CHAPTER 11 Interest in the circulatory contributions to osteoarthritis (OA) has been stimulated by the demonstration that experimental venous occlusion of bone is accompanied by hallmarks of OA bone — intraosseous hypertension, focal avascular necrosis, trabecular remodeling, and thickening of the subchondral bone plate. Subsequent observations demonstrated that decreased perfusion, venous outflow obstruction, andvenous stasis, are associated with late-stage OA of the hip and that these circulatory changes can result in hypoxia in subchondral bone. Sophisticated imaging techniques including dynamic contrast-enhanced magnetic resonance imaging (DCE-MRI) and positron emission tomography (PET) with a variety of isotopes are demonstrating venous outflow obstruction resulting in intraosseous hypertension, reductions in perfusion, hypoxia, and remodeling of trabecular structure and architecture. In response to changes in pressure, perfusion, and oxygen content similar to that seen in OA, OA osteoblasts alter their expression of structural and signaling cytokines relevant to bone remodeling and cartilage breakdown. The changes in bone circulation may contribute to the initiation or progression of OA through associated changes in the physicochemical environment of osteocytes in subchondral bone. The circulatory contributions to the pathology of OA have been emphasized by the venous ligation studies of Brookes.9 After venous ligation, he observed decreased bone blood flow, pO2, and pH. These changes were associated with trabecular remodeling and calcification of the deep cartilage zones. Other studies by this group demonstrated that venous obstruction is associated with increased intraosseous pressure and appeared causally related to the structural remodeling of bone. These studies were confirmed and extended some years later by Welch who showed in a caprine model that venous occlusion was associated with intraosseous hypertension and bone remodeling.53 Metaphyseal bone formation was demonstrated with an increase of periosteal (+138%), endosteal (+286%), and trabecular (+889%) bone. Bone remodeling resulted in new bone volume of +376% with increases in the numbers of both osteoblasts and osteoclasts. Together, these and other studies of venous occlusion demonstrate an association of intraosseous hypertension with the histopathological features of OA bone — focal avascular necrosis, trabecular remodeling, thickening of the subchondral bone plate, endosteal and periosteal new bone formation, and sclerosis. Studies of bone circulation of the human hip have been done by Arnoldi using static imaging with intraosseous venography.3 The intraosseous injection of 5 ml of contrast filled the venous system of the proximal femur. In normal hips, blood exited within 3–6 minutes in a centrifugal pattern through retinacular veins at the femoral neck (Fig. 11.1). OA hips were characterized by an absence of venous outflow through extraosseous retinacular veins and a centripetal pattern of venous drainage through diaphyseal intramedullary veins. Dilated intramedullary veins filled with contrast have been observed in OA.6 Intramedullary venous stasis persisted in the OA hips for greater than 30 minutes after injection of contrast. This marked temporal and functional difference between normal and OA hips suggested the presence of venous stasis in OA (Fig. 11.2). Venous stasis was suggested further by subtraction venography showing contrast accumulation around the trocar tip in OA hips that persisted for 20 minutes, while in normal hips, clearance of contrast was prompt, occurring within minutes after injection of contrast (Fig. 11.3). Fig. 11.1. Intraosseous venogram 30 seconds after contrast injection. Venous outflow of normal hips occurs through extraosseous retinacular veins at the femoral neck — s.r. = superior retinacular vein; i.r. = inferior retinacular vein. OA hips exhibit absence of retinacular venous flow and intramedullary venous drainage. (Reprinted from: Arnoldi CC, Linderholm H, Mussbichler H (1972). Venous engorgement and intraosseous hypertension in osteoarthritis of the hip. J Bone Joint Surg Br, 54(3), 409–421, with permission from The British Editorial Society of Bone & Joint Surgery.) In summary, these studies demonstrate that venous drainage in the normal hip (femur) occurs in a centrifugal pattern through extraosseous veins with a short emptying time, under 10 minutes. Venous outflow in OA femora occurs in a centripetal pattern through diaphyseal intramedullary veins and is markedly delayed with a considerably longer emptying time, suggesting not only anatomic variance from normal but also outflow obstruction and venous stasis. Other studies have indicated the presence of intraosseous hypertension in association with venous outflow obstruction, OA, and bone pain.4, 5, 29 Fig. 11.2. Intraosseous venogram 30 minutes after contrast injection. All contrast has exited from the normal hip. Persistence of contrast in intramedullary veins is observed in the OA hips suggesting venous stasis. (Reprinted from: Arnoldi CC, Linderholm H, Mussbichler H (1972). Venous engorgement and intraosseous hypertension in osteoarthritis of the hip. J Bone Joint Surg Br, 54(3), 409–421, with permission from The British Editorial Society of Bone & Joint Surgery.) While static imaging is useful for delineating anatomic patterns of blood flow, it cannot quantify perfusion characteristics. Dynamic imaging techniques with DCE-MRI and 18F PET can be used to characterize changes in regional perfusion in subchondral bone and correlate that information with bone metabolism of interest to the pathophysiology of OA. Pharmacokinetic modeling techniques permit the characterization of perfusion kinetics and insight into physiology of blood flow in bone. DCE-MRI offers the opportunity to extract kinetic information on bone circulation including bone blood flow, capillary permeability, and venous outflow.13, 31 PET using the bone-seeking isotope 18F fluoride provides estimates of bone blood flow and bone formation.35, 40 These parameters have been compared to the severity of cartilage lesions and bone remodeling, and temporal and spatial relationships have been described. These functional imaging techniques provide insight into pathophysiologic mechanisms that are associated with bone remodeling and cartilage degeneration in OA, and put previous static vascular studies into functional pathophysiological contexts. Fig. 11.3. Subtraction intraosseous venogram 1.5 minutes after injection of contrast. Accumulation of contrast is seen around the trochar tip in OA hips but not in normal hips reflecting venous stasis in OA. (Reprinted from: Arnoldi CC, Linderholm H, Mussbichler H (1972). Venous engorgement and intraosseous hypertension in osteoarthritis of the hip. J Bone Joint Surg Br, 54(3), 409–421, with permission from The British Editorial Society of Bone & Joint Surgery.) The techniques of DCE-MRI in subchondral bone include image acquisition, signal processing, and pharmacokinetic modeling, and have been described previously.1, 28 Briefly, signal enhancement in DCE-MRI is achieved with the use of a paramagnetic contrast medium, such as gadolinium, which causes local magnetic field fluctuations and signal enhancement in specific tissues. Signal enhancement and perfusion are proportional to the gadolinium concentration. Using pharmacokinetic models, the change in signal enhancement over time, described by signal intensity-time curves (Fig. 11.4), can be used to extract quantitative information concerning perfusion kinetics including the perfusion parameters, slope, amplitude (A), and elimination rate constant (kel).8, 48 These parameters reflect vascular inflow, extracellular contrast-agent retention, and vascular outflow in the perfused tissue. Regions of interest can be identified and kinetics measured for comparison. Fig. 11.4. Signal enhancement of the human femoral condyle. (a) Regions of interest are identified for comparison. (b) Time-intensity curve of the MRI signal demonstrating inflow slope, amplitude, and clearance or washout phases of perfusion. (Reprinted from: Lee JH, Dyke JP, Ballon D, Ciombor DM, Tung G, Aaron RK. (1972). Assessment of bone perfusion with contrast-enhanced magnetic resonance imaging. Orthop Clin North Am, 40(2), 249–257, with permission from Elsevier.) We have used DCE-MRI with gadolinium to describe abnormalities in perfusion in subchondral bone in the Dunkin-Hartley guinea pig model of spontaneous OA. The hypothesis underlying these studies is that changes in circulatory dynamics in subchondral bone bear functional relationships to bone remodeling and cartilage degeneration. One study explored relationships between perfusion in the subchondral bone and cartilage lesions of developing OA to characterize circulation kinetics and the time course of changes in perfusion in the developing arthritis.28 Changes of OA in subchondral bone and cartilage begin at about 9 months of age and are well established by 12 months of age. This study demonstrated a decrease in perfusion temporally preceding OA changes in cartilage (Fig. 11.5). Fig. 11.5. Decreased venous outflow (kel) temporally preceding cartilage degeneration. Cartilage degeneration begins at about nine months of age (Mankin score). The kel is markedly reduced between six and nine months of age, reflecting venous outflow obstruction. Decreased perfusion was only observed in the medial compartment at the site of eventual bone and cartilage lesions and, therefore, it was concluded that circulatory abnormalities spatially localized at the site of OA. Time intensity curves studies demonstrated that, at six months of age before the development of arthritis, bone circulation was normal with normal inflow, amplitude, and washout of contrast dye. By 12 months of age, lesions of OA in articular cartilage were visible. Time intensity curves demonstrated delayed venous outflow suggesting that the primary patho-physiological circulatory event was venous stasis (Fig. 11.6). These studies showed that decreased perfusion and venous stasis in developing guinea pig OA temporally preceded and spatially co-localized with the eventual morphological changes of bone and cartilage. A second study, using DCE-MRI and 18F PET, examined alterations in subchondral bone circulation and their relationships to bone structure and cartilage degeneration in the Dunkin-Hartley guinea pig with OA of varying severity.14 DCE-MRI used small molecular contrast medium (gadolinium, 530 Da) to characterize perfusion. Prolongation of signal enhancement and delayed contrast clearance (kel) during the washout phase was correlated with the severity of OA (p =0.015). Time intensity curves again suggested venous stasis in OA (Fig. 11.7). Fig. 11.6. Time-intensity curves in developing OA. At six months of age before OA, the kel = 0.06 indicating normal venous outflow. By 12 months of age, the kel = −0.11 reflecting venous outflow obstruction. (Reprinted from: Aaron RK, Dyke JP, Ciombor DM, Ballon D, Lee J, Jung E, Tung GA. (2007). Perfusion abnormalities in subchondral bone associated with marrow edema, osteoarthritis, and avascular necrosis. Ann N Y Acad Sci, 1117, 124–137, with permission from John Wiley and Sons.) To demonstrate that the changes in signal intensity were due to venous stasis, vascular permeability was measured with a macromolecular contrast medium (gadolinium-albumin, 92,000 Da). These studies showed no increase in vascular permeability with increasing severity of OA indicating that the increased signal intensity observed in OA is most likely not due to increased transvascular migration of gadolinium to the intraosseous extravascular space. These studies revealed a positive correlation of venous outflow obstruction with the severity of arthritis. 18F PET quantifies a perfusion phase within two minutes of radionuclide injection. The 18F is then taken up into the hydroxyapatite crystal to provide a measure of mineralization and osteoblastic activity. Fig. 11.7. Time-intensity curves in severe OA. Normal curve is seen with minimal OA (Mankin score of 1). Delayed contrast clearance is observed in severe OA (Mankin score of 14) reflecting venous stasis. 18F PET scans have been used in the Dunkin-Hartley guinea pig model to demonstrate bone blood flow and bone formation at the site of OA morphological lesions.14 The PET data have confirmed that decreased perfusion is related to the severity of OA and have extended that observation to show a relationship between changes in the circulatory environment in subchondral bone (decreased perfusion) and abnormal bone formation (decreased 18F-fluoride influx) associated with the severity of cartilage degeneration (Mankin scores). Specifically, we observed in the medial tibial plateau: (1) a decrease in blood volume (p = 0.005) and blood flow (p = 0.019) with increasing cartilage degeneration; (2) a decrease in radionuclide incorporation into bone matrix reflecting decreased bone formation with increasing severity of cartilage lesions (p = 0.05); and (3) a correlation between blood flow and 18F-fluoride in corporation into bone matrix (p < 0.001). These observations confirmed in functional terms associations among perfusion, bone remodeling, and cartilage lesions in OA. DCE-MRI has the capability of extracting kinetic information on bone circulation including bone blood flow, capillary permeability, and venous outflow. 18F PET, because it has both perfusion and osteogenic phases, can provide estimates of bone blood flow and correlate perfusion with bone formation. To the extent that perfusion is related to OA, these functional imaging techniques provide insight into pathophysiologic mechanisms that are associated with, and may precede, structural changes of bone remodeling and cartilage degeneration in OA. Fig. 11.8. Kinetic parameters of perfusion in normal OA knees. Data is derived from time-intensity curves. Signal amplitude (A) is greater in OA than in normal knees indicating accumulation of contrast media. The elimination constant (kel) is reduced in OA knees indicating venous outflow obstruction and stasis.
CIRCULATORY PATHOLOGY
IN OSTEOARTHRITIS
11.1 Introduction
11.2 Venous Stasis
11.2.1 Experimental interruption of venous outflow
11.2.2 Static imaging of human OA
11.2.3 Dynamic imaging of OA
11.2.3.1 DCE-MRI
11.2.3.2 18F PET
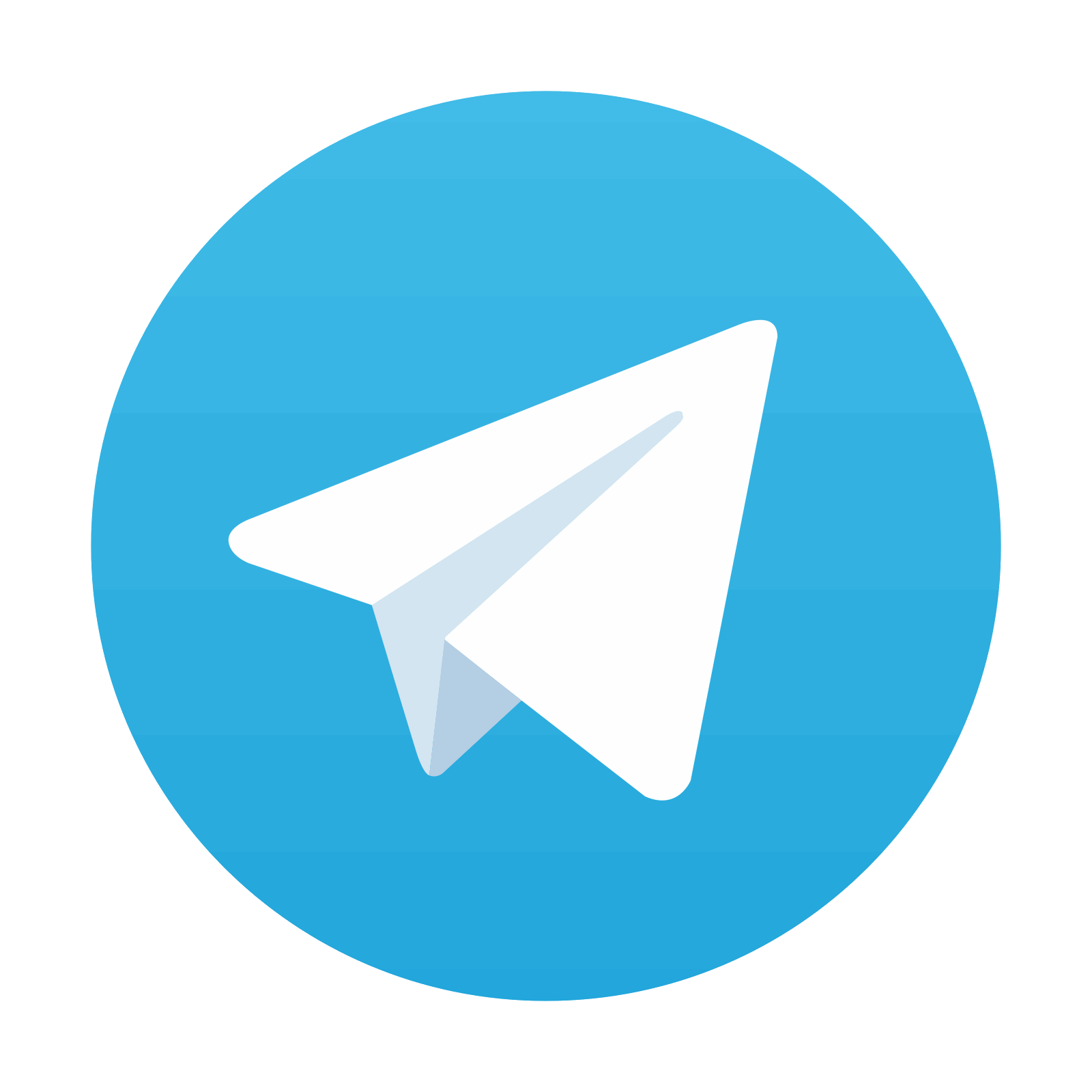
Stay updated, free articles. Join our Telegram channel

Full access? Get Clinical Tree
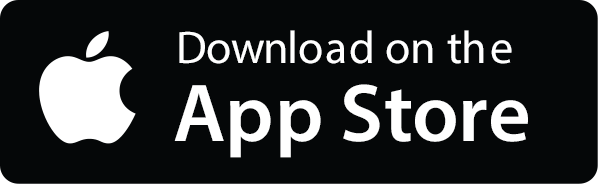
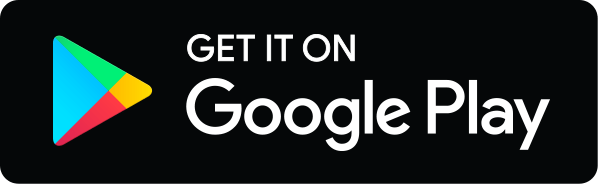