Cardiac PET myocardial perfusion imaging has gained considerable acceptance in the clinical world of Nuclear Cardiology for many reasons. One driving force has been the substantial expansion of PET oncology, which has led to widespread availability of PET scanners in hospital settings. However, the burgeoning literature has also provided a demonstration of the incremental clinical value of cardiac PET, which has resonated in the marketplace and resulted in a gradual but steady growth of this modality into office-based and hospital settings. Finally, the sustained availability of radiotracers in the form of generator-produced 82Rb and cyclotron-produced 13N-ammonia has provided a stable milieu for the growth of cardiac PET myocardial perfusion imaging. This chapter describes the latest documentation of the important aspects of cardiac PET that makes this a compelling story for the present and future of nuclear cardiology. Sections include: brief history of cardiac PET, description of available tracers, diagnostic accuracy, image quality, unique aspects of PET MPI data, including myocardial blood flow (MBF), risk stratification, radiation exposure, protocols, and patients best suited for evaluation and tracers in development.
William Sweet and Frank Wren used positron-emitting radionuclides in the 1950s to localize brain tumors.1,2 This was achieved by using two opposing gamma-ray detectors. Subsequently, in the 1970s, using multidetector systems, Burnham et al3 were able to map out a 2-dimensional distribution of positron-emitting radionuclides. The first PET scanner for use in humans was developed by Michael Phelps and Ed Hoffman in 1976.4 Short-lived radionuclides, such as carbon-11, nitrogen-13, and oxygen-15, however, were used mainly in neurology.
Detectors used in PET scanners changed from Bismuth Germinate (BGO) in 19855 to a new scintillation material called lutetium oxyorthosilicate (LSO). The faster decay time of LSO meant that it could handle higher count rates, which allowed dynamic image acquisition. The first commercial scanner with LSO crystals was introduced in 2001 by Siemens. Initially, attenuation correction (AC) on PET systems was performed using a radioactive source. It was only during the early 21st century that CT was integrated into PET systems that led to more accurate AC, which enabled accurate quantification of tracer distribution and along with LSO crystal technology made quantifying myocardial perfusion in mL/gm/min feasible.
It was not long after thallium-201 was seen as a viable myocardial perfusion tracer for gamma camera imaging in the mid- to late 1970s, that 13N-ammonia was among the first radioactive tracers to be investigated for use in myocardial PET (Figure 1-1). In 1979, Schelbert et al6 demonstrated images of myocardial perfusion in humans, with the quantitation of MBF in normal volunteers at stress and rest in 1989.7 The use of 13N-ammonia for cardiac imaging, however, was only approved by the US Food and Drug Administration (FDA) in 2007.
Around the same time of developments with 13N-ammonia, the use of 82Rb in PET myocardial perfusion imaging was reported by Selwyn et al.8 Soon after this publication, larger clinical studies took place using generator-produced 82Rb chloride9, leading to FDA approval of the first commercial strontium-82/82Rb generator in 1989 and reimbursement within the United States in 1995.10 In 2016, a second commercial generator also received FDA approval. Initially for only perfusion imaging, or flow determination using external detectors11, the advent of current generation PET scanners and application of compartmental models to dynamically acquired 82Rb images has more recently allowed the tracer to be used to quantify MBF.12 The optimization of fluorodeoxyglucose FDG imaging for several nonperfusion applications, such as myocardial viability, infection, and inflammation imaging has added breadth to cardiovascular PET.
Cardiac PET tracers used in clinical practice are produced in a cyclotron or by a generator. Cyclotrons are particle accelerators that can produce positron-emitting isotopes by bombarding a target with rapidly accelerating charged particles. 13N-ammonia (13N NH3), O-15 water (15O-H2O) and fluorine-18 fluorodeoxyglucose (18F–FDG) are cyclotron-produced PET tracers. The former two are perfusion tracers and the latter is used in viability and infection/inflammation imaging. Table 1-1 shows the characteristics of the two most commonly used tracers, 82Rb and 13N-ammonia.
13N ammonia | 82Rb | |
---|---|---|
Half-life (minutes) | 9.96 minutes | 76 seconds |
Imaging duration | 10–20 min | 6–10 min |
Administered activity (3D scanner) | 740 MBq (20 mCi) | 740-1480 MBq (20-40 mCi) |
Production | Cyclotron | Generator |
Typical distances to annihilation event in tissue (FWHM) | 0.26–0.62 mm | 0.56–1.43 mm |
Image resolution | Moderate | Low |
First-pass extraction fraction | 80% | 65% |
Perfusion defect contrast | Moderate | Low |
Pharmacological stress | Routine | Routine |
Treadmill exercise | Possible (static/gated only) | Technically challenging |
82Rb is a potassium analog radiopharmaceutical that is produced with commercially available generators that can be delivered on-site. It is the result of the decay of strontium-82 (82Sr), which has a half-life of 25.5 days. Thus, the generator life in the laboratory is 4–6 weeks depending on the camera system used. Once produced, 82Rb has a half-life of 76 seconds. The extraction fraction of 82Rb is estimated to be 65%-75%13,14, substantially higher than SPECT technetium agents.
The use of 82Rb is provided by an on-site generator and delivery system, which can be stored conveniently in the camera room. The generator is supplied at a fixed cost, independent of the number of studies performed. Because of the cost involved with the generator, consideration for PET use in a facility should include adequate patient volumes, generally considered 3–4 patients a day or more. When in use, the generated rubidium is replenished quickly, within 10 min via 82Sr to 82Rb decay. This permits minimal downtime between stress and rest imaging, allowing efficient utilization of the generator. The protocol for stress imaging requires the use of a pharmacologic stress agent because of the short half-life of 82Rb. Protocols are described in more detail later in this chapter.
Advantages of 82Rb include the ability to have rapid acquisition times for rest and stress imaging and continuous availability. The acquisition time is generally 7 minutes after injection of tracer. There are also disadvantages to 82Rb imaging. Because of the half-life of 82Sr, the same generator is used for a month to 6 weeks. Depending on the density of surrounding tissue the annihilation event occurs typically 0.56–1.43 mm from the emission15, producing lesser quality images although still superior to SPECT. The short half-life of rubidium limits its use to pharmacologic stress and not exercise, and mandates an on-site generator, rather than regional “per dose” distribution. Thus, adequate patient volume is very important for financial feasibility.
13N NH3 is one of the earliest PET tracers that was used for myocardial perfusion imaging. 13N NH3 undergoes several enzymatic metabolic changes to 13N glutamine and is retained in the myocardium and liver. Implementation requires the presence of an on-site or close-by cyclotron because it has a half-life of 9.96 min. 13N has an extraction fraction of approximately 80%.16 Advantages of 13N-ammonia include the high tomographic counts and a clear blood-to-myocardial delineation. In comparison to rubidium, the annihilation event is much closer to the emission (0.26–0.62 mm)15 and, therefore, image quality is considered superior. Because of the longer half-life, exercise is possible, in contrast to rubidium. Limitations include the finding that even in normal subjects, the retention of 13N in the lateral wall is 10% less than other segments creating false-positive results.17 Prominent liver activity in some studies can make interpretation of the inferior wall difficult.18 Finally, the necessity for on-site or close proximity cyclotron availability because of short half-life limits its implementation. This has severely limited its use, with perhaps 15–20 laboratories using this agent in the United States. This may change with the availability of a relatively small cyclotron designed for 13N-ammonia production, which can be placed onsite.
15O-H2O is a perfusion tracer that is not approved by FDA. It is mainly used for research purposes and in clinical practice in some European centers and is only briefly discussed. 15O-H2O is a cyclotron-produced tracer with short half-life of 2.07 minutes. It is a freely diffusible agent with very high (95%-100%) myocardial extraction19 and, hence, a near linear relationship to MBF. It has no myocardial retention because of rapid equilibration with extracellular tissue and is independent of MBF. The linearity to MBF makes it the most physiological radiopharmaceutical and is the gold standard for noninvasively measuring MBF. However, myocardial perfusion imaging is not possible owing to poor myocardial retention as well as retained blood pooling with resultant poor image quality. Despite this limitation, the use of MBF has been used to validate the use of cardiac PET in comparative studies with other modalities, to be discussed later in this chapter.
The diagnostic accuracy of cardiac PET perfusion imaging has been assessed with multiple studies. However, most studies are small and selected, and the same patients not used for comparison between modalities. The “gold standard” has been that of anatomic findings at cardiac catheterization, which has its own issues for judging accuracy. That said, there is a growing literature for cardiac PET, although fewer than for SPECT. Generally results are from 82Rb and line source AC although a few studies also assess 13N-ammonia and PET/CT systems. Most evaluations have used the meta-analysis format to provide the best assessment of diagnostic accuracy for PET and in particular comparison to SPECT.
With regard to independent literature of the diagnostic accuracy for cardiac PET perfusion, several studies report high sensitivity and specificity. Nandalur performed a meta-analysis of more than 1400 patients who underwent PET imaging and reported a sensitivity of 93% and a specificity of 83%.20 Beanlands, several years later, evaluated the literature of PET alone, reporting on 18 studies and found a weighted sensitivity of 90% with a specificity of 89% similar to that of Nandalur.21 In a single center study, Sampson et al22 reported a high sensitivity and specificity for rubidium PET/CT imaging, a newer technique for cardiac PET growing in use and often replacing dedicated PET cameras for AC.
Jaarsma et al23 reported a meta-analysis comparing the diagnostic accuracy of myocardial perfusion imaging using SPECT, PET, and CMR. A total of 166 studies were included in their analysis (114 SPECT, 15 PET, and 37 CMR). However, this review included studies with both SPECT tracers (thallium and technetium) and nonstandard imaging protocols (predominantly nonattenuation corrected studies and no reference to ECG gating, as well as retrospective studies). The PET studies included mostly 82Rb as the tracer (1/15 with Copper-62) and only three were with PET/CT while the rest of the studies used line source for AC. The authors concluded that all three modalities have similar sensitivity. PET had better specificity and overall best diagnostic accuracy among the three modalities. However, this study had limitations, some of which are highlighted earlier. An important limitation was in allowing retrospective studies to be included in the analysis.
A more contemporary comparative meta-analysis of only SPECT and PET technology was undertaken by McArdle et al.24 Inclusion criteria included prospective, observational, and retrospective studies as well as case series using either 82Rb PET, or 99mTc SPECT with both ECG-gating and AC using either CT or radionuclide sources. Coronary angiogram was used as the reference standard for diagnosis of obstructive CAD. Fifteen PET comprising 1344 patients and 8 SPECT studies with 1755 patients, met their inclusion criteria. Using weighted averages proportional to the patient population size, sensitivities for PET and SPECT was 90% (CI 0.88–0.92) and 85% (CI 0.82–0.87), respectively, and specificities of 88% (CI 0.85–0.91) and 85% (CI 0.82–0.87), respectively, and ROC curve was 0.95 and 0.90 for PET and SPECT, respectively (P <0.0001) (Figure 1-2). Despite some limitations caused by referral bias and heterogeneity in the study population, this is an important study that confirmed that PET perfusion imaging is more accurate in detecting CAD despite technological advances in SPECT.
Figure 1-2
Summary receiver operating characteristic curves demonstrating superior diagnostic performance of MPI PET over SPECT. (Reproduced with permission from Mc Ardle BA, Dowsley TF, deKemp RA: Does rubidium-82 PET have superior accuracy to SPECT perfusion imaging for the diagnosis of obstructive coronary disease? A systematic review and meta-analysis, J Am Coll Cardiol. 2012 Oct 30;60(18):1828-37)

Another meta-analysis was reported by Parker et al.25 The authors included only prospective studies and excluded any that explicitly stated that it was retrospective. Additionally, they used a bivariate random effects model, rather than pooled sensitivity and specificity from individual studies. They identified 117 studies, including 108 evaluating SPECT MPI, 4 evaluating PET MPI, and 5 evaluating both modalities. Bivariate meta-analysis demonstrated a significantly higher pooled mean sensitivity with PET [92.6% (95% confidence interval 88.3%-95.5%)] compared with SPECT [88.3% (95% confidence interval 86.4%-90.0%)] (P = 0.035). While the specificity for PET was higher than SPECT, the differences did not reach statistical significance [81.3% (95% confidence interval 66.6%-90.4%)] and SPECT [75.8% (95% confidence interval 72.1%-79.1%)] (P = 0.39). Thus, two major studies reported higher sensitivity for PET and improved overall diagnostic accuracy (Figure 1-3). The more general study of Parker reflecting current SPECT practice reported higher specificity as well although it did not reach statistical significance.
Figure 1-3
Summary receiver operating characteristic curves demonstrating superior diagnostic performance of MPI PET over SPECT. (Reproduced with permission from Parker MW, Iskandar A, Limone B: Diagnostic accuracy of cardiac positron emission tomography versus single photon emission computed tomography for coronary artery disease: a bivariate meta-analysis, Circ Cardiovasc Imaging 2012 Nov;5(6):700-707)

Using 13N-ammonia PET/CT, a prospective study26 was undertaken to assess the added diagnostic value of MPR independent to findings on relative perfusion images for predicting CAD (luminal narrowing ≥50%) using invasive coronary angiography as the reference standard. The study included 73 patients who underwent a standard adenosine protocol. The pretest likelihood of CAD was high in this patient group (73%). Per patient, the sensitivity, specificity, positive predictive value, negative predictive value, and accuracy of MPI for detecting significant CAD were 79%, 80%, 91%, 59%, and 79%, respectively. With a cutoff of less than 2.0 for global MPI to relative perfusion images findings improved the values to 96% (P <0.005), 80%, 93%, 89% (P <0.005), and 92% (P <0.005), respectively. The authors demonstrated that quantification of global MPR with 13N-ammonia provides a substantial added diagnostic value, particularly improvement in sensitivity for detection of CAD, in a selective group of patients.
The diagnostic accuracy of cardiac PET has not been systematically evaluated in subgroups of patients, such as diabetic patients, women, and body habitus. However, a recent article by Taqueti and Dorbala27 strongly endorsed the use of cardiac PET in women for diagnostic accuracy based on the two meta-analysis studies as well as reduction of false-positives, excellent image quality and low radiation exposure, particularly important in these patients. In addition, a meta-analysis by Iskander et al28 as a subevaluation of the study by Parker et al25 found no difference in SPECT diagnostic accuracy between genders. Thus, it may be inferred that the improved accuracy reported by both Parker et al and McArdle et al applies to women.24,25
Many consider PET useful in obese patients only. In a comparison of PET and SPECT patients with similar risk profiles undergoing clinically indicated coronary angiography, Bateman et al did address gender and body weight accuracies between these two tests.29 With 70% stenosis in one or more major vessels at catheterization, these authors reported significantly greater diagnostic accuracy for cardiac PET than SPECT regardless of gender (male and female) and body habitus (BMI >30 or <30). These findings are illustrated in Figure 1-4. Another important comparison in this study was the ability to correctly identify multivessel CAD at catheterization.29 Bateman et al found that PET was significantly better at identifying two or greater vessel CAD than SPECT (71% vs 48%, P <0.001). An example of this is shown in Figure 1-5 of a patient who underwent a rest/stress SPECT and rest/stress PET study on the same day prior to catheterization. In Panel A is the rest/stress SPECT study demonstrating a reversible infero/lateral perfusion abnormality suggesting single vessel disease, while in Panel B the PET study demonstrated TID and reversible defects in the inferior, anterior, and lateral distributions, a multivessel pattern. At catheterization the patient had >70% stenosis in all three major vascular territories.
Figure 1-4
Comparison of sensitivities for PET (red) and SPECT (blue) in the subgroup analysis of Bateman et al32 for BMI (A) and gender (B) demonstrating superior performance of PET MPI over SPECT in both subgroups.

Figure 1-5
Patient who underwent a rest/stress SPECT and rest/stress PET study on the same day prior to catheterization; the (A) rest/stress SPECT study demonstrating a reversible infero/lateral perfusion abnormality suggesting single vessel disease, (B) PET study demonstrating TID and reversible defects in the inferior, anterior, and lateral distributions, a multivessel pattern. At catheterization the patient had >70% stenosis in all three major vascular territories.

Diagnostic accuracy with cardiac PET can also be improved using MBF data, particularly in minimizing CAD likelihood. In a small study reported by Nava et al30, the presence of normal MBF reserve (in this study >1.93), the negative predictive value was 97%.
In summary, the diagnostic accuracy of cardiac PET perfusion imaging with both tracers of 82Rb and 13N-ammonia is high for both sensitivity and specificity. In two large meta-analyses comparing PET with SPECT, diagnostic accuracy was higher with cardiac PET. Data supports that this applies to both male and female patients, is body habitus-independent, and that PET is significantly better at identifying multivessel disease. These findings have implications for patient selection, to be discussed in a later section of this chapter.
Myocardial PET perfusion imaging utilizes radionuclides with higher energy (511 keV), but with very short half-lives. This necessitates higher administered activity to patients but with overall lower radiation exposure owing to very short half-lives and better acquisition characteristics. PET imaging procedures result in high myocardial counts, higher spatial and contrast resolution, and high signal-to-noise ratio. Thus one would anticipate superb image quality. This was demonstrated by Yoshinaga et al31 in which patients with equivocal SPECT studies were referred for 82Rb cardiac PET imaging. In a very high percentage of patients, the PET study resulted in good to excellent image quality compared to the equivocal findings of SPECT. Using a comparison of similar but matched patients undergoing SPECT or PET, Bateman et al32 also reported a significant improvement in image quality with PET over SPECT, with fewer equivocal reports.
The routine use of AC plus higher energy levels of PET tracers substantially reduces attenuation artifacts in images, in turn reducing the potential for false-positive studies for interpretation. This may be particularly useful in obese patients. It should be noted that obesity should not be a prerequisite for a cardiac PET examination. In a study by Bateman et al32 the specificity of PET was significantly higher in comparison to SPECT, regardless of body habitus, including obese patients; see Figure 1-4. Similarly, in this study, patients who had equivocal SPECT studies were found to have diagnostically useful PET studies in 98% of the referred patients; this is exemplified in Figure 1-6 where an equivocal area of reduced perfusion on the 99mTc-SPECT/CT study is much more apparent on the 82Rb PET/CT study. Additionally, the incidence of gut activity affecting interpretation was significantly and substantially reduced with PET in relation to pharmacologic SPECT. An example of image quality between SPECT and PET in the same patient is shown in Figure 1-7. As noted, considerably less gut and liver activity is observed in the PET study as well as uniformity in counts in the myocardium.
These results were also confirmed by Flotats et al.33 In this study the imaging results were compared in 27 patients who underwent both rest/stress SPECT and PET. In a blinded read format, the image quality, interpretive certainty, and catherization results were all statistically significantly better with PET than SPECT.
In conclusion, image quality of cardiac PET studies in comparison to SPECT is significantly better for both rest and stress imaging. While this holds true for obese patients, it is not confined to those alone, particularly with regard to diagnostic confidence and, therefore, consideration should be given to the use of cardiac PET in any patient who is a candidate for either study.
The acquisition of data with cardiac PET perfusion imaging is performed shortly after injection of tracer, particularly with 82Rb, owing to short half-lives. For example, as described in the protocol section, acquisition of 82Rb perfusion data is 90–120 seconds after tracer injection in contrast to SPECT acquisition, which occurs 45–60 minutes later. The implication of this is that data acquisition is accomplished under the hyperemic phase of both dipyridamole, and regadenoson infusion and shortly thereafter adenosine. This has implications for such information as transient ischemic cavity dilation (TID) and stress-induced wall motion abnormalities and changes in ejection fraction (EF).
Transient ischemic cavity dilation (TID) with PET differs from SPECT in that the acquisition of data is still in a hyperemic phase of pharmacologic influence, particularly with the tracer 82Rb. The protocol for 82Rb begins collection of perfusion data 90–120 seconds after tracer injection. Thus, there could be true stress-induced cavity dilation, which is separate from subendocadial ischemia. Observational data have identified the presence of TID with both 82Rb and 13N-ammonia to be relatively common, perhaps more so than with SPECT. The presence of TID with PET is strongly associated with CAD, very uncommon without disease, and associated with both single vessel and multivessel conditions. As with SPECT, PET TID can be both visual and quantitative. Two studies have established that a TID ratio of 1.13–1.15 +/- 2 SD to be upper limits of normal.34,35 In these studies, the presence of TID was associated with more extensive perfusion abnormalities and left ventricular dysfunction35; however, it was noted to be found in patients with single vessel ischemia as well as single vessel CAD at catherization.34 Both studies concluded that TID was specific, but not necessarily sensitive. The differences between SPECT and PET TID need to be elucidated in future studies. Thus, the clinical value of TID is in supporting the diagnosis of CAD and may be an indication of more severe disease.
As with SPECT, left ventricular functional evaluation in the form of wall motion and overall EF has clinical value. It is generally accepted that in normal subjects, the PET EF rises about 5% during stress in relation to rest. Traditional examination of stress EF with cardiac PET has been shown to have prognostic value in more than 1600 patients and is complementary to perfusion data.36 As shown in Figure 1-8, from that study, patients with the worse outcomes had markedly abnormal perfusion and function. Stress-induced wall motion abnormities as well as a fall in EF during stress are occasionally noted in SPECT but is more commonly observed with cardiac PET studies. The frequency of this finding in PET, as well as previously noted with TID most likely results from the rapid acquisition of data following tracer injection, thus in the hyperemic phase of the pharmaceutical drug. One of the first studies to note a relationship between change in EF between rest/stress cardiac PET and perfusion abnormalities was reported by Brown et al.37 They reported an inverse relationship between negative change in LVEF between rest and stress and the presence and severity of PET perfusion abnormalities as well as reversibility. Dorbala and colleagues developed the concept of EF reserve, and drew an association between EF reserve <0 and severe reversibility and multivessel disease, while a less dramatic fall in EF was correlated with mild-moderate reversibility in more than 900 patients.38 In a subsequent study, the same group examined the prognostic value of EF reserve.39 In the latter study, they reported that an EF reserve of <0 (stress EF lower than rest) was associated with a significantly higher risk of all-cause mortality and cardiac death (Figure 1-9).
Figure 1-8
Incremental prognostic value of functional data derived from rubium-82 MPI studies. Patients with the worse outcomes had markedly abnormal perfusion and function. (Modified with permission from Lertsburapa K, Ahlberg AW, Bateman TM, et al: Independent and incremental prognostic value of left ventricular ejection fraction determined by stress gated rubidium 82 PET imaging in patients with known or suspected coronary artery disease, J Nucl Cardiol 2008 Nov-Dec;15(6):745-53)

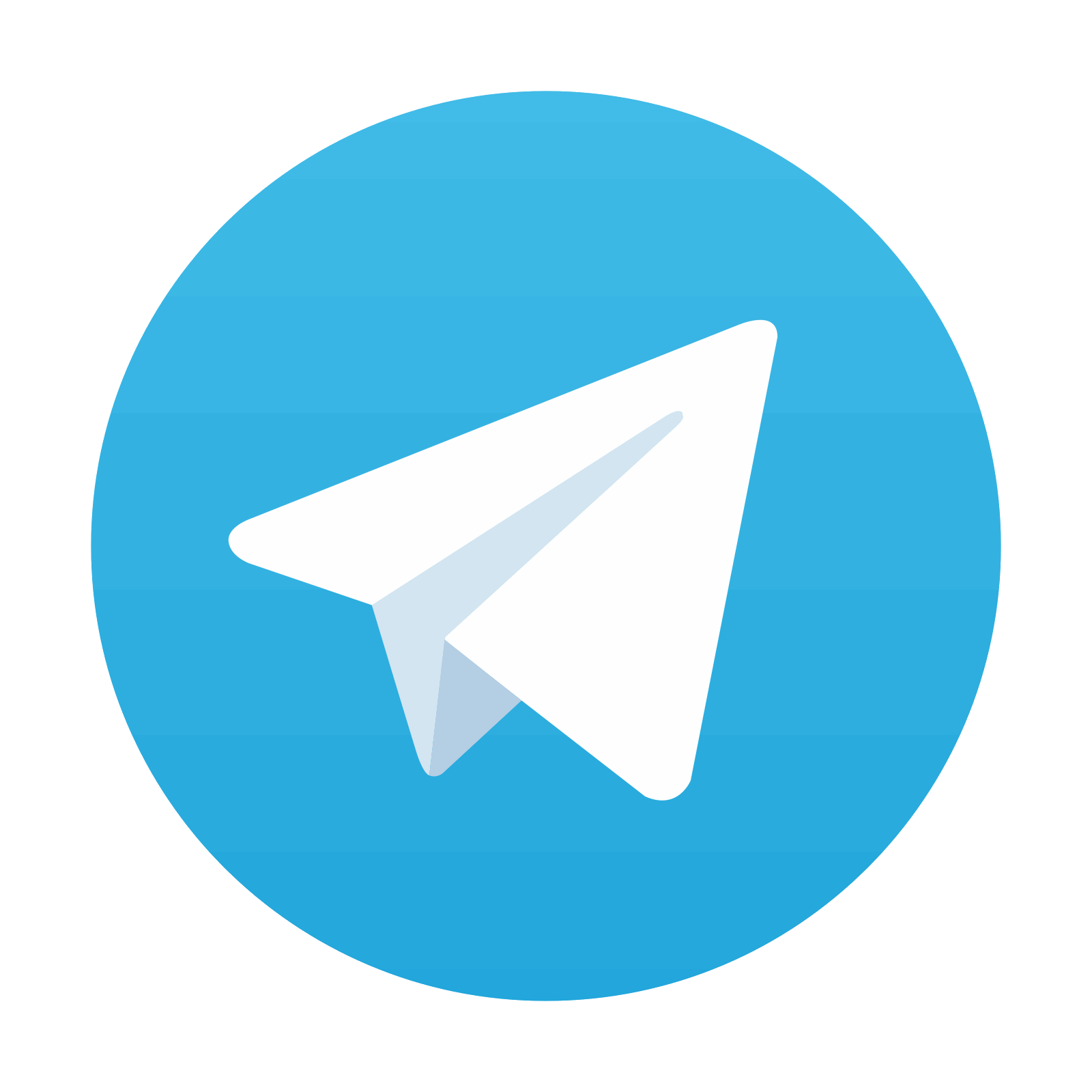
Stay updated, free articles. Join our Telegram channel

Full access? Get Clinical Tree
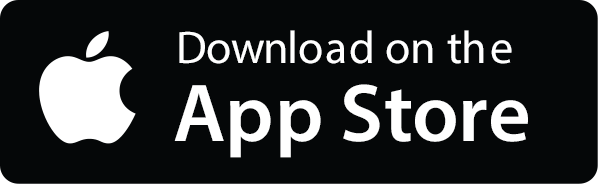
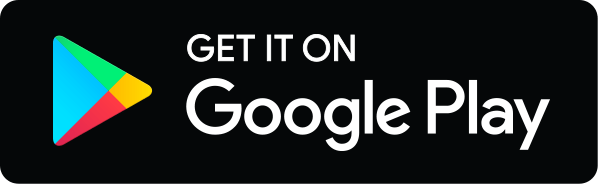
