CHAPTER 13 Managing disorders affecting the vascularity of the hip remains a challenging dilemma for orthopaedic surgeons. From pediatric patients afflicted with Legg-Calve-Perthes disease or slipped capital femoral epiphysis to adults with post-traumatic avascular necrosis (AVN) of the femoral head, conditions injuring the blood supply to the femoral head continue to result in long-term poor clinical outcomes. In order to better understand this pathology, we must first understand the natural anatomy of the hip, and in particular, its vascular supply. The embryonic stage of human development encompasses approximately the initial eight weeks of development following fertilization of the oocyte. During the latter half of the embryonic stage, a majority of joint differentiation is completed. The remaining period of gestations is termed the fetal stage. It is during this time that the limbs and joints undergo continuing growth, maturation, and differentiation in preestablished spatial orientations.82 Limb formation begins at four weeks of intrauterine life with protrusions of the ventro-lateral wall of the embryo, or terminal buds.The limb buds consist of an outer ectoderm shell and an inner cellular mass of mesoderm. Bone, cartilage, muscle, tendon, and the joints arise from the mesoderm layer.59 The ectoderm gives rise to the skin, nails, and hair of the developing limb. At about six weeks following fertilization, the lower limb buds have continued to elongate and form terminal footplates. From these structures, cellular multiplication and differentiation continue to form a club-shaped cartilage model of the future femur. Chondroblasts condense at the proximal, central, and distal ends of the femoral template. Continued chondrification of precursor cells and fusion of the primitive chondrification centers leads to formation of the complete cartilaginous model of the femur by about seven weeks of gestation. Similarly, the acetabulum develops concurrently with the fusion of the primitive ilium, pubis, and ischium. The ilium and ischium are first to fuse, followed by the ilium and pubis. This occurs in rapid progression until finally the pubis and ischium coalesce during the seventh week of embryonic development. These two ossification centers leave a small opening laterally that results in formation of the acetabular fossa.84 At this stage in the late embryonic period, both the femoral and acetabular cartilaginous models have been fully formed. The mass of cells between these models now undergoes apoptosis to yield the beginning of the future synovial hip joint.84 During the eighth week of development, the primary ossification center of the femur appears in its shaft. Ossification proceeds both distally and proximally from this center. Concurrently, the arterial supply to the proximal femur begins its development with the appearance of the primary ossification center.82 At about the time of the transition from the embryonic to fetal stages of development, the primary ossification center of the femoral shaft has fully developed. Concurrently, capillaries deliver fibroblastic and hematopoietic cells into the marrow by breaking through the periosteum at the middle third of the femoral shaft cartilaginous anlage. This corresponds to the level of the adult nutrient artery. For the next four to six weeks, this remains the only feeding vasculature for the entire femur.82 At about 12 to 14 weeks, a vasculature ring forms around the neck of the femur, giving rise to the future medial and lateral circumflex vessels. The obturator and superior and inferior gluteal vessels also arise from this early vascular ring.59 Blood vessels connected to this ring invade the head and neck of the femur to give rise to the future retinacular vessels vital to the perfusion of the fully developed proximal femur. The formation of the blood supply to the acetabulum, similar to the development of the cartilaginous model, proceeds just after initiation of the arterial supply to the proximal femur. This occurs at 12–14 weeks of development. There is evidence of capillary invasion into the pulvinar and ligamentum teres at around eight weeks. However, the significance of this blood flow to the future development of the hip is controversial. The contribution of the ligamentum teres and pulvinar to the blood flow to the hip remains a disputed topic throughout the development and the life of the hip joint. No anastomosis with the arterial terminals in the femoral head was observed by Strayer until around age 15.82 At this stage, ossification of the femoral head is generally nearly complete, if not fully complete. Only one out of seven fetuses that he examined demonstrated vessels entering the femoral head from the ligamentum teres.These findings suggest that the artery of the ligamentum teres does not seem to contribute significantly to the development of the femoral head. Clinically, this holds true in the treatment of the dysplastic hip undergoing osteotomy and open reduction of the hip joint itself. During the procedure, the ligamentum teres is routinely sectioned as it has become elongated and redundant due to lateral subluxation of the femoral head from the acetabulum. This increased size and length make it a block to concentric reduction of the femoral head into the acetabulum. Routinely, the ligamentum teres is completely excised. Clinically, this has not been reported to cause adverse consequences to the further development of the femoral head. The arterial supply to the proximal femur and acetabulum established during fetal life largely endures throughout remaining childhood. Chung demonstrated this in a perfusion study of fetuses and children aged 26 weeks to 14 years.18 An extracapsular arterial ring formed by the conjoining of branches from the medial and lateral circumflex arteries gives rise to ascending retinacular or cervical vessels that enter the proximal aspect of the hip joint capsule. These retinacular vessels ascend the femoral neck intra-articularly toward the femoral head. They are divided into four groups based upon their location on the femoral neck: lateral, posterior, medial, and anterior. Branches of the retinacular arteries enter the femoral neck to supply the greater trochanter laterally and the femoral neck medially, or anastomose with the ascending nutrient vessels from the femoral shaft.59 As the cervical vessels continue to track proximally, they form another anastomosis at the junction of the femoral neck and the articular cartilage of the femoral head.59 This gives rise to an intra-articular subsynovial ring. This ring in turn gives rise to epiphyseal and metaphyseal branches which provide arterial nourishment to the most proximal portion of the femur. The metaphyseal branches invade the femoral neck and proceed distally. The epiphyseal branches gain access to the epiphysis by crossing the perichondrial ring superficially and then entering the cartilaginous femoral head. None of these vessels directly transverse the physeal plate. The subsynovial ring can sometimes be incomplete. This is more common in males and may have implications in the predilection of Legg-Calve-Perthes disease in the male gender. The ring is also typically more robust on the medial and lateral aspects of the femoral neck as compared to the anterior and posterior surfaces.18 The overall redundancy of the blood supply of the acetabulum makes pathologic conditions resulting from interruption of blood flow to this area far less of a clinical concern. The adult blood supply of the acetabulum is established early in development and has no significant alterations from childhood anatomy. Branches of the superior gluteal artery supply the acetabular dome with blood flow, whereas the inferior gluteal artery provides nutrient vessels to the medial acetabulum. Finally, a posterior branch of the obturator artery provides nourishment to the inferior acetabular bone (Fig. 13.1).72 An extensive network of intra- and extraosseous anastomoses between these vessels makes AVN of the acetabulum extremely rare.59 This is true even in the setting of various methods of pelvic osteotomy done for the treatment of any number of hip disorders in the young child. The physeal plate provides the main anatomic variation in the proximal femur of children as compared to mature adults. This cartilaginous growth plate serves as a barrier to vessels, which do not cross it. As discussed above, the ephiphyseal branches of the subsynovial ring formed from the anastomosis of the ascending cervical arteries, reach the femoral head by tracking along the periphery of the physis at the perichondrial ring. There is, however, an extraosseous anastomosis of the intraosseous circulation of the neck and head of the femur at the subsynovial ring. When the physis closes at maturity, vessels can then course through the femoral neck to the femoral head and form an intraosseous connection.20 Fig. 13.1. Lateral (a) and medial (b) views of the right acetabulum with its associated vascular supply. Note the clockwise arrangement of the superior gluteal artery, obturator artery, and inferior gluteal artery contributions to the acetabulum. Source: Beck M, Leunig M, Ellis T, Sledge B, Ganz R (2003). The acetabular blood supply: Implications for periacetabular osteotomies. Surg Radiol Anat, 361–367. Reproduced with permission. It is widely recognized that in the mature adult, the medial circumflex artery and its terminal branch, the lateral portion of the extracapsular arterial ring, provide the majority of the blood supply to the femoral head, neck, and great trochanter.18 Gautier et al. described the anatomy of the medial circumflex artery in great detail by examining 24 cadaveric hips.31 They described five consistent branches: deep, descending, acetabular, ascending, and superficial. The deep branch was found to enter the hip capsule and divide into terminal branches termed the lateral epiphyseal arteries. These arteries would in turn course beneath the synovial sheath of the reflected capsule posterosuperiorly to insert into the neck of the femur.31, 79 A maturation process occurs in the circulation of the proximal femur throughout childhood to result in this supply. Whereas the number of lateral and posterior ascending cervical arteries remains constant through maturation, the number of vessels along the anterior and medial femoral neck decreases by about 50%.18 This was confirmed in a separate study by Lauritzen that demonstrated that the lateral retinacular arteries supply the majority of blood supply to the femoral head by the age of ten.58 Also during the course of development, independent vascular territories in the femoral head defined by the epiphyseal branches supplying their blood flow coalesce into a larger network via anastomoses between the vascular zones. This network may not be complete, and therefore occlusion of specific ascending retinacular arteries may cause necrosis of specific defined anatomic zones.59 With a clearer understanding of the normal vascular supply to the hip, we can understand more fully the clinical conditions that cause injury to this system of vasculature and the resulting pathology. AVN following femoral neck fractures in children is a known and feared phenomenon.There is no general consensus on the exact causative factor in the development of interrupted blood flow following trauma to the femoral neck. More likely, a combination of factors plays a role in the development of AVN. Moon and Mehlman performed a systematic review of 360 cases of femoral neck fracture in children aged 1 to 16 years old and found that fracture type, displacement, age of the patient, and treatment type were all independent predictors of AVN.66 However, after performing logistic regression analysis, fracture type and age were the only two statistically significant variables with regard to the development of AVN. Transphyseal and transcervical fractures were associated with the highest rates of AVN. Intracapsular fractures were demonstrated to have a 14-fold increase in the frequency of AVN as compared to extracapsular fractures (Table 13.1). Extracapsular fractures likely cause less direct injury to the subsynovial ring, which provides blood flow to the proximal femoral epiphysis. In addition, with each advancing year of age, the older child was 1.14 times more likely to develop AVN.66 A number of studies have supported higher rates of AVN in older children as compared to their younger counter-parts.50, 66, 73 With the changing vascularity of the proximal femur discussed above, this may be a predictable consequence. The physis remains open, thus inhibiting intraosseous blood supply to the femoral head. Also, the number of retinacular vessels decreases, particularly those along the anterior and medial femoral neck. The younger child seems to have an increased ability to revascularize and remodel the femoral head, possibly due to the more abundant collateral blood flow to the femoral head during earlier ages in childhood prior to the loss of those ascending cervical vessels originating from the lateral circumflex artery. Table 13.1. AVN risk as related to location of femoral neck fracture. Adapted from Moon ES, Mehlman CT. (2006). Risk factors for avascular necrosis after femoral neck fractures in children: 25 Cincinnati cases and meta-analysis of 360 cases. J Orthop Trauma, 323–329. Reprinted with permission. There is a general consensus that displacement of a femoral neck fracture is an important predictor in the development of AVN.66 This would seem to be consistent with the hypothesis that AVN results from direct trauma to the retinacular vessels supplying the proximal femur. However, this does not offer an explanation as to the development of AVN following non-displaced femoral neck fractures. It has been hypothesized that increased intracapsular pressure following an acute hemarthrosis from an intracapsular fracture, can cause a tamponade effect and compromise blood flow to the femoral epiphysis. It has been suggested by some authors that treatment of acute femoral neck fractures should include a decompression of the hip joint capsule. However, the protective nature of this procedure has not been convincingly demonstrated in the literature to date. By the above rationale, a displaced fracture could be somewhat protective by causing a rupture of the hip joint capsule and thus preventing this tamponade effect. There is conflict in the existing literature relating to the role of surgical intervention in the development of AVN. This is clearly very difficult to assess due to the constantly evolving surgical techniques, implantable devices available for fixation, and treatment protocols utilized to treat the acute femoral neck fracture. Currently, it is generally felt that obtaining an anatomic reduction and providing stable fixation is the standard of care in treating these fractures. This provides the appropriate anatomic scaffolding that the vascularity of the hip depends upon. Displaced fractures may cause spasm or occlusion of retinacular vessels and thereby diminish blood flow to the femoral head. By restoring proper anatomy, the spasm or occlusion of injured, but not ruptured; vessels may resolve and re-establish blood flow to the femoral head. Conversely, there is literature demonstrating that repeated reduction attempts or the use of internal fixation can cause further damage to the blood supply to the femoral head and increase the risk of AVN.25, 73 However, there is significant selection bias in many of the studies demonstrating poor outcomes in operatively treated patients. The operatively treated patients tended to have more displaced fractures that could certainly have had more damage to the retinacular vessels even prior to surgical intervention. More recent studies have shown better outcomes with early reduction and fixation of displaced femoral neck fractures, sometimes with arthrotomy of the hip capsule as well.17, 26, 81 Dendane et al. demonstrated that a delay in reduction greater than 48 hours was an independent risk factor for the development of AVN as well.22 The data outlined above, as well as the vast amounts of data existing in the orthopedic literature, do not associate the development of AVN following a femoral neck fracture with one specific risk factor. Rather, progression to AVN likely develops due to the interrelationship of a number of factors including the location of the fracture, the age of the patient, and noniatrogenic and iatrogenic trauma to the retinacular vessels supplying the femoral head. Our understanding of the anatomy of the blood supply to the proximal femur can help the physician counsel patients about the risks of developing AVN after a femoral neck fracture. Unfortunately, the currently available knowledge base does not offer a reliable method of preventing AVN or successfully treating AVN after it develops. Although not technically the same as a transphyseal fracture of the femoral neck, a slipped capital femoral epiphysis (SCFE) has similarities to an acute femoral neck fracture, particularly as it pertains to the development of AVN. Unlike a femoral neck fracture, which results from acute high-energy trauma, a SCFE is thought to occur through a diseased and weakened physis with minimal to no trauma. The femoral head remains in the acetabulum, retroverted from the femoral neck and shaft, which have displaced anteriorly. Much research has been directed to the study of AVN resulting from SCFE due to reports that the treatment of SCFE resulted in iatrogenic disruption of the blood flow to the proximal femur during manipulation of displaced SCFEs.14, 16 This has numerous medical legal implications to the practicing orthopaedic surgeon. A differentiation must be made between an unstable and stable slipped capital femoral epiphysis. This classification first described by Loder has important treatment and prognostic implications. A stable SCFE is defined by Loder as an SCFE upon which the child can bear weight, with or without the use of assistive devices. The patient with an unstable slip cannot bear weight at all on the injured extremity. The practical implications of this classification system are that the stable SCFE tends to be the result of a slowly progressive and chronic developing deformity. In contrast, the unstable SCFE is more of an acute event involving significant deformity, which develops nearly instantaneously. The ramifications for the development of AVN cannot be overstated. Whereas the stable SCFE has a very low rate of AVN approaching zero,16, 38, 69 the unstable SCFE has rates of AVN reported as high as 50%. The pathoanatomy of an unstable SCFE is likely quite similar to that of an acute femoral neck fracture, particularly as it pertains to the development of AVN. An angiographic study performed by Maeda et al. elegantly described the vascular anatomy of the retinacular vasculature as it relates to SCFE.63 They demonstrated via selective angiography of the medial circumflex artery that the posterior ascending cervical branch was filled in all stable SCFE hips. The superior retinacular artery branching off the posterior ascending cervical artery formed an acute angle with that feeding vessel as it coursed posteromedially to the retroverted femoral head. However, all five stable SCFEs studied had intact perfusion of both of these vessels. This was found independent to the degree of slip, or amount of deformity that occurred as a result of the SCFE. Of the five unstable SCFE hips examined, the posterior ascending cervical artery was filled in two out of the five hips. Of the three hips that did not show filling of the posterior ascending cervical artery, one went on to develop AVN. Interestingly, an angiographic exam was conducted on one unstable slip before and after a gentle manipulation to correct the alignment of the proximal femur. In that particular hip, there was no flow in the posterior ascending cervical artery prior to manipulation. However, there was reconstitution of flow following gentle manipulation into correct anatomy. These findings seem to suggest that the vascular insult occurs at the time of an acute unstable slip due to interruption of blood flow through the ascending cervical arteries. This is similar to the likely pathoanatomy in the acute fracture of the femoral neck. This interruption, at least in some patients, can be due to a kinking of the vessels, which can be relieved by manipulation back into correct anatomy. It is important to note, however, that this does not take into account possible surgical damage to the vasculature caused by an open approach to the hip. Many studies have demonstrated much lower rates of femoral head AVN in stable as opposed to unstable SCFEs.16, 38, 69 This is in concordance with the findings of Maeda et al. that demonstrated intact superior retinacular vessel flow in all stable slips.63 This was independent of the degree of deformity noted and thought to be related to the slow development of femoral head retroversion in stable SCFEs. This theoretically gives the retinaculum and the retinacular vessels time to slowly stretch and accommodate the deformity. The angle between the posterior ascending cervical branch and the superior retinacular artery was found to be much more acute in stable SCFEs when compared to normal hips.63 A rare complication of a rare injury provides more insight into the tenuous vascular supply to the proximal femur. As discussed previously, injury to the posterolateral branches of the medial circumflex artery has been associated with the development of AVN of the femoral head. There have been a few case reports in the literature describing AVN of the femoral head following greater trochanteric fracture of the proximal femur.49, 62, 70 In the developing child, the femoral capital epiphysis and greater trochanteric apophysis share a common physeal plate. The differential growth rates between the medial and lateral aspects of this physis during development leads to the final formation of the adult proximal femur. To review, Chung demonstrated that the greatest arterial supply to the femoral head originated from a branch of the medial circumflex femoral artery at the posterosuperior aspect of the hip capsule at the junction of the greater trochanter and the base of the femoral neck.18 He termed this artery the lateral ascending cervical artery. This artery was often found to be a single artery crossing the base of the capsule prior to branching into multiple posterosuperior epiphyseal vessels and supplying the majority of blood to the growing femoral epiphysis.18, 70 As the contribution from medial epiphyseal vessels decreases in the growing child, the lateral ascending cervical artery takes on much more importance. This is particularly true since Chung found only two of the 26 specimens that he examined had an anastomosis between the medial and lateral circumflex vessels.18 Therefore, injury to the isolated lateral ascending cervical artery prior to closing of the proximal femoral physis causes an interruption to the majority of femoral head blood supply as there is no intraosseous route for blood flow into the proximal femoral epiphysis. When the greater trochanter is fractured, particularly with anterior avulsion by the hip abductors, the medial circumflex artery can be disrupted.70 At this terminal location, it is tethered by the lateral ascending cervical artery and the descending metaphyseal arteries piercing the femoral neck proximal to the physis, making it susceptible to injury. The mechanism by which dislocation of the femoroacetabular joint results in AVN of the femoral head is thought to be similar to those discussed in relation to femoral neck fracture, SCFE, and greater trochanteric fracture. A direct traumatic injury, by longitudinal stretch and resulting vessel spasm or avulsion, limits blood flow to the susceptible femoral head circulation resulting in AVN. The earliest histologic change noted in AVN is diffuse cell death in the marrow space, disorganization of the marrow stroma, and loss of osteoblasts lining the trabeculae.55 Empty lacunae appear in the necrotic bone within a few weeks. Chondronecrosis of the deep layer of articular cartilage surrounding the secondary ossification center occurs shortly after the onset of necrosis.53 The physis, however, shows limited cell death; what cell death does occur, happens on the epiphyseal side of the physis.52 The vascularity of the metaphysis and femoral neck is well maintained by the anastomoses with the femoral shaft circulation. Revascularization of the femoral head occurs via fibrovascular tissue invasion of the necrotic marrow and bone.54 Osteoclast activity is then observed to return in this area; however, osteoblastic bone formation does not seem to immediately return. The mechanical failure allowing collapse of the femoral head may likely involve the loss of normal bone metabolism as evidenced by the loss of normal balanced osteoblastic and osteoclastic activity. Legg-Calve-Perthes (Perthes) disease is a self-limiting idiopathic condition of the hip in young children leading to osteonecrosis of the femoral head, which progresses through four distinct radiographic stages. During the first, or initial, stage the femoral head ossific nucleus appears more radiodense and smaller than the unaffected side. The medial joint space also appears wider, likely due to hypertrophy of the epiphyseal cartilage. During the second, or fragmentation, stage, the ossific nucleus has areas of increased radio-density and radiolucency giving the appearance of a “fragmenting” femoral head. The third, or reparative, stage is characterized by re-ossification of the ossific nucleus of the femoral head with return of more normal radiographic appearance of the bone density. In the final, or healed, stage, the process is completed and the proximal femur is left with any residual deformity. The spherecity of the femoral head and the congruency of the hip joint, at this stage ultimately determine the long-term prognosis as it relates to function of the hip, pain, and development of future osteoarthritis. Perthes disease cannot be compared with AVN following femoral neck fracture or slipped capital femoral epiphysis. Healing from these processes occurs rapidly without progressing through the prolonged stages of fragmentation and repair seen in Perthes disease.76 The presumed etiology of Perthes disease has gone through many iterations throughout the years of advancing medical knowledge. Most early theories centered on an inflammatory or infectious etiology.74, 75 Congenital and traumatic etiologies have also been proposed. However, most recent theories suggest some form of vascular embarrassment to the proximal femur. Hemodynamic alterations, endothelial injury, and hypercoagulability or hypofibrinolysis have all been proposed as possible pathogenic factors.4 Perthes disease is most common between the ages of four and eight, and also more common in the male gender by a 4:1 ratio when compared to females. These tendencies correlate well with Chung’s findings of an incomplete subsynovial articular ring more commonly found in boys.18 Also, as the child continues to mature, fewer vessels are present along the anterior and medial neck of the proximal femur to provide collateral flow to the femoral head, which could explain why Perthes is rarely seen prior to age four. The epiphyseal and metaphyseal branches of the lateral ascending cervical artery, which contribute the majority of the blood supply to the proximal femoral epiphysis, originate from a single vessel that crosses the trochanteric notch to pierce the hip capsule.82 The space between the femoral neck and the trochanter is extremely narrow in children under the age of eight,82 which results in this single artery being vulnerable to occlusion at this point as it crosses into the hip joint. Whatever blood supply to the femoral head arises from the ligamentum teres likely does not affect Perthes disease, as it has not been shown to vary by age or sex.82 Shore et al. examined 22 hips in 19 children with healed Perthes disease with high-resolution contrast-enhanced magnetic resonance imaging (MRI) to visualize the path of the medial circumflex artery and the lateral epiphyseal arterial branches supplying the femoral head.79 That study demonstrated a significant decrease in lateral epiphyseal arteries inserting into the femoral head (2.63 on average) when compared to controls with developmental dysplasia of the hip (5.2 on average). In Chung’s work, he identified an average of 4.1 lateral vessels in 150 pediatric specimens.18 Shore also found that despite the fewer number of lateral epiphyseal vessels, their caliber also was diminished when compared to controls. These results were consistent with previous angiographic work done by Atsumi et al., which demonstrated 68% of patients with Perthes disease had disruption of the true lateral epiphyseal artery at its origin at the joint capsule.6 Their work also found that in the healing phase, the lateral epiphyseal arteries were small in caliber and in an irregular pattern. A scintographic classification system based upon reperfusion of the lateral epiphyseal arteries has been proposed by Conway.19 He and others hypothesize that the prognosis for children with Perthes disease is related to the reperfusion of the lateral epiphyseal arteries. In his classification system, he describes that revascularization can occur via two methods: either recanalization of the existing vessels which is a quick process, or by slower neovascularization through the development of new vessels. “Lateral column” visualization on bone scintography carries a good prognosis. However, neovascularization is demonstrated by a “mushroom filling” appearance and is associated with a poorer prognosis.79 This system has clear parallels with the lateral pillar classification proposed by Herring that has widespread acceptance as an important prognostic indicator in Perthes disease. The Herring classification is based upon the radiographic maintenance of the height of the lateral pillar (lateral third) of the femoral head throughout the stages of Perthes disease. Diffusion MRI has also been utilized to evaluate the changes in vascularity in the pathologic femoral neck of Perthes disease. It has been demonstrated that there is an early and significant increase in the apparent diffusion coefficient (ADC) in the femoral neck of a Perthes hip, when compared to normal controls.13, 43, 60, 65 This relates to an increase in neovascularization and revascularization occurring through the osseous femoral neck as opposed to through the lateral epiphyseal arteries (Fig. 13.2). Much like Conway’s scintographic data, higher ADCs are associated with more neovascularization of the femoral neck. This in turn correlates with decreased recanalization of the lateral epiphyseal arteries and a worse prognosis. Boutault et al. demonstrated a correlation between increase in ADC and the Catterall classification.13 This classification system is based upon the radiographic appearance of femoral head involvement with hips having more complete epiphyseal involvement having higher scores and, therefore, worse prognoses. Fig. 13.2. Seven-year-old child, four months after the onset of symptoms: (a) T1 sequence LCPD on the right side in the fragmentation stage; (b) DWI: b0 cut passing through the necks of the femurs; (c) DWI: b1000 cut for the same area; (d) corresponding ADC cartography showing a hypersignal of the pathological side compared to the left side (ADC increase by 95%). Source: Boutault JR, Baunin C, Berard E, Vial J, Labarre D, Domenech C, Sales de Gauzy J, Sans N(2012). Diffusion MRI of the neck of the femur in Legg-Calve-Perthes disease: A preliminary study. Diagn Interv Imaging, 78–83. Reproduced with permission.
CIRCULATION OF THE PEDIATRIC
AND ADOLESCENT HIP
13.1 Development of the Vascularity of the Hip
13.1.1 Embryology of the hip
13.1.2 Fetal development of the arterial supply to the hip
13.1.3 Childhood arterial supply to the hip
13.2 Traumatic Clinical Conditions Related to Pathologic Vascularity
13.2.1 Femoral neck fractures
Fracture Location
% Cases
AVN %
Transepiphyseal
8.1
38
Transcervical
40.3
28
Cervicotrochanteric
33.3
18
Intertrochanteric
18.3
5
13.2.2 Slipped capital femoral epiphysis
13.2.3 Fractures of the greater trochanter
13.2.4 Hip dislocation
13.3 Histology of Avascular Necrosis
13.4 Non-Traumatic Clinical Conditions Related to Pathologic Vascularity
13.4.1 Legg-Calve-Perthes disease
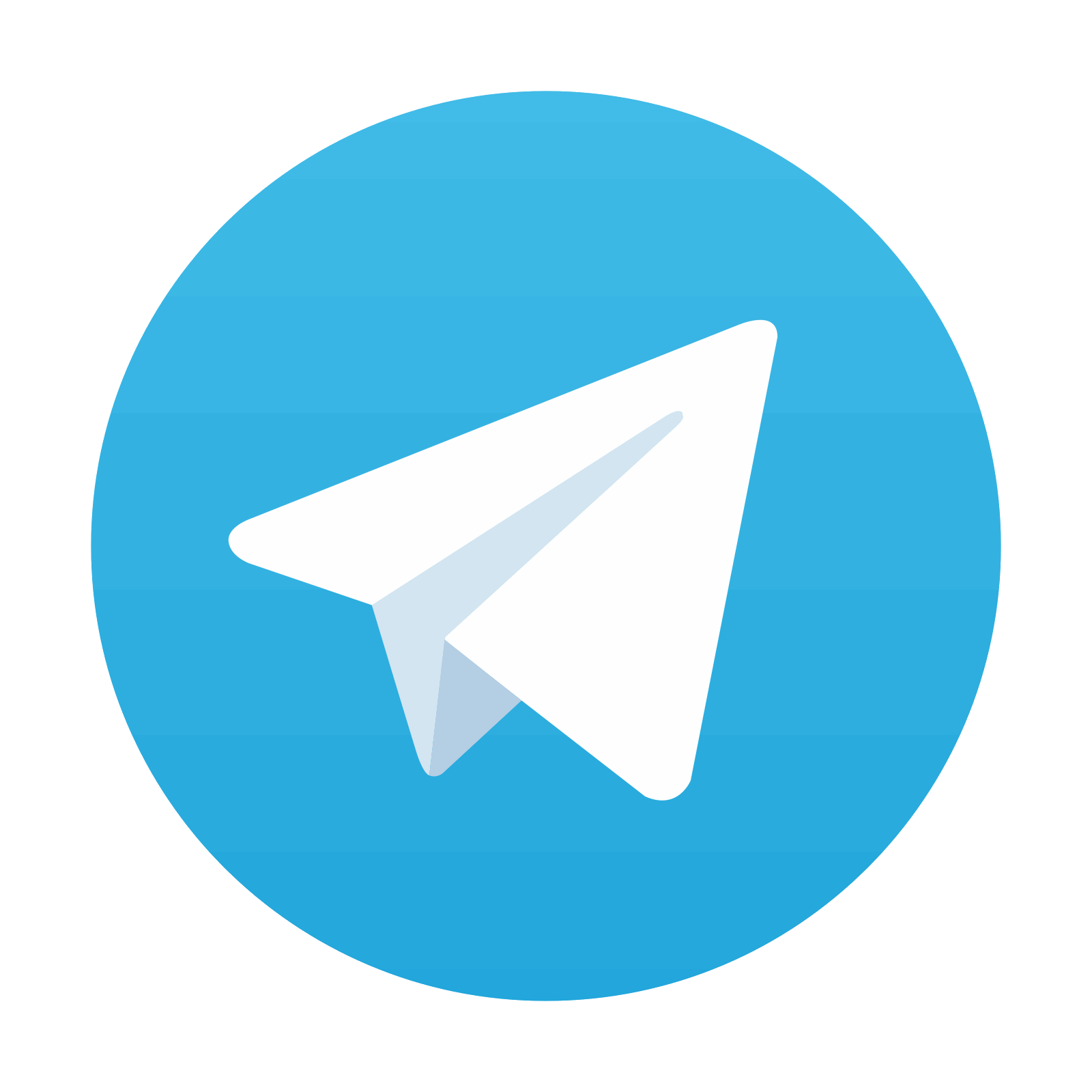
Stay updated, free articles. Join our Telegram channel

Full access? Get Clinical Tree
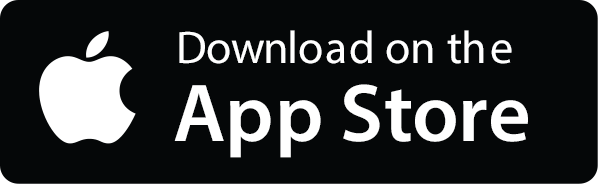
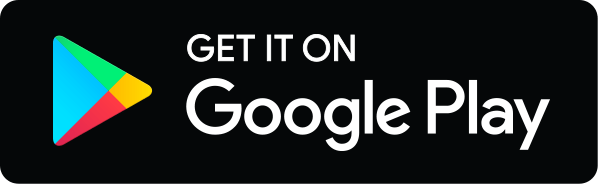