Background
Automatic quantification of real-time three-dimensional (3D) full-volume color Doppler transthoracic echocardiography (FVCD) has been proposed as a feasible and accurate method for quantifying MR. We aimed to explore the clinical implications of real-time 3D-FVCD for mitral regurgitation (MR) with various clinical manifestations, in comparison with the conventional two-dimensional (2D) proximal isovelocity surface area (PISA) and volumetric method and cardiac magnetic resonance imaging (CMR) methods.
Methods
A total 186 patients with MR were enrolled prospectively. Based on exclusion criteria and image quality review, 152 patients were included in the final analysis for 3D-FVCD and 2D transthoracic echocardiography. Among them, 37 patients underwent subsequent CMR for the validation of 3D-FVCD.
Results
MR volume from 3D-FVCD demonstrated a better agreement ( r = 0.94) with CMR than 2D-PISA or the 2D volumetric method (VM; r = 0.87 vs 0.56). Overall, 2D methods underestimated MR when compared with 3D-FVCD (35.4 ± 28.4 mL for 2D-VM vs 43.8 ± 24.6 mL for 2D-PISA vs 64.6 ± 35.1 mL for 3D-FVCD; P < .001). In subgroup analysis, multijet MR (odds ratio [OR], 6.30; 95% CI, 2.52–15.72) and dilated left ventricular end-systolic diameter ≥40 mm (OR, 2.90; 95% CI, 1.12–7.50) were predictors of significant difference in MR volume (>30 mL for primary MR and >15 mL for secondary MR) between 2D-PISA and 3D-FVCD. In identifying surgical candidates, patients with multijet MR (OR, 4.53, 95% CI, 1.99–10.35) demonstrated a higher risk of discrepancy between 2D-PISA and 3D-FVCD, which were consistent in both primary and secondary MR, respectively.
Conclusions
MR quantification with 3D-FVCD showed better correlation and agreement than conventional 2D methods. MR was underestimated by 2D methods, especially in multijet and dilated left ventricle. Multijet MR demonstrated higher risk of discrepancy for the identification of surgical candidate, regardless of MR etiology.
Mitral regurgitation (MR) is one of the most prevalent valvular heart diseases. Of greatest importance is the fact that the survival tends to decline in patients with significant MR irrespective of its etiology. Quantitative assessment of MR severity is imperative because of its link to prognosis, and further still, surgical management is indicated in those who present with severe MR.
There are several methods and parameters for assessing MR by two-dimensional (2D) transthoracic echocardiography (TTE). Proximal isovelocity surface area (PISA) by 2D-TTE is well validated and recommended for quantifying MR and is widely utilized in clinical practice. The volumetric method (VM) and pulse-wave Doppler flow (PWDF) method use the difference of stroke volume (SV) from left ventricular (LV) and aortic forward flow volume. Despite its apparent utility, there are some potential pitfalls. For the PISA method, a multistep process with multiple assumptions may introduce significant error toward MR severity. VM is also known for the potential error on MR quantification due to its assumption on left ventricular outflow tract (LVOT) as a circular structure.
The recent development and implementation of real-time three-dimensional (3D) full-volume color Doppler echocardiography (FVCD) has made it possible to quantify mitral inflow and aortic outflow SVs, in parallel with an automated quantification algorithm. Notably, in prior validation studies, the automated quantification of MR by 3D-FVCD was feasible and accurate. However, the significance of quantifying MR by 3D-FVCD in a clinical setting with various characteristics is yet to be established.
Therefore, we aimed to explore the differential impact of MR quantification by real-time 3D-FVCD in a wide spectrum of MR phenotypes, while comparing it with the conventional 2D and cardiac magnetic resonance imaging (CMR) methods.
Methods
Study Population and Protocols
Initially, this study comprised 186 consecutive patients presenting with mild-to-severe native valve MR who were referred to Severance Hospital for TTE examination between December 2011 and March 2015. The severity of MR was defined by comprehensive assessment with LV remodeling, effective regurgitant orifice area (EROA), regurgitant volume by the VM and PISA methods, vena contracta width (VCW), jet characteristics, and the presence of systolic flow reversal in pulmonary veins according to the guidelines. Patients with more than a mild degree of aortic regurgitation ( n = 22), abnormal intracardiac shunt ( n = 2), or significant arrhythmia including ventricular arrhythmia and poorly controlled atrial fibrillation (AF; heart rate > 100 beats/min and/or beat-to-beat variability > 30 beats/min, n = 6) were excluded. Hence, subsequent 3D-FVCD study was performed on the remaining 156 patients. After reviewing the image quality, four patients were further excluded due to poor image quality. A flow diagram of study population is summarized in Supplemental Figure 1 .
The cause of MR was classified as primary or secondary. Primary MR was defined as MR related to intrinsic valvular abnormalities, and secondary MR was defined as leaflet tethering or incomplete coaptation of leaflets in the presence of regional or global LV remodeling without intrinsic valvular abnormalities. MR was further classified by the jet direction (central vs eccentric) and by the number of jets (single vs multiple). The cardiac rhythm was classified as sinus rhythm or AF by electrocardiography. Candidates for mitral valve (MV) surgery were classified according to current guidelines based on the symptom, ejection fraction, chamber dimension, and MR severity. The criteria for severe MR was EROA ≥ 40 mm 2 and MR volume ≥ 60 mL for primary MR and EROA ≥ 20 mm 2 and MR volume ≥ 30 mL for secondary MR. Informed consent was obtained from all participants, and the study protocol was approved by the ethics committee at Severance Hospital.
Image Acquisition and MR Quantification
Each of the image acquisitions was obtained during the same day for all patients. In the event of AF, MR volume was quantified when the preceding and pre-preceding R-R intervals differed by less than 10%.
Two-Dimensional Echocardiography Image Acquisition and the MR Quantification
Two-dimensional TTE was performed using an ACUSON SC2000 ultrasound system (Siemens Medical Solutions USA Inc., Mountain View, CA) with a 4V1c phased-array transducer at a frequency of 4.5 MHz. Measurements for MR assessment was done according to American Society of Echocardiography guidelines.
The VCW of the MR jet was measured at the narrowest portion of the regurgitant jet in zoom mode with an adapted Nyquist limit (59.3 cm/sec) and averaged from the measurements in the four-chamber and three-chamber views.
Two volumetric methods were used: (1) the PWDF method used quantitative Doppler using mitral and aortic SVs and (2) the VM was calculated from the difference of SV, which measured from LV end-diastolic and end-systolic volume and aortic forward flow volume. The PWDF method used the difference between MV inflow volume and LV SV, with measurements of multiple parameters. To obtain the Doppler spectral profiles of mitral annulus (MA) and LVOT, a pulsed-wave Doppler sample was carefully placed as parallel as possible (angle < 20°) to the blood flow in apical four-chamber and three-chamber views. The MA diameter was measured between the inner edges of the base of posterior and anterior leaflets in early to mid-diastole at maximal MV opening, in the apical four-chamber view; and the LVOT diameter was measured just below the aortic valve in early to midsystole, in the parasternal long-axis view. Both diameters were measured at levels at which the volume samples were placed. To obtain the velocity-time integral (VTI), the modal velocity profile on Doppler recordings was traced. Regurgitant VTI was averaged from the measurements in apical four-chamber and three-chamber views.
The flow convergence of MR was measured where the hemispheric morphology of flow convergence can be optimized, allowing for continuous Doppler signaling to be well aligned with the regurgitant jet. Doppler aliasing velocity was shifted in order to optimize the appearance of PISA (e.g., from 31.0 to 42.0 cm/sec; mean, 36.2 ± 7.1 cm/sec). We reviewed multiple apical planes and phasic changes to find the representative PISA. The selected cine loop was reviewed stepwise, and a clearly defined mid- to late-systolic maximal flow convergence zone was measured from the zenith to the regurgitant orifice. In multijet MR, major PISA was chosen for the measurement. The values were used to calculate the MR volume and EROA using the following formulas:
EROA = 2 Π r 2 : × V a PkV Regurg
and
MR volume = EROA × VTI MR ,

Real Time 3D-FVCD Image Acquisition
Using the ACUSON SC2000 ultrasound system with a 4Z1c real-time volume imaging transducer (2.8 MHz), three consecutive beats of real-time FVCD images with a clear electrocardiographic signal were acquired. The imaging plane was adjusted to permit distinct visualization of the LVOT and MA in a single volume within the apical long-axis view settings. Depth and color Doppler region of interest were optimized to maximize the color volume rates while ensuring that complete MV inflow and LVOT were captured without omission of any mitral or aortic annular color flow. Color Doppler frequency was adjusted at 2.0 MHz, and the color Doppler velocity scale was adjusted to minimize aliasing, unless significant aliasing was not detected in 2D-TTE acquisition. During acquisition, two 2D reference planes focusing on mitral and aortic valve were displayed at the left side of the screen to ensure that the region of interest was contained within the volume.
Automated Quantification of Mitral Regurgitant Volume
Acquired Digital Imaging and Communications in Medicine data were analyzed using validated offline software (Siemens Medical Solutions USA Inc.). The following steps were performed using the prototype software for automated quantification of mitral regurgitant volume: (1) anatomy detection of the LV endocardial border, MA, and the LVOT was automated; (2) the algorithm tracked the 3D motion of the detected LV wall, MA, and the LVOT; (3) the software placed 3D hemispheric flow sampling planes in MA and LVOT—the locations of these planes were dynamically adjusted throughout the cardiac cycles ( Figure 2 A); and (4) the software detected the flow velocities at each voxel in the sampling plane and computed the flow volume by aggregating all sample color flow values. A flow-time curve was derived from flow volume aggregated at each frame in the cardiac cycle, and a calculation of the mitral and aortic SV was performed by temporal integration of this curve ( Figure 2 B). (5) Based on the tracked LV endocardial boundaries, an automated dealiasing algorithm was applied as described elsewhere. This dealiasing factor was then automatically applied to each frame if aliased flow was detected by the algorithm; (6) the dealiased flows of three consecutive cardiac cycles were displayed as a flow-time curve, and additional optional operator-defined dealiasing adjustments could have been performed if automated dealiasing was incomplete, as expressed as a notch in the flow-time curve ( Figure 2 B); and (7) the software computed the flow volumes as the area under the curve of both the MA and LVOT flow in three cardiac cycles, and MR volume was calculated by subtracting LVOT SV from MA SV. A representative case comparing the quantitative analysis of MR by different methods is presented in Figure 3 .


CMRI
CMR data acquisition was performed with a 1.5-T MR scanner (Philips), using a 12-channel phased-array surface coil. The volume of the LV was determined by planimetry from a series of short-axis acquisitions covering the LV completely from the apex to the base. Quantifications of LV end-systolic volume, end-diastolic volumes, and ejection fraction were performed on the short-axis series with manual contour segmentation of the endocardial borders. The total SV was obtained by subtracting the LV end-systolic volume from the LV end-diastolic volume (typical echo time, 1.5 msec; repetition time, 3.1 msec; slice thickness, 10 mm; 25 phase). Aortic forward flow volume was obtained from 2 to 3 cm above the aortic valve in an orthogonal orientation to the aortic root (typical echo time, 2.8 msec; repetition time, 4.7 msec; slice thickness, 8 mm; 50 frames/cardiac cycle; aliasing velocity, 200 cm/sec; number of excitations, 2). MR volume was calculated by subtracting the aortic forward flow volume from the total LV and SV.
Reproducibility Analysis
To determine intra- and interobserver reproducibility, 2D- and 3D-FVCD image analyses were repeated by a second observer in 20 randomly selected patients at a different time point, as well as by the primary reader at least 1 month later. During all analyses, the investigators were blinded to the results of previous measurements. We acquired at least two clips of 3D images. To evaluate interobserver variability, each observer reviewed and selected a 3D image and subsequently assessed its MR volume.
Statistical Methods
Continuous variables are presented as means ± SD. Categorical variables are reported as absolute counts or percentages. The MR volume quantified by each method was compared by use of a paired t -test. One-way analysis of variance was used for continuous variables among groups. Linear regression analysis was performed to evaluate the linear relationship between MR volumes from each method. A Bland-Altman plot was generated to evaluate the agreement between methods. The agreement of MR volume between methods was compared using the intraclass correlation coefficient (ICC). Logistic regression models were used to identify predictors for the significant difference in MR volume (>30 mL) between the 2D- and 3D-FVCD methods. Factors resulting in the discrepant assessment of surgical candidates were also assessed using logistic regression models. P < .05 was considered statistically significant. All analyses were performed using PAWS statistic version 18.0 (SPSS Inc., Chicago, IL) and MedCalc version 12.3 (MedCalc Software, Mariakerke, Belgium).
Results
Baseline Characteristics
Baseline characteristics of the patients and MR phenotypes are presented in Table 1 . The mean age was 57.4 ± 14.8 years, and 78 patients were male. Mean LV end-diastolic diameter (LVEDD) and end-systolic diameter (LVESD) were 59.5 ± 9.0 and 42.8 ± 11.6 mm, respectively. Mean left atrial volume index (LAVI) was 71.1 ± 47.7 mL/m 2 , and AF was present in 46 patients. The cause of MR was primary in 101 patients and secondary in the remaining 51 patients. Fifty-one patients had eccentric MR jet. Thirty-two patients showed multijet MR. The severity of MR was categorized as mild in 39 patients, moderate in 60 patients, and severe in 53 patients.
Variables | Overall patients ( N = 152) | Validation group ( n = 37) |
---|---|---|
Age (years) | 57.4 ± 14.8 | 57.5 ± 12.9 |
Male gender | 78 (51.3%) | 20 (54.1%) |
Cause of MR: primary/secondary | 101/51 (66.4%/33.6%) | 27/10 (73.0%/27.0%) |
Jet direction: central/eccentric | 101/51 (66.4%/33.6%) | 11/24 (31.4%/68.6%) |
Number of jets: single/multiple | 120/32 (78.9%/21.1%) | 27/10 (73.0%/27.0%) |
Rhythm: sinus/AF | 106/46 (69.7%/30.3%) | 24/13 (64.9%/35.1%) |
Systolic BP (mmHg) | 118.7 ± 20.8 | 115.8 ± 15.3 |
Diastolic BP (mmHg) | 76.7 ± 14.2 | 74.3 ± 10.4 |
Heart rate (beats/min) | 76.7 ± 18.6 | 76.4 ± 15.6 |
Severity of MR by 2D | ||
Mild | 25 (16.4%) | 2 (5.4%) |
Moderate | 60 (39.5%) | 19 (51.4%) |
Severe | 67 (44.1%) | 16 (43.2%) |
Echocardiographic parameter | ||
LVEDD (mm) | 59.5 ± 9.0 | 60.7 ± 9.0 |
LVESD (mm) | 42.8 ± 11.6 | 43.7 ± 12.1 |
LVEDV (mL) | 162.0 ± 63.5 | 173.3 ± 61.2 |
LVESV (mL) | 74.9 ± 54.3 | 82.1 ± 52.0 |
LVEDD/BSA | 36.0 ± 6.3 | 36.9 ± 6.2 |
LVESD/BSA | 25.9 ± 7.2 | 26.5 ± 7.4 |
LVEDV/BSA | 97.0 ± 34.9 | 104.2 ± 34.8 |
LVESV/BSA | 44.7 ± 30.2 | 49.2 ± 30.3 |
LV EF (%) | 55.3 ± 19.0 | 55.7 ± 20.5 |
LA volume index (mL/m 2 ) | 71.1 ± 47.7 | 75.0 ± 51.6 |
E/é | 17.2 ± 8.6 | 17.4 ± 6.1 |
VCW (mm) | 5.2 ± 1.4 | 5.4 ± 1.0 |
EROA (cm 2 ) | 0.35 ± 0.25 | 0.38 ± 0.23 |
Comparison of 2D- and 3D-FVCD Methods with CMR as a Reference
The comparison of the 2D echocardiography and 3D-FVCD methods was performed in the validation group ( n = 37), who underwent concurrent CMR within 24 hours of TTE. The baseline characteristics of the validation group are summarized in Table 1 . Using 2D echocardiography, the two volumetric methods of VM and PWDF, along with PISA method, were assessed. MR volume was 44.7 ± 23.8 mL by 2D-PISA, 42.5 ± 29.8 mL by 2D-VM, 110.8 ± 58.1 mL by 2D-PWDF, 68.2 ± 33.8 mL by 3D-FVCD, and 59.8 ± 31.1 mL by CMR. The difference between MR volumes measured by echo-based methods and CMR is summarized in Table 2 and Supplemental Figure 2 . While 2D-VM underestimated MR volume, 2D-PWDF overestimated MR volume. The difference between MR volumes measured by these 2D volume-based methods is further exhibited in Supplemental Figure 3 according to MR etiology, which describes the differences in MR volumes measured by CMR and other methods that use either 2D or 3D echocardiography. However, no significant difference was found between the MR volumes of primary ( n = 27) and secondary MR ( n = 10). Three-dimensional assessment of MR volume demonstrated the better correlation and agreement with CMR ( r = 0.94, P < .001, 1.96 SD, 22.7 mL) compared with 2D-based methods ( r = 0.53, P = .001, 1.96 SD, 57.8 mL for 2D-VM; r = 0.56, P < .001, 1.96 SD, 94.2 mL for 2D-PWDF; and r = 0.81, P < .001, 2 SD, 35.9 mL for PISA method, respectively; Figure 4 ).
MR volume (mL) | Difference of MR volume (mL) between CMR and echocardiography-based methods | |
---|---|---|
2D-PWDF | 110.8 ± 58.1 | −51.0 ± 48.1 |
2D-VM | 42.5 ± 29.8 | 17.4 ± 29.4 |
2D-PISA | 44.7 ± 23.8 | 15.2 ± 18.3 |
3D-FVCD | 68.2 ± 33.8 | −8.7 ± 11.6 |
CMR | 59.8 ± 31.1 |

Comparison of MR Quantification by 2D- and 3D-FVCD Methods
MR assessment with 3D-FVCD was done in 152 patients with suitable images for analysis (97.4%). The volume rate of 3D-FVCD ranged between 15 to 31 volumes/sec, and the mean volume rate was 21.3 ± 5.2 volumes/sec. The mean time required for the 3D-FCVD image acquisitions was 4.3 ± 2.2 minutes (range, 2.7–7.1 minutes).
In overall patients, MR volumes were 43.8 ± 24.6, 35.4 ± 28.4, and 64.6 ± 35.1 mL from 2D-PISA, 2D-VM, and 3D-FVCD, respectively. Compared with MR volume by 3D-FVCD, 2D-PISA showed good correlation ( r = 0.87, P < .001), while 2D-VM demonstrated poor correlation ( r = 0.44, P < .001). This trend was similar in the multijet subgroup ( r = 0.56, P = .001 for 2D-VM vs 3D-FVCD).
Compared with 3D-FVCD, MR volume was underestimated by both 2D-based methods ( P < .001) as shown in the CMR group. The mean difference in MR volume by 3D-FVCD was 20.7 mL for 2D-PISA and 29.2 mL for 2D-VM. Both 2D methods demonstrated the increased difference of MR volume as MR severity progressed. Consequently, severe MR demonstrated a more prominent intermethod difference compared with mild MR (8.7 ± 8.5 in mild vs 24.3 ± 19.9 mL in severe MR, P = .001, for 2D-PISA; 2.1 ± 24.4 in mild vs 37.9 ± 34.9 mL in severe MR, P < .001, for 2D-VM).
Subgroup Analysis according to Characteristics of MR
Subgroup analysis was stratified by MR characteristics, rhythm, and LV dimension. As 2D-VM showed poor correlation with CMR and 3D-FVCD in previous analyses, we used 2D-PISA for comparison with 3D-FVCD. The ellipticity of PISA was bigger in secondary MR than in primary MR (e.g., 1.8 ± 0.4 vs 1.5 ± 0.4; P = .001). Table 3 reports the difference and agreement of MR volume between 2D-PISA and 3D-FVCD. Most groups showed good agreement in MR volume between the two methods when classified according to cause of MR (primary vs secondary MR, ICC = 0.81 vs 0.74), jet direction (central vs eccentric, 0.83 vs 0.72), rhythm (sinus vs AF, 0.81 vs 0.82), and LVEDD (<40 mm vs ≥40 mm, 0.84 vs 0.79). However, 2D-PISA underestimated MR volume compared with 3D-FVCD regardless of MR characteristics in all subgroups. The multijet group ( n = 32) displayed significant intermethod differences in MR volume (16.3 ± 14.7 vs 37.2 ± 20.6 mL; P < .001), while subgroups stratified by other characteristics did not show significant intermethod differences. Multijet MR also demonstrated weaker agreement compared with single-jet MR (ICC = 0.79 vs 0.88, respectively). This poor agreement in multijet MR was also found between the 2D-VM and 3D-FVCD (ICC = 0.55; 95% CI, 0.25–0.75). A detailed etiology of multijet MR ( n = 32) is shown in Supplemental Table 1 .
N = 152 | RV (mL) | Between 3D-FVCD and 2D-PISA | ||||
---|---|---|---|---|---|---|
2D-PISA | 3D-FVCD | ICC (95% CI) | RV difference (mL) | P value | ||
Cause of MR | ||||||
Primary | 101 | 50.2 ± 25.0 | 71.6 ± 36.0 | 0.81 (0.74–0.85) | 21.4 ± 18.7 | .500 |
Secondary | 51 | 31.2 ± 18.1 | 50.5 ± 28.7 | 0.74 (0.59–0.84) | 19.3 ± 17.1 | |
Jet direction | ||||||
Central | 101 | 38.9 ± 25.3 | 60.3 ± 37.5 | 0.83 (0.76–0.88) | 21.4 ± 18.3 | .534 |
Eccentric | 51 | 53.5 ± 20.0 | 73.0 ± 28.2 | 0.72 (0.56–0.83) | 19.4 ± 18.0 | |
Number of jets | ||||||
Single | 120 | 45.4 ± 24.9 | 61.7 ± 33.4 | 0.87 (0.82–0.91) | 16.3 ± 14.7 | <.001 |
Multiple | 32 | 38.0 ± 22.9 | 75.2 ± 39.5 | 0.79 (0.62–0.89) | 37.2 ± 20.6 | |
Rhythm | ||||||
Sinus | 106 | 43.3 ± 24.9 | 63.5 ± 35.1 | 0.81 (0.74–0.87) | 20.1 ± 18.4 | .556 |
AF | 46 | 45.0 ± 24.1 | 67.0 ± 35.4 | 0.82 (0.70–0.90) | 22.0 ± 17.8 | |
LVESD | ||||||
<40 mm | 75 | 45.8 ± 25.4 | 64.1 ± 33.8 | 0.84 (0.77–0.90) | 18.4 ± 16.4 | .114 |
≥40 mm | 77 | 41.9 ± 23.8 | 65.0 ± 36.5 | 0.79 (0.70–0.86) | 23.0 ± 19.6 |
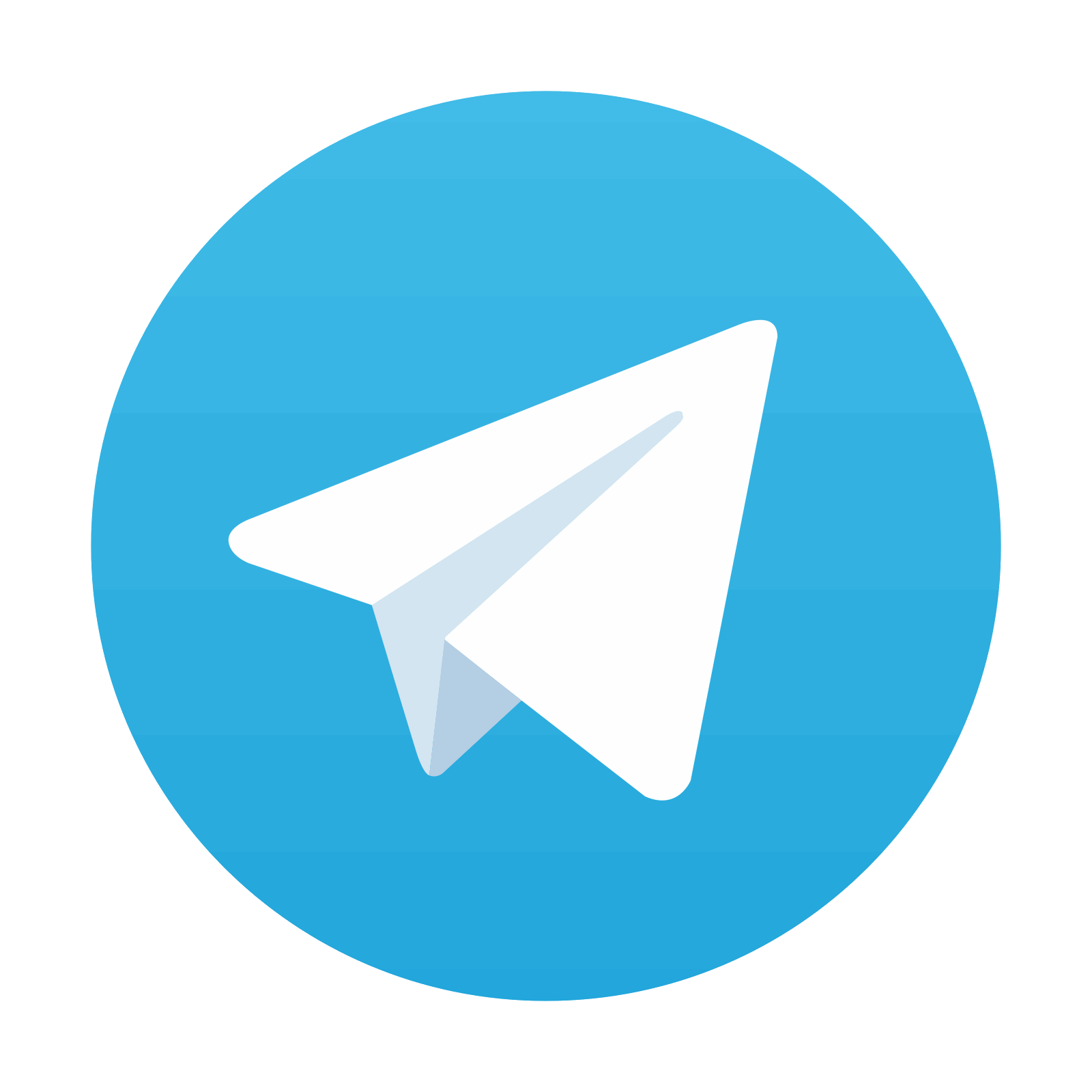
Stay updated, free articles. Join our Telegram channel

Full access? Get Clinical Tree
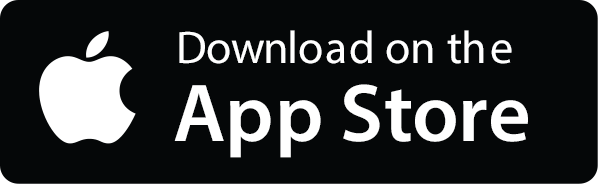
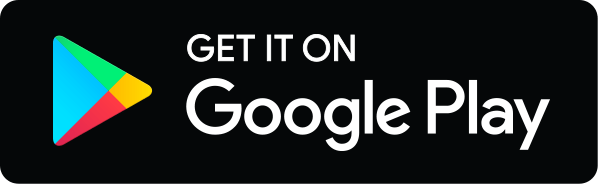
