Circadian Rhythm Sleep Disorders Management
R. Robert Auger
Sleep and wakefulness are conceptually governed by two processes, “process S” and “process C” (1). The homeostatic drive to sleep (process S) is proportional to the duration of sleep restriction, and becomes maximal at about 40 hours (2). In contrast, process C creates a drive for wakefulness that variably opposes process S and is dependent upon circadian (“approximately daily”) rhythms intrinsic to the organism (Fig. 13.1). Master coordination of this sleep/wake rhythm (and numerous other behavioral and physiologic variables) is provided by the neurons of the suprachiasmatic nuclei (located within the hypothalamus) (3, 4, 5 and 6), whose rhythmic output results from an autoregulatory feedback loop, within which oscillating circadian gene products regulate their own transcription and translation (reviewed in [7]). As this intrinsic period is typically slightly longer than 24 hours (8), synchronization to the 24-hour day (entrainment) is accomplished by various environmental inputs (zeitgebers, or “time givers”), the most important of which is photic exposure (9).
Misalignment between endogenous circadian rhythms and the light/dark cycle can result in pathologic disturbances in the form of erratic sleep timing (irregular sleep-wake rhythm), complete dissociation from the light/dark cycle (circadian rhythm sleep disorder, free-running type), delayed sleep timing (delayed sleep phase disorder), or advanced sleep timing (advanced sleep phase disorder). Whereas these four conditions are thought to involve predominantly intrinsic mechanisms, circadian dysrhythmias can also be induced by exogenous challenges, such as those imposed by extreme work schedules or rapid transmeridian travel, which overwhelm the ability of the master clock to acclimate with commensurate rapidity, and in turn impair approximation to a desired sleep schedule, as evidenced by the shift work and jet lag sleep disorders (10). This review will focus on evidencebased treatment options for circadian rhythm sleep disorders (CRSDs). Topics are subcategorized when applicable and if sufficient data exist. The length of text associated with each disorder compositely reflects the abundance of associated literature, complexity of management, and the expected prevalence of each condition within general medical practice. The clinical presentation, epidemiology, classification, diagnostic evaluation, and differential diagnosis associated with the CRSDs are reviewed elsewhere.
JET LAG SLEEP DISORDER
Jet lag is a direct result of humankind’s ability to traverse numerous time zones in a short period of time. In addition to nocturnal sleep disturbances and impaired daytime alertness, other symptoms include general malaise and gastrointestinal distress (10). Although of minor consequence to some, debilitation can occur to a degree that engagement in business or other productive activities is compromised. The severity of impairment is dependent upon numerous variables. As would be predicted based upon the average length of the human circadian period (8), westward travel is typically less taxing than eastward travel (as it requires a phase delay, rather than a phase advance). As maximal daily delays and advances can occur on the order of 2 and 1.5 hours, respectively (11,12), traversal of two or less time zones is only transiently problematic for most individuals (if at all). The degree to which
travel occurs in excess of this “window” correlates to the rapidity at which the circadian pacemaker can re-entrain, which in turn contributes to the duration and magnitude of associated symptoms. An individual’s innate circadian preference (i.e., their relative “morningness” or “eveningness”) may also confer a greater or lesser ability to adjust to a particular time shift, but this has not been systematically assessed.
travel occurs in excess of this “window” correlates to the rapidity at which the circadian pacemaker can re-entrain, which in turn contributes to the duration and magnitude of associated symptoms. An individual’s innate circadian preference (i.e., their relative “morningness” or “eveningness”) may also confer a greater or lesser ability to adjust to a particular time shift, but this has not been systematically assessed.
One’s ability to sleep at an abnormal circadian phase (“phase tolerance”) also affects the degree to which adverse symptoms are experienced, and this adaptability varies among individuals (13,14). In a simulated jet lag study (requiring a 6-hour phase advance), middle-aged subjects (37-52 years) experienced a greater degree of polysomnographically-recorded fragmented sleep than their younger counterparts (18-25 years), and also exhibited greater impairments in daytime alertness, suggesting that advanced age reduces phase tolerance (14). Conflicting results were obtained from two field studies, however (involving both eastward and westward travel), which suggested that age may actually be protective with respect to the development of this syndrome (15, 16 and 17). Methodological differences preclude direct comparisons of the investigations, as do wide variances in the age groups studied.
Proper exposure and avoidance of light upon arrival to the travel destination can facilitate adaptation to the local time zone, while improper practices can impede progress. In a common process known as antidromic re-entrainment, phase shifts occur in a direction opposite to the shift in external time (e.g., a phase delay instead of a phase advance occurs subsequent to eastward travel), the occurrence of which is dependent upon the number of time zones crossed and ambient light exposure in the local area (12,18). As an example, a typical flight from Chicago to Paris (7 hours eastward) will arrive early in the morning at the travel destination. Upon viewing Figure 13.2, taking into account that circadian rhythms will not yet have shifted, one can see that the core body temperature minimum (MCBT; inverted triangle) will occur during the early afternoon in the destination time zone (equivalent to early morning at home-based clock hours). As the MCBT serves as an inflection point to determine whether light exposure will delay or advance circadian rhythms (before or after the MCBT, respectively; see Fig. 13.3), one can see that there is a greater propensity to delay rather than advance circadian rhythms, creating a scenario whereby circadian re-entrainment (and dissipation of jet lag) is delayed (19). Preventative strategies to reduce the occurrence of this phenomenon will be discussed in more detail below.
![]() FIGURE 13.3 A schematic human phase response curve (PRC) to light (dark line) and a PRC to exogenous melatonin (dashed line). The y-axis shows the direction and relative magnitude of the phase shift produced by the administration of light or melatonin at various times, which are shown on the x-axis. The x-axis covers more than 24 hours in order to better illustrate the PRCs. The rectangle represents the sleep episode, the triangle represents the core body temperate minimum (MMCBT), and the arrow represents the dim light melatonin onset (DLMO). The clock time axis shows the DLMO at about 22:00, the sleep interval from 00:00-08:00, and the MMCBT at about 05:00. These represent typical times and phase relationships among these rhythms when the circadian clock is entrained to a 24-hour day. For individuals with earlier or later circadian rhythms the local time axis should be adjusted accordingly. The light PRC is a schematic based upon the results of numerous studies. The melatonin PRC is based upon a single study using 0.5-mg doses of melatonin [reviewed in (37)]. (Reprinted from: Burgess HJ, Sharkey KM, Eastman CI. Bright light, dark and melatonin can promote circadian adaptation in night shift workers. Sleep Med Rev 2002;6(5):407-420, with permission.) |
Clearly, numerous other variables related to extensive travel contribute to the overall symptoms of travel fatigue, in the absence of a direct circadian correlate. These include sleep deprivation preceding (or en route to) the destination, acute discomfort related to the aircraft’s ascent to higher altitudes (20), and excessive alcohol and/or caffeine intake on flight. While effects of such variables will rapidly dissipate once one reaches a comfortable travel destination, jet lag symptoms will persist until circadian re-entrainment occurs (11).
Treatment of Jet Lag
The goal of treatment is to achieve circadian realignment in the most rapid and efficient way possible and to minimize associated symptoms during the interim period. Prior to proceeding with a treatment plan, however, it is important to determine the length of stay in the new time zone. Frequent shifts to differing time zones (such as those required by business travelers) are impossible to accommodate, and the individual may actually do better remaining on the home-based schedule, insofar as this is practical. In one balanced crossover field study, comparisons were made between subjects that kept home-based sleep hours versus those that adopted destination hours, during a 2-day stay after a 9-hour westward flight (21). The group
that remained on home-based hours exhibited reduced sleepiness and global jet lag ratings than the group that reverted to the destination time, in part due to improved sleep quality and duration. Nevertheless, approximately one-third of participants expressed a preference for adherence to the travel destination schedule.
that remained on home-based hours exhibited reduced sleepiness and global jet lag ratings than the group that reverted to the destination time, in part due to improved sleep quality and duration. Nevertheless, approximately one-third of participants expressed a preference for adherence to the travel destination schedule.
Timed Light Exposure
If the duration of time to be spent at the travel destination is sufficiently long, strategic avoidance and exposure to light can facilitate re-entrainment and avoid an antidromic process (19). Burgess and Eastman have devised “jet lag plans” that allow one to determine whether a phase delay or phase advance is most desirable, depending on the number of time zones crossed (22,23). The first step in this process is to create a grid with concurrent listing of home and destination times. In the example depicted in Figure 13.2, the individual is traveling seven time zones east, which will require a phase advance. On day 0, a rectangle is drawn around the times representative of home-based sleep hours. A symbol (inverted triangle) is subsequently added to represent the MCBT, which serves as an inflection point to determine whether light exposure will delay or advance circadian rhythms (before or after the MCBT, respectively, see Fig. 13.3). The precise placement is based on known relationships between the MCBT and habitual wake time, resulting in positioning either 2 or 3 hours prior to sleep offset, depending upon whether one typically sleeps 7 or fewer hours or more than 7 hours, respectively (22,24). At the bottom of the grid, a rectangle is drawn to represent the desired sleep schedule at the traveler’s destination, with inclusion of an MCBT symbol with the same relationship to the wake time described above. The distance between the home and destination-based MCBT symbols is representative of the required degree of circadian realignment. Generally speaking, shifts earlier in time are required for eastward flights (as in Fig. 13.2), and shifts later in time are required for westward flights. Advances of 8 hours or more are more readily accomplished by phase delays, however, as shown in Figure 13.4 (22,25).
If advances are required, the MCBT symbol is drawn on day 1 in the same location as day 0. For each subsequent day, the symbol is moved 1 hour earlier, based upon approximations of maximal phase advances (11,23), until a clock time within 1 hour of the desired destination MCBT time is reached and/or satisfactory sleep and daytime functioning is achieved (see Fig. 13.2) (22). If delays are required, the MCBT symbol is drawn 2 hours later on day 1 than on day 0 (reflecting the greater ease at which delays are achieved) (11,23), with subsequent daily shifts in 2-hour increments, again until a clock time within 1 hour of the desired destination MCBT time is reached and/or satisfactory sleep and daytime functioning is achieved (Fig. 13.4) In instances of a desired phase advance, 3 hours of dark are required prior to the MCBT and 3 hours of light are required immediately after the MCBT (depicted as “D” and “L”, respectively, on Figs. 13.2 and 13.4), as predicted by the light phase response curve (PRC) (see Fig. 13.3). If attempting to delay circadian rhythms, the opposite light/dark patterns are sought, as depicted in Figure 13.4. As flight/layover patterns will likely not allow for strict adherence to these measures, they represent idealized scenarios for which one should strive.
Requirements for darkness can be accomplished with sleep, protective eyewear, or by remaining in a dark room. Light requirements can be met with outdoor exposure, with a commercial light box, or with a separate apparatus (e.g., goggles or visors), many of which are sufficiently portable so as to be suitable for travel. Light boxes are considered more effective in achieving phase shifts than alternative devices, however, and widely available blue-light equipment may confer greater benefit than previously realized (22,26,27).
Guidelines regarding preflight circadian advances have also been developed in anticipation of eastward travel (12,18,28). The goal of such an intervention is to reduce the risk of antidromic re-entrainment and to hasten circadian adaptation and dissipation of jet lag symptoms upon arrival at the travel destination. In this 3-day protocol, the sleep/wake schedule is advanced by 1 hour each day, and subjects are exposed to intermittent bright light for 3.5 hours upon awakening (mean intensity ∽5000 lux1), administered as four 30-minute pulses, separated by 30 minutes in ordinary room light. Light administered in this fashion was found to be as effective as continuous bright light in a previous study (18), which has important practical implications. A synergistic response can be achieved by using concomitant melatonin (at a recommended chronobiotic dose of 0.5 mg), starting at 5 hours prior to habitual (home-based) bedtime (timed to achieve phase advances; see Fig. 13.3), with 1-hour incrementally earlier administration times, for resultant circadian advances of approximately 1-hour daily (compared with placebo) (25,28). Importantly, standardized assessments have revealed that this is not met with production of jet lag prior to departure, and can align the MCBT such that light exposure will properly facilitate completion of re-entrainment upon arrival to the travel destination (12,25). The same processes can be performed in preparation for a return flight, either predeparture or upon arrival. No pretravel guidelines have been published in anticipation of circadian delays (westward flights), and would be much more difficult to accomplish for those with inflexible work hours.
Nocturnal Medication Administration for Jet Lag
Melatonin.
Numerous field studies involving nightly dosing of melatonin (2-8 mg) have been conducted and, with few exceptions (29,30) have demonstrated improved quality of sleep (31, 32 and 33) and alleviation of daytime symptoms of jet lag (17,30,32, 33, 34, 35 and 36). Immediate-release preparations appear to be more effective than slow-release formulations (33). Although the majority of studies looked exclusively at adaptation to eastward travel (31, 32 and 33,35,36), one investigated the effects of melatonin on westward travel (30) and another assessed its effects during both departure and return trips that traversed 11 time zones (34).
Among the positive studies that involved preflight dosing, melatonin was scheduled for up to 3 days prior to departure (and, in two instances, en route [32,34]), at clock hours corresponding to the nocturnal sleep period at the travel destination (consistent times daily), and then for a subsequent 3 to 4 days between 22:00 and 00:00 destination time (i.e., q.h.s.) (32, 33, 34, 35 and 36). Several studies further simplified this regimen such that subjects took nocturnal melatonin only upon arrival at the travel destination, either for eastward (31,33) or westward travel (30).
Only two studies were reviewed that allowed for direct comparison of regimens, either for different directions of travel (34), or with different schedules (30). In the initial study, subjects received equivalently timed and dosed melatonin in preparation for departure and return trips that traversed 11 time zones (34). During the 3 predeparture days, subjects took melatonin (5 mg) between 10:00 and 12:00, followed by 3 nights of dosing at the travel destination between 22:00 and 00:00, all of which would tend to favor phase delays (see Fig. 13.3) which, given the number of time zones crossed, would be more readily achieved than phase advances in both directions of travel (see Fig. 13.4). If realignment indeed occurred in this direction, it would represent one possible explanation as to why jet lag symptoms were more severe with the westward return, as the duration to circadian realignment would be greater (34). Nevertheless, melatonin exhibited superiority to placebo in both directions of travel (34).
The study involving solely westward travel was the only one identified (among those with positive findings) that allowed for comparisons between those that received predeparture melatonin (3 days at 5-mg doses, taken between 07:00 and 08:00), in conjunction with 5 days of nightly postarrival dosing, to a regimen that began only upon arrival (30). Significantly better jet lag outcomes were found in the latter group, which might be viewed as unexpected, since both the pre- and postdeparture regimens would appear to favor phase delays (see Fig. 13.3). As physiologic circadian markers were not utilized in any of the aforementioned studies, however, it is impossible to ferret out the relative hypnotic and chronobiotic effects of the administered melatonin. Although speculative, it is possible that the dose of melatonin administered in the aforementioned predeparture group (and in other studies) was sufficiently high so as to exert an undesired hypnotic effect, thereby confounding the results. The chronobiotic properties of melatonin are thought to be optimal at doses of 0.5 mg or less (37).
Benzodiazepine Receptor Agonists.
The use of standard hypnotics to facilitate sleep during periods of circadian realignment would appear to be commonplace, but has been infrequently studied (17). Upon reviewing the data involving the newer generation benzodiazepine receptor agonists (three studies involving zolpidem [32,38,39], two involving zopiclone2 [31,40], consistently favorable subjective [32,38] and/or objective [31,39,40] results were seen with respect to counteracting jet lag-induced insomnia (for both eastward and westward travel), but data are less clear with respect to daytime symptoms, and have been reported as favorable (32), equivocal (40), or were not assessed (31,38,39). Nevertheless, in a study that assessed zolpidem (10 mg) in the setting of a simulated 8-hour eastward time-shift, use of the medication was found to limit the rebound secretion of thyroid stimulating hormone typically recorded during sudden phase advances, suggesting that it may have an independent circadian effect (39).
In two studies that compared these standard hypnotics to oral melatonin, one found that zopiclone (5 mg) and melatonin (2 mg) were equally beneficial with respect to sleep parameters (other jet lag symptoms were not assessed) (31). In the other investigation, zolpidem (10 mg) was found to be superior to melatonin (5 mg) with respect to both sleep and other jet lag symptoms, and the combined treatment group (zolpidem plus melatonin) demonstrated no benefit over zolpidem alone (32). Importantly, however, adverse events were recorded at a higher rate in those receiving zolpidem and included nausea, vomiting, and confusion (32). Although not deemed to be serious in nature, 14 subjects (10%) withdrew from the study.
Stimulants
Caffeine is presumably the first-line “medication” utilized to combat jet-lag induced sleepiness, but only two controlled field studies are available to assess its efficacy. Both studies utilized slow-release preparations at a dosage of 300 mg. In the first, following an eastward flight traversing seven time zones, subjects took the pill at 08:00 destination time for a period of 5 days (41). Urine and saliva samples were tested serially to determine the degree of circadian re-entrainment. Although alertness and other jet lag symptoms were curiously not assessed, circadian rhythms (as determined by salivary cortisol levels) were re-entrained at a more rapid rate than placebo, and to a degree comparable to that achieved by exogenous melatonin. In a follow-up study by the same group, those receiving
caffeine demonstrated an objective decrease in sleepiness compared with the melatonin and placebo group (assessed by multiple sleep latency tests), but intergroup subjective differences were not identified (42). Furthermore, those receiving caffeine demonstrated a significant increase in both objective and subjective nocturnal sleep complaints.
caffeine demonstrated an objective decrease in sleepiness compared with the melatonin and placebo group (assessed by multiple sleep latency tests), but intergroup subjective differences were not identified (42). Furthermore, those receiving caffeine demonstrated a significant increase in both objective and subjective nocturnal sleep complaints.
SHIFT WORK SLEEP DISORDER
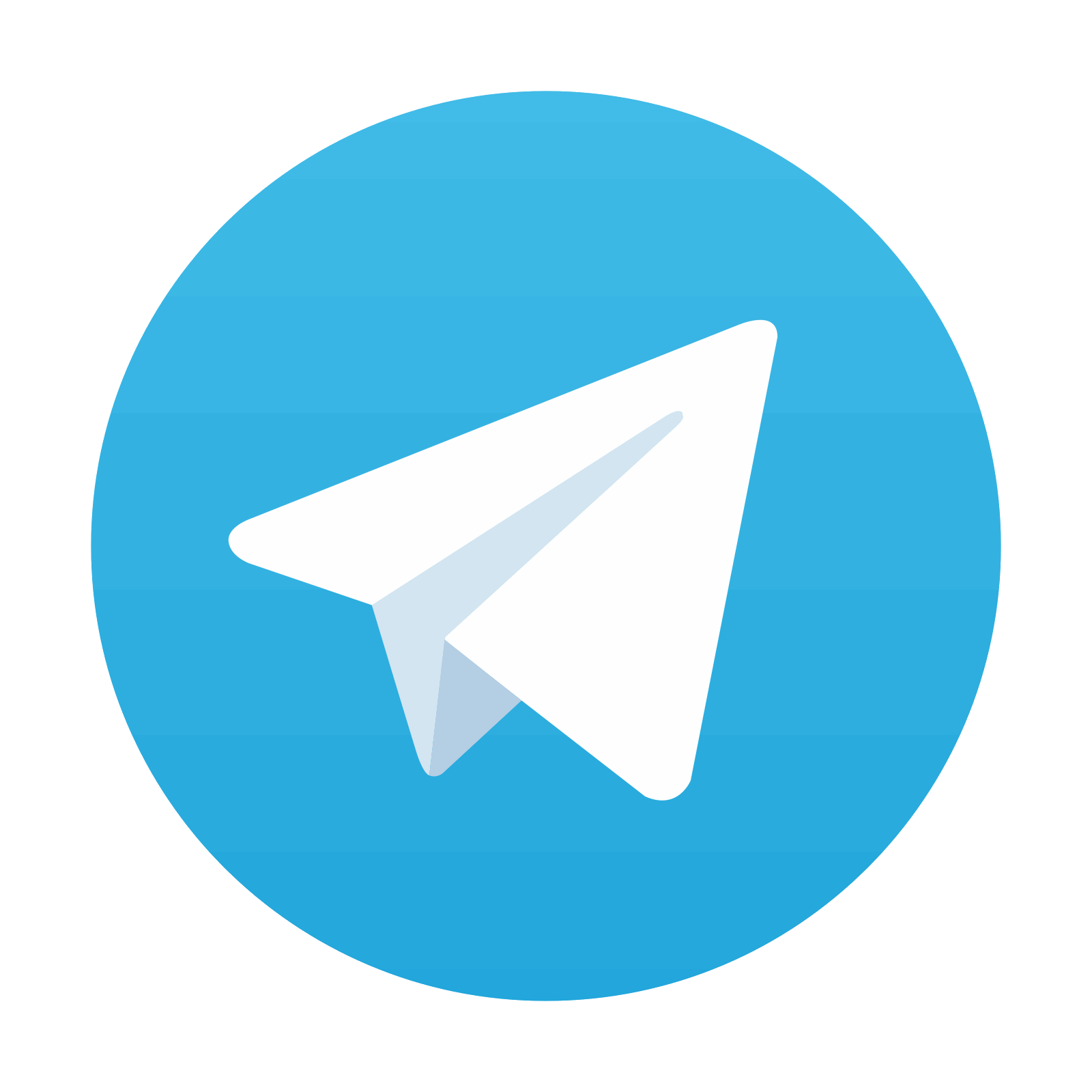
Stay updated, free articles. Join our Telegram channel

Full access? Get Clinical Tree
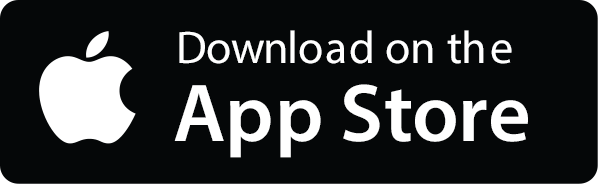
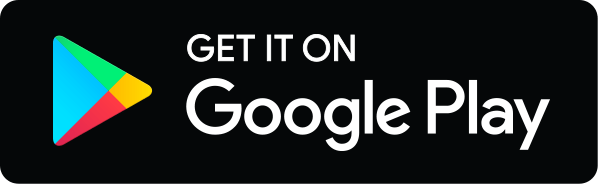