Fig. 9.1
Cachectic patient in right semilateral decubitus position in preparation to undergo retroperitoneal transaortic endarterectomy
Other symptoms are vague and nonspecific. They include nausea and vomiting, fullness, and right upper quadrant discomfort. These symptoms are believed to be caused by hypoperfusion of the celiac artery (CA) territory rather than the superior mesenteric artery (SMA) territory leading to ischemic gastropathy. They occur in about 15 % of cases. Finally, changes in bowel habits can occur. Hematochezia and gastrointestinal bleeding due to CMI are rare.
Atherosclerosis is responsible for the majority of patients with CMI. Thus classical risk factors for atherosclerosis can often be elicited from the patients. In fact, half of the patients have known atherosclerosis in other vascular beds such as peripheral or coronary arteries [10]. Another study found the following comorbidities and risk factors at the time of presentation: hypertension 91 %, peripheral vascular disease 86 %, coronary artery disease 82 % with previous myocardial infarction 46 %, diabetes mellitus 66 %, hypercholesterolemia 48 %, and heavy smoking of >25 pack years 53 %.
The findings on physical examination are rather nonspecific. Often patients are underweight, sometimes to the point of cachexia due to poor oral intake related to food fear. Occasionally one can auscultate a bruit over the origin of the mesenteric vessels in the epigastrium. The bowel sounds, however, are frequently hyperactive. On palpation of the abdomen, there is rarely tenderness. Signs of peritonitis such as guarding or rebound tenderness are rarely found and suggest the possibility of acute or acute-on-chronic mesenteric ischemia. Similar to the physical examination, laboratory findings are nonspecific and often normal. Markers for the nutritional state of the patient such as albumin and prealbumin for long-term and short-term caloric intake are the exception and are low in many cases.
Etiology
Chronic mesenteric ischemia is usually caused by atherosclerotic changes of the three mesenteric vessels. However, as mentioned earlier, most patients have involvement of at least two vessels to cause clinically evident disease. According to some estimations, up to 95 % of cases of CMI are due to atherosclerosis [9]. The atherosclerotic changes occur at the ostium of the affected vessel. These changes are also found on the anterior aortic wall, and one might consider the ostial lesions of the mesenteric vessels as progressive aortic disease. This probably also explains the rare occurrence of isolated atherosclerosis-related disease of only one vessel.
Atherogenesis describes the multistep process of forming atheromatous plaques in arteries [14]. A brief synopsis of the involved steps is given below (Fig. 9.2). An early event in this process is the activation of endothelial cells (ECs). Usually resisting leukocyte attachment, ECs respond to irritants such as hypertension or dyslipidemia by upregulation of cell adhesion molecules. Changes in endothelial permeability also lead to increased deposition of cholesterol-containing low-density lipoprotein (LDL) particles. Together with other chemoattractants, this leads to eventual leukocyte adhesion and migration to deep layers of the arterial wall. There, blood monocytes become tissue-resident macrophages and take up LDL. On pathological examination these macrophages have taken up such large amounts of lipid that they become foam cells. Foam cells and other cells of the immune system then further stimulate the process by releasing pro-inflammatory cytokines such as interleukin-1β (IL-1β) and tumor-necrosis factor (TNF). Smooth muscle cells from the intima as well as the media proliferate in response to these stimuli and produce extracellular matrix proteins including collagen and elastin, which form a fibrous cap over the plaques. This cap covers viable as well as apoptotic foam cells and extracellular debris and lipids. Over time the plaque grows and causes clinical manifestations by producing flow-limiting stenoses or by thrombus formation with distal embolization.
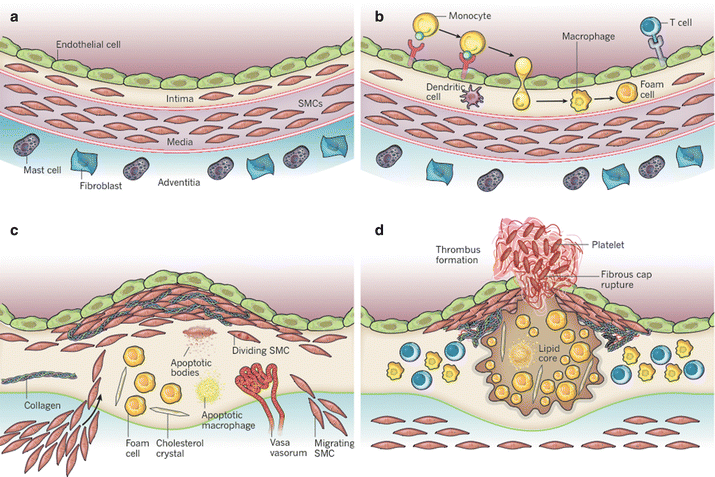
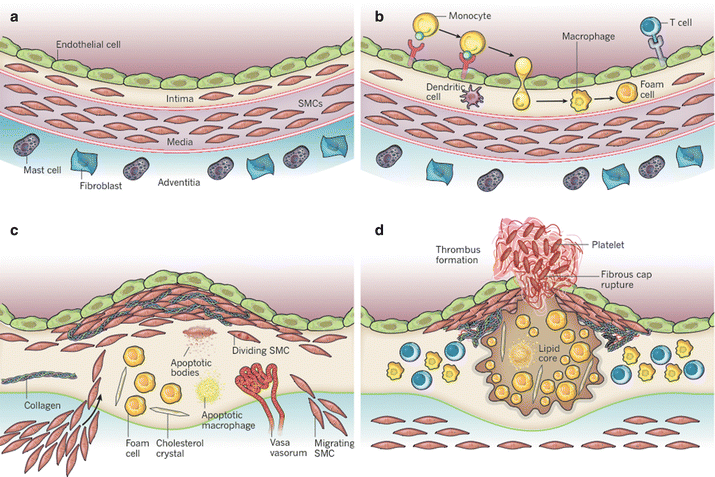
Fig. 9.2
Stages of development of atherosclerotic plaques and eventual rupture. (a) The normal muscular artery with its three layers. (b) Activation of ECs and subsequent attraction of monocytes, which eventually become foam cells. (c) Proliferation of smooth muscle cells and production of extracellular matrix proteins after cytokine stimulation by foam cells and other immune cells. (d) fracture of a fibrous cap (Adapted from Libby et al. [14], with permission of Macmillan Publishers, Ltd)
Beyond atherosclerotic changes causing mesenteric ischemia, there are a variety of systemic diseases that can affect the mesenteric vasculature. The prevalence of mesenteric compromise related to these diseases is difficult to estimate but certainly much lower than atherosclerosis as an etiology as mentioned above. Most available data are derived from case reports or small case series. A study from the Mayo Clinic seems to confirm this notion [15]. Over a period of 24 years, they treated only 15 patients (13 female and 2 male) for occlusive mesenteric vasculitis. Etiologies were Takayasu’s arteritis in 7, polyarteritis nodosa in 4, indeterminate in 3, and giant cell arteritis in 1. The majority of patients underwent open revascularization. A few interesting points can be extrapolated from this paper. Patients with vasculitis as the underlying etiology are significantly younger than patients with mesenteric ischemia due to atherosclerosis. The mean age was 38 versus 65. These patients lack the classic cardiovascular risk factors of hypertension, hyperlipidemia, diabetes, and smoking. Finally, the Mayo experience confirms the low prevalence of mesenteric vasculitis (15 patients with vasculitis versus 163 patients with atherosclerosis).
Takayasu’s arteritis was the most common form of vasculitis in this series. This finding is further supported by the majority of case reports on this subject [15]. Min et al, for example, report their experience with Takayasu’s disease in 25 patients [16]. Two of them had involvement of the mesenteric vessels and were treated with stenting. Similarly, Kalangos et al report on 10 pediatric patients of whom 4 underwent endovascular or open repair of the SMA to treat ischemic symptoms [17].
Thrombangiitis obliterans, also known as Buerger’s disease, is another inflammatory process that can lead to occlusion of the intestinal arteries over time. It is associated with smoking and usually affects the peripheral arterial beds [18]. It has long been known, though, that Buerger’s disease can affect the visceral arteries on rare occasions [19]. Data are limited to case reports. Pfitzmann et al describe such a rare event. A patient with abdominal pain was found to have necrotic small bowel and hepatic hypoperfusion upon laparotomy [20]. Angiography eventually showed occlusion of celiac trunk and SMA. He was revascularized by SMA thrombectomy and vein bypass from SMA to hepatic artery.
Giant cell arteritis can also cause mesenteric ischemia [21]. Evans et al found smooth narrowing of the SMA and confirmed the diagnosis by temporal artery biopsy. The patient was started on steroids and improved slowly over a 6-month period. Other patients with giant cell arteritis can present more dramatically with intestinal infarction or perforation and subsequent mortality as high as 30 % [22, 23].
Other forms of vasculitis can cause mesenteric ischemia infrequently. Chubachi et al describe a patient with occlusive Behcet disease [24]. Churg-Strauss syndrome, Wegener’s granulomatosis, microscopic polyangiitis, and other small-vessel arteriopathies can also on occasion affect the mesenteric circulation [25].
There are other unusual etiologies for mesenteric ischemia. Often there are only one or two patients described with a particular pathology. Krupski reviewed them in detail [26]. One patient suffered from an isolated spontaneous SMA dissection and presented with classic symptoms of CMI [27]. Resection of the intimal flap with vein patch angioplasty was performed with good result. Retroperitoneal fibrosis and neurofibromatosis can also cause mechanical obstruction by external compression [28, 29]. Median arcuate ligament syndrome is similarly recognized as an external lesion leading to external mechanical obstruction of the celiac trunk with ensuing ischemic symptoms. The syndrome is discussed in detail elsewhere in this book.
Diagnostic Considerations
The diagnosis of CMI remains a formidable challenge to the medical practitioner. It requires careful history taking to suspect mesenteric ischemia in a patient with vague abdominal symptoms. It has been shown, however, that the establishment of a multidisciplinary splanchnic diseases workgroup can lead to increased detection of CMI [30]. This Dutch group was able to increase the rate of diagnosis from 7 to 23 persons per million per year due to an increased degree of suspicion and an extensive investigation by several specialists.
Beyond a thorough history, the key to making a tentative diagnosis of CMI lies with various imaging modalities as well as physiologic testing. These imaging modalities include noninvasive techniques such as duplex ultrasonography (DUS), computed tomographic angiogram (CTA), and magnetic resonance angiography (MRA) and invasive techniques like conventional angiography, which served for a long time as the gold standard. Tonometry as a physiologic test has been employed to detect pH changes after stimulation. These tests can be used alone or in combination but even then a preoperative definitive diagnosis is impossible [9]. They can suggest a high confidence of the correctness of the diagnosis, but only the improvement of symptoms after a successful intervention, either open or endovascular, confirms the diagnosis definitively.
Angiography
Biplanar selective angiography is the gold standard for detecting CMI against which all other modalities are tested (Fig. 9.3). It used to be the only test available to aid in diagnosis but has largely been replaced by newer technologies as outlined below. It is employed in therapeutic efforts, though, and in this capacity has gained importance due to the increase in endovascular treatment of CMI. If performed for diagnostic purposes, it is crucial to obtain several views of the ostia of mesenteric vessels. Plain anterior-posterior (AP) views do not allow for visualization of the vessel origins. Lateral views are better suited to assess this area of the vessel where most disease is located. Imaging should be performed early and late: early to visualize the CA and SMA origin and late to evaluate the retrograde flow, delayed proximal visualization, and collateral pathways between CA, SMA, and inferior mesenteric artery (IMA) [31].
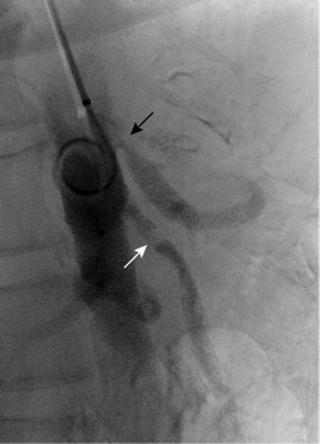
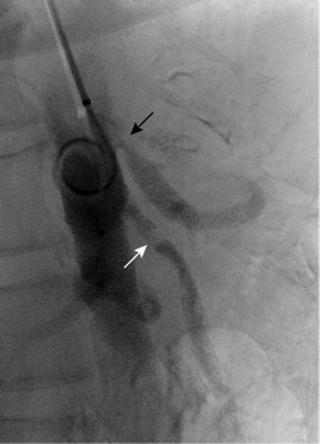
Fig. 9.3
Lateral projection aortogram with proximal mesenteric vessels (CA and SMA). Note the significant stenosis of the CA with post-stenotic dilation (black arrow) and the reduced intensity of contrast in SMA indicative of stenosis (white arrow). Further information can be obtained from selective cannulation of single vessels
Duplex Ultrasonography
Gene Strandness and colleagues at the University of Washington in Seattle demonstrated 30 years ago the utility of duplex ultrasonography (DUS) to detect stenoses and occlusions of mesenteric vessels [32]. This group found elevated velocities of CA and SMA in a patient with postprandial abdominal pain and weight loss. Angiography confirmed the diagnosis. Since that time DUS has become the foremost screening tool to assess CMI due to its low cost, high speed, and general availability (Fig. 9.4). Starting in the early 1990s, several groups published retrospective reviews to establish velocity parameters and thresholds that would reliably predict critical stenoses of the mesenteric vessels. Moneta et al first published duplex criteria for diagnosis of SMA or CA stenosis in 1991 [33]. They used peak systolic velocities (PSV) and found that velocities >275 cm/s for the SMA and >200 cm/s for the CA predicted stenosis of at least 70 %. Sensitivity, specificity, and positive predictive values were 89, 92, and 80 % for the SMA and 75, 89, and 85 % for the CA, respectively. In their hands end-diastolic velocities (EDV) or calculated velocity ratios did not add additional accuracy to the test. This is in contrast to the data from Dartmouth where the authors found EDV more accurate than PSV in the diagnosis of SMA stenosis [34]. They reported an EDV > 45 cm/s in the SMA to be the best indicator of severe stenosis (sensitivity 100 %; specificity 92 %). In their hands, PSV > 300 cm/s was less sensitive (63 %) but highly specific (100 %) for severe SMA stenosis. They also reported issues with imaging the CA and correctly identifying threshold values. This was attributed to increased collateral flow through the gastroduodenal artery that would reduce the velocities across a CA lesion. In a later publication, the authors proved this to be the case and reported reversal of flow in the common hepatic artery to predict severe CA stenosis or occlusion [35].
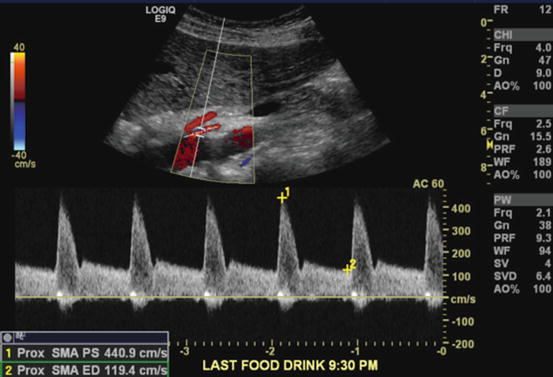
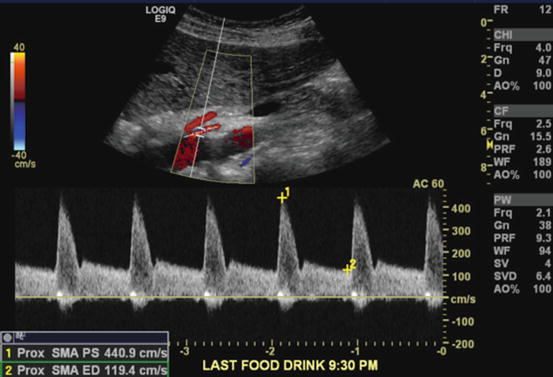
Fig. 9.4
Duplex ultrasonography examination of patient with symptoms of abdominal pain, weight loss, and food fear. DUS shows laminar flow in the aorta and CA. There is, however, turbulent flow at the SMA origin indicative of a stenosis, which was confirmed by pulse waveform analysis of this area. This showed PSV of 440.9 cm/s, a significantly elevated value
Shortly after publishing the diagnostic threshold criteria using PSV, Moneta et al published a prospective study validating their initial results [36]. They studied 100 patients who underwent routine aortography and subsequently had DUS. Sensitivity, specificity, positive predictive value, negative predictive value, and overall accuracy for detection of a 70 % or greater SMA stenosis were 92, 96, 80, 99, and 96 % and for a 70 % or greater CA stenosis 87, 80, 63, 94, and 82 %, respectively. Similarly, Zwolak et al confirmed the Dartmouth criteria using EDV to diagnose stenoses [37]. An EDV threshold of 45 cm/s had a sensitivity of 90 %, specificity of 91 %, positive predictive value of 90 %, negative predictive value of 91 %, and overall accuracy of 91 % for detecting SMA lesions. For the CA, a threshold of 55 cm/s or no flow signal had best overall accuracy (95 %) with high sensitivity (93 %) and specificity (100 %). They also confirmed that a CA PSV > 200 cm/s or no signal has excellent accuracy (93 %), sensitivity (93 %), and specificity (94 %). The PSV data for the SMA were less convincing. It provided low overall accuracy (81 %), low sensitivity (60 %), but high specificity (100 %). The parameters that were established by both groups have been used for the last 2 decades to screen for and diagnose critical lesions of the mesenteric vessels. Recently though, a study by AbuRahma et al questioned these thresholds [38]. They found PSV > 295 cm/s to provide the highest accuracy for detecting SMA stenosis > 50 % and PSV > 400 cm/s for SMA stenosis > 70 %. The sensitivity for a 50 % stenosis was 87 %, specificity was 89 %, and overall accuracy 88 %, and for detecting a 70 % stenosis, it was 72, 93, and 85 %, respectively. Finally, DUS is a dynamic examination that relies on operator expertise as well as patient factors such as respirations, body habitus, timing of last meal, bowel gas, and anatomic variations [39].
The role for duplex extends beyond the initial diagnosis. Several studies show the utility of this powerful technology to follow patients after interventions. This allows for timely recognition of restenosis before it becomes clinically apparent and thus provides a window for a second intervention. There is, however, no clearly defined threshold for re-intervention. Baker et al document their experience of pre- and postprocedural duplex on 23 patients [40]. The mean PSV before intervention was 464 cm/s while it dropped to 335 cm/s afterwards. This number is still higher than the threshold of 275 cm/s used to diagnose the critical stenosis in the first place. From these rather preliminary data, they concluded that a threshold of PSV > 500 cm/s inside the stent or a change from baseline PSV obtained at 1-month follow-up should be used to trigger a re-intervention. Schoch et al come to a similar conclusion in their review of 107 patients having undergone endovascular therapy [41]. Eighty-three percent of patients had recurrent stenosis on surveillance duplex but 53 % remained asymptomatic. Thus clinical context and change from early baseline PSV values should trigger an intervention.
Computed Tomographic Angiography
Computed Tomographic Angiography (CTA) is a modern, fast, and accurate imaging modality to visualize the mesenteric vessels and their pathology. Especially since the introduction of multi-row detector technology in the late 1990s, the quality of temporal and spatial resolution is excellent [42].
Compared to conventional angiography, it allows for more complete assessment of the mesenteric vasculature [43]. It can be viewed in multiple planes and may include three-dimensional (3D) reconstructions (Fig. 9.5). CTA depicts vascular narrowing as well as the atherosclerotic plaque itself. A normal CTA virtually rules out CMI [44]. Finally, the CT part of the study enables evaluation of the bowel at the same time. Thickening of the bowel wall can indicate inflammatory changes associated with ischemia. A late finding of severe ischemic is the presence of pneumatosis intestinalis, air within the bowel wall. This might not be as critical for CMI as for AMI but is certainly beneficial and lends to a more thorough assessment. Similarly to conventional angiography, early and late phase images can be reacquired and thus provide more information. Late phase sequences examine the venous system including IMV, SMV, splenic vein, and portal vein.
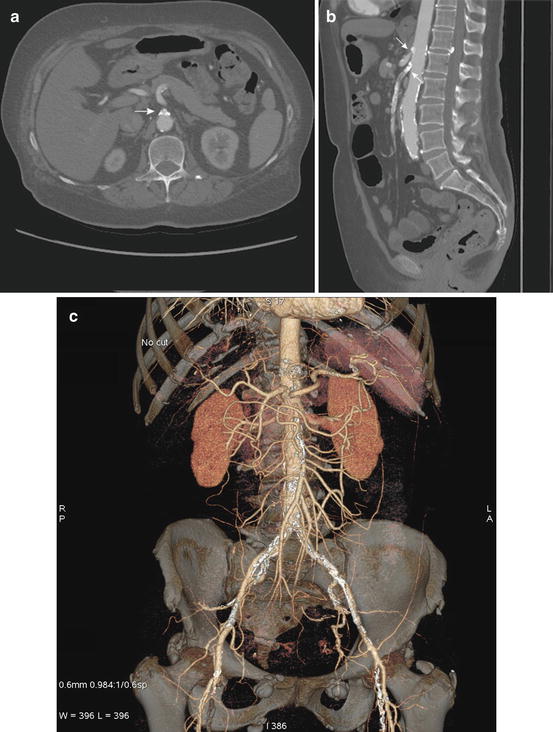
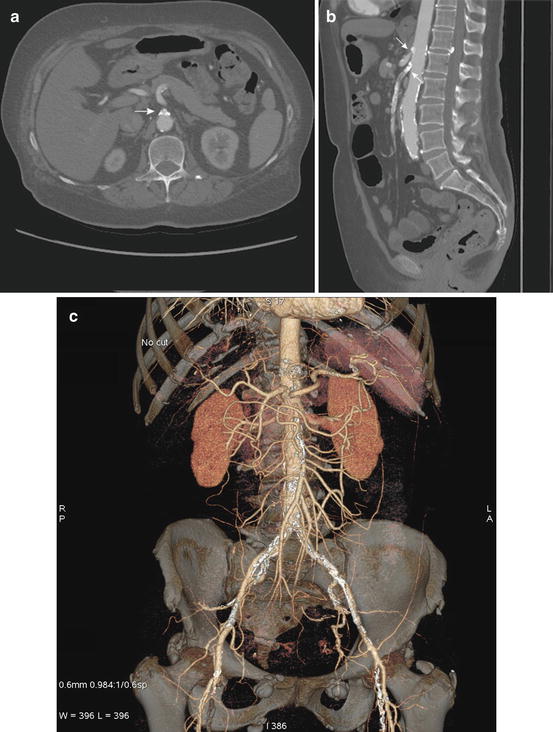
Fig. 9.5
(a–c) Axial and sagittal reconstructions of CTA. (a) The axial view is well suited to assess calcifications, stenoses, and occlusions at the origin of the mesenteric vessels as seen here (white arrow). (b) The sagittal reformat is particularly helpful in assessing the length of a stenosis as it allows for depicting the vessel in a longitudinal section. (c) Finally, 3D reconstructions are possible from CTA raw data that permits complete assessment of the mesenteric vasculature. These images can be viewed in all planes and help in preparation of endovascular interventions, e.g., choice of ideal projection angles to see the stenosis during angiography
Other findings include calcified and noncalcified atherosclerotic plaque, typically in the CA and SMA and less commonly in the IMA. The plaque is usually found in the proximal segment of the vessel within a few centimeters from its origin [45]. CTA is the superior modality to evaluate the plaque in detail as this has implications for treatment such as clamp placement and choice of inflow and target. Through adjustments of the window and level, and comparison to non-contrast images, calcified plaque can usually be distinguished from contrast. Changes in vessels in the proximity of a stenosis can increase the certainty of the diagnosis. One can detect the presence of collateral pathways, e.g., prominent vessels around the head of the pancreas that should raise immediate suspicion of a hemodynamically significant stenosis of the CA resulting in dilatation of the pancreaticoduodenal arteries [43]. This is somewhat similar to DUS, which can also assess the proximity of the stenotic vessel and detect ante- and retrograde flow in the hepatic artery. Detection of flow, however, is not possible with CTA, and only indirect findings such as the development of collaterals can hint at changes in flow.
Several studies evaluated the sensitivity and specificity of CTA compared with conventional angiography as gold standard. Cikrit et al performed a retrospective review of 32 patients, who had both angiography and CTA to evaluate stenoses of mesenteric arteries [46]. Spiral CTA had a sensitivity of 75 % and specificity of 100 % for detection of a 75 % celiac arterial stenosis. For detection of a similar stenosis in the SMA, sensitivity was 100 % and specificity was 91 %. By means of the Pearson correlation coefficient, significant correlation (p < 0.001) was confirmed between spiral CT and arteriography for evaluation of stenosis of the SMA (r = 0.8991) and celiac artery (r = 0.8260).
Stueckle and colleagues confirmed this early experience and reported their results of 52 patients who underwent both CTA and angiography [47]. All aneurysms, occlusions, stenoses, and calcifications were diagnosed correctly by CTA in axial and multiplanar projections (sensitivity 100 %; specificity 100 %). The degree of stenosis was overestimated in three cases when using axial projections. Three-dimensional volume-rendered CTA showed a sensitivity of 91 % for aneurysms, 82 % for stenoses, 75 % for occlusions, and 77 % for calcifications. The specificity was 100 % in all cases.
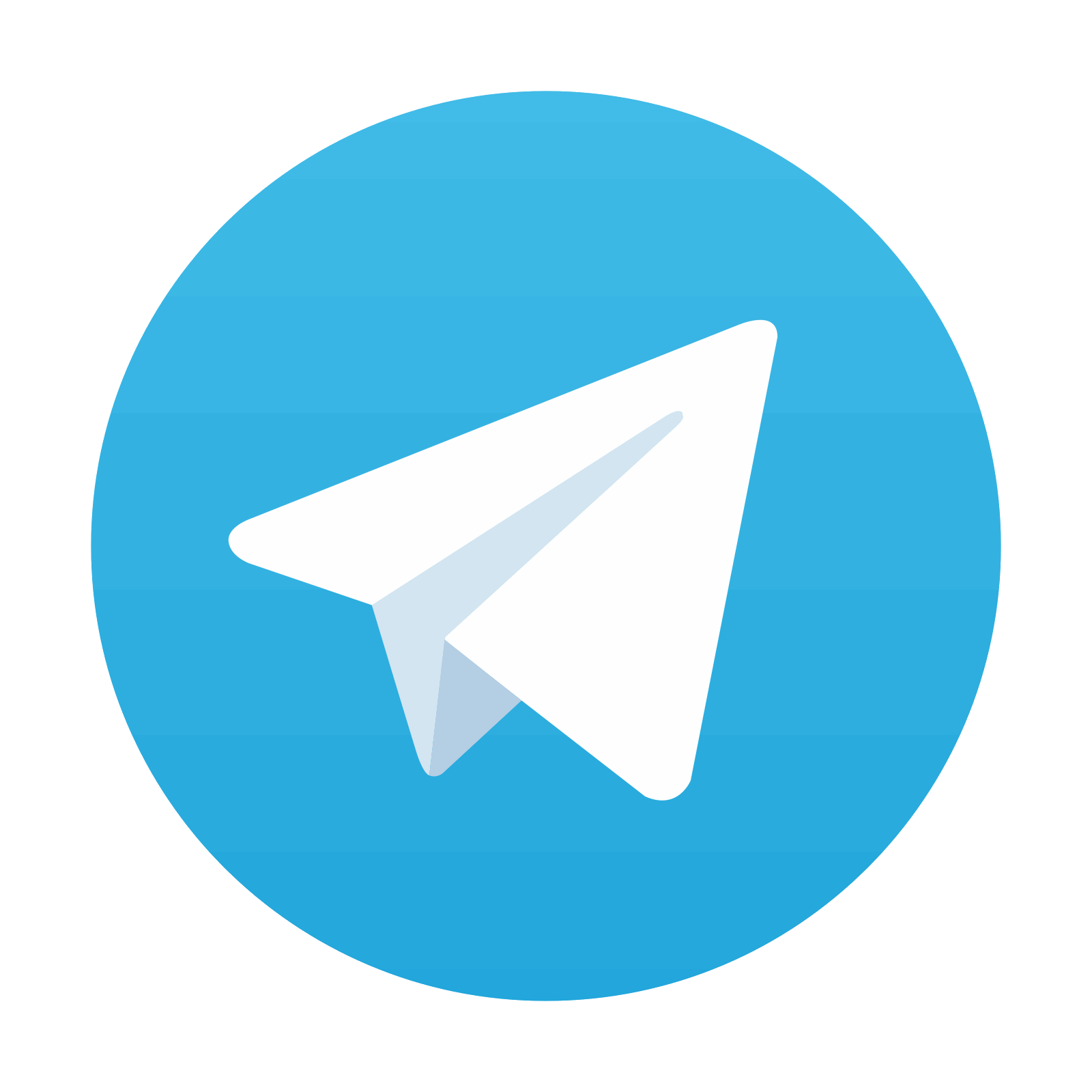
Stay updated, free articles. Join our Telegram channel

Full access? Get Clinical Tree
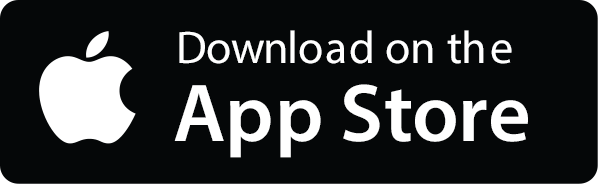
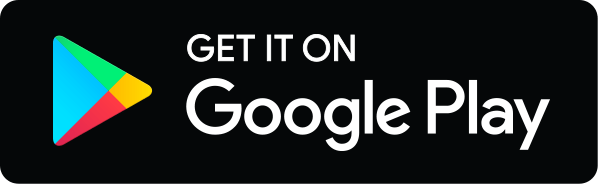