearly distinguishable without uncertainty in contour definition or shadowing effects because of large amounts of calcium or large side branches. The post-hoc analysis was performed for 2 selected vessel segments (n = 20) of each preprocedural pullback and 2 selected stent segments (n = 10) for each postprocedural pullback. Care has been taken to select segments without severe calcifications, large side-branches or artifacts importantly hampering automatic image analysis, while accommodating a length of approximately 250 frames (4.0 mm). The analysis was performed with frame skipping enabled at 5 frames. Manual adjustment of contours on a per frame basis was mandatory.
The single frame QCU analyses were performed in CAAS-IV by two independent observers (observer 1a and 2). The exact same analyses were thereafter repeated by observer 1b in CAAS-IV, Curad and on the Volcano S5 console. Each analysis session was followed by an interval of 4 weeks to avoid measurement bias. The pullback analysis was performed by two observers (observer 1a and 2) and repeated after the aforementioned interval in Curad (observer 1a). The post-hoc analysis was performed according to the same routine as the pullback analysis.
2.4
Statistical analysis
Data were analyzed using IBM SPSS Statistics software version 20 (IBM Corp., Armonk, NY). Results for continuous variables are presented as means ± SD or medians [interquartile range] as considered appropriate. Categorical data are presented as counts and percentages. Intraobserver (observer 1a vs. observer 1b), interobserver (observer 1a vs. observer 2), intersoftware (CAAS-IV vs. Curad) and interplatform (CAAS-IV vs. the Volcano s5 Console) variability were evaluated by calculating the mean difference ± SD and the percentage difference (using the formula ((measurement 1-measurement 2)/measurement 1)*100) for repeated measurements. Statistical significance of the difference between two measurements was assessed with the paired sample T-test or the Wilcoxon signed ranks test, depending on normality and sample size. Agreement of repeated measurements was further assessed by two way random intraclass correlation coefficient (ICC), simple linear regression and construction of Bland–Altman plots with limits of agreement (mean difference + 1.96*SD for the upper limit and mean difference –1.96*SD for the lower limit). A p-value < 0.05 was regarded statistically significant.
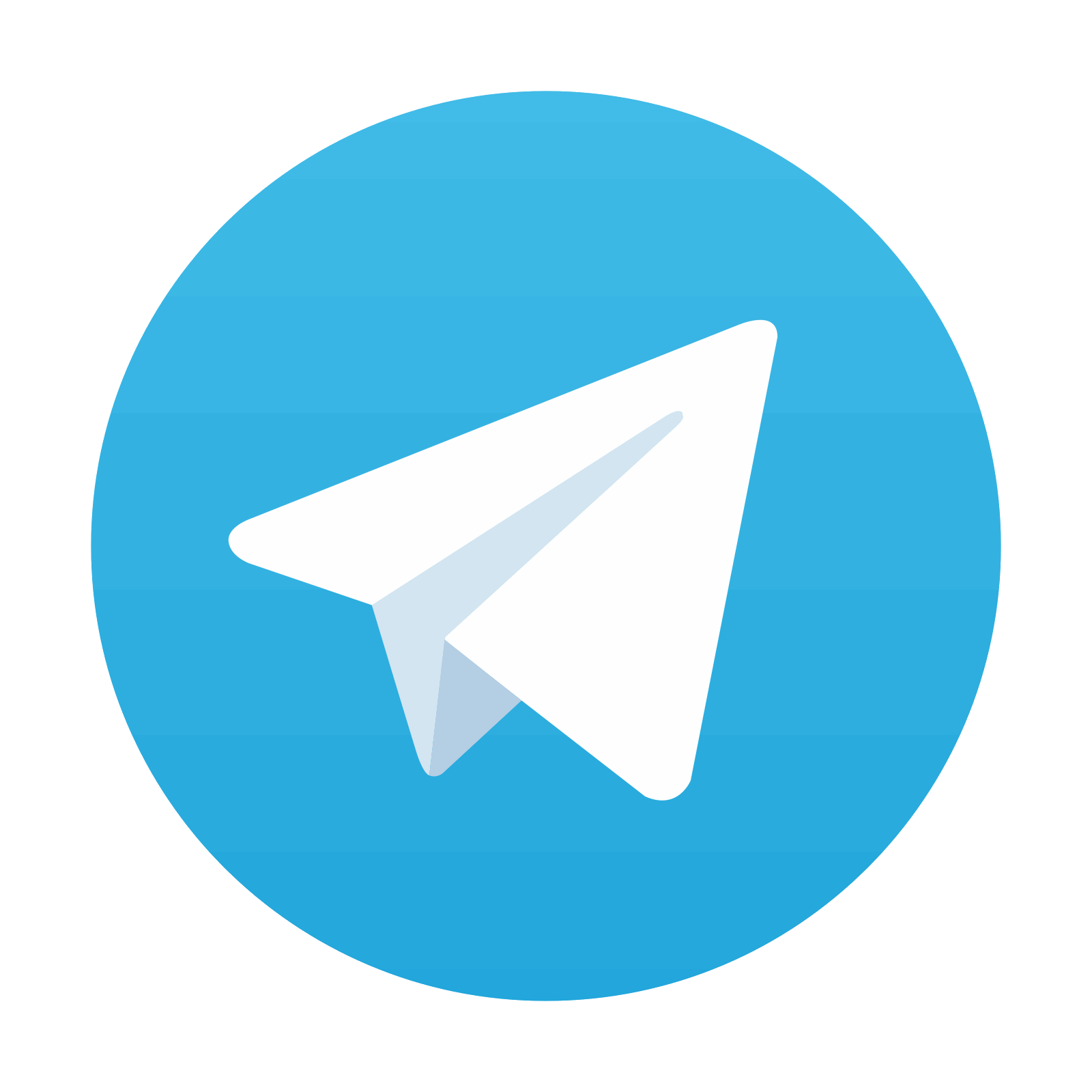
Stay updated, free articles. Join our Telegram channel

Full access? Get Clinical Tree
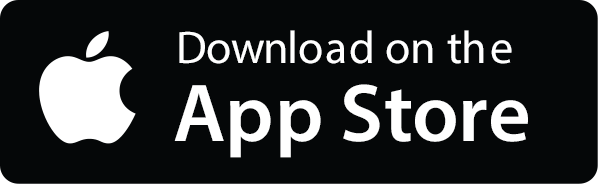
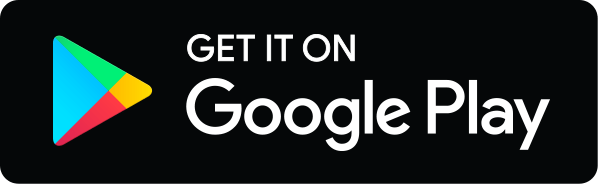