, Germaine Cornelissen2 and Franz Halberg2
(1)
Department of Chronomics & Gerontology, Tokyo Women’s Medical University Medical Center East, Arakawa-ku, Tokyo, Japan
(2)
Halberg Chronobiology Center, University of Minnesota, Minneapolis, MN, USA
Abstract
This chapter sketches some of the highlights of chronobiology. In a nutshell, considering time as the fourth dimension in biology amounts to changing variability from foe to friend. Akin to the splitting of the “indivisible” atom, chronobiology indeed splits the otherwise neglected seemingly indivisible range into rhythms and other predictable changes, such as trends and chaotic behavior.
Keywords
Time structuresCosinor methodEnvironmental geophysical cyclesCircadiansExtracircadian oscillations4.1 Introduction
While counting eosinophils in mouse blood, Franz Halberg was faced with confusing variability [1], Fig. 4.1. Large changes observed in several kinds of blood cell counts persisted when additional precautions were taken not to disturb the animals. He also found large variations in the content of glycogen in liver and in the number of cells in the state of division in the liver and other tissues. After collecting data at different times and pursuing the patterns of change as a function of time, predictable about-24-hour cycles became apparent. Some of these periodicities had large amplitudes and were highly reproducible. Relatively simple statistical methods applied to the data brought about quantification of the underlying patterns, which were to be known as circadian rhythms [2]. The “circa” in “circadian” indicates that the variations recurred “about” every 24 hours and the prefix conveys their statistical rather than purely deterministic causality. By noting that rhythms had periods near (“circa”) 24 hours as they recurred in similar (“circa”) but not identical sequences and at similar (“circa”) intervals, which differed statistically from their precise environmental and societal counterpart, the partly endogenous nature of circadian rhythms is recognized.


Fig. 4.1
Confusing variability in eosinophil counts partly resolved by large-amplitude circadian rhythm
Many more periodic changes characterize biological variables, which coexist with trends as a function of development, age, and transition from health to disease. Intermodulations among these different components broadened the scope of time structures (chronomes) beyond the circadian system. “Chronome” is derived from “chronos” (time), “nomos” (rule), and “chromosome” to describe the critical lawfulness of biologic structures in time. Mapping the rhythmic structure not only of eosinophil counts but also of the activity of the body as a whole, and its resistance to various stimuli, of mitoses in different organs and tissues, and of different liver functions related to different stages of the cell cycle led to applications to cancer treatment and to the fields of chronopharmacology and chronotherapy, based on the demonstration of the hours of changing resistance to a variety of stimuli [3–6].
As the emerging discipline of chronobiology developed and the ubiquity of circadian rhythms became evident, statistical methods for rhythm detection and quantification of rhythm characteristics with an estimate of uncertainty were devised that could handle non-equidistant data series. The cosinor method Franz Halberg introduced [7] was to play a critical role in providing a solid statistical foundation for the new discipline.
As Franz proceeded with his study of blood eosinophils, mice were kept under different experimental conditions, using different lighting regimens and feeding schedules. Reversing the regimen of 12 hours of light alternating with 12 hours of darkness, Franz found that the timing of rhythms along the 24-hour scale could be moved to any desirable location in time by manipulating the lighting regimen [8], thereby clarifying the role of the environment as a synchronizer of circadian rhythms, Fig. 4.2. Meal timing was also found to affect the timing of circadian rhythms [9].


Fig. 4.2
The environment serves as a synchronizer of circadian rhythms. Inverting the LD12:12 lighting regimen is associated with a 12-hour shift in the circadian acrophase
Environmental geophysical cycles, such as the astronomic day/night cycle, the phases of the moon, the tides, and the seasons, may induce periodic responses in certain species, but in many cases, they rather serve as synchronizers for partly endogenous rhythms [10]. The influence and importance of each of these synchronizers vary among species, depending, for instance, on the degree of exposure to one or the other of these geophysical cycles. An environmentally synchronized endogenous rhythm usually does not undergo an immediate abrupt phase shift of its synchronizer, but rather does so progressively over a number of transient cycles. The time it takes for the phase of a rhythm to adjust to the new environmental schedule depends upon the organism involved, the relative strength of the dominant synchronizer, the extent of the shift in synchronizer phase, the direction of shift (i.e., whether it is a phase advance or a phase delay), and the interaction with other environmental synchronizers (for instance, the lighting and feeding schedules). The rate of phase adjustment also differs among different variables [10].
In order to understand the mechanism through which the alternation of light and darkness affected circadian rhythms, marker variables such as rectal temperature were monitored longitudinally around the clock in mice born anophthalmic, in blinded mice, and in sham-operated control mice. These studies by Franz Halberg [11] led to the discovery of free-running rhythms, established on a rigorous statistical basis, the circadian rhythm persisting with a period differing slightly but statistically significantly from 24 hours, and also differing among some of the mice, Fig. 4.3. This finding provided a key element to the development of chronobiology as it indicated that rhythms were not an immediate response to the cyclic changes in the environment, but rather a fundamental internal property of organisms. It also suggested that the eyes served as the transducer and pointed to the hypothalamus as an important organ involved in the internal coordination of circadian rhythms [12–14].


Fig. 4.3
The circadian rhythm persists under constant environmental conditions, assuming a period close to but statistically significantly different from exactly 24 hour
The subsequent discovery of a molecular mechanism underlying circadian rhythms [15, 16] confirmed their partly endogenous nature. Current knowledge considers the suprachiasmatic nuclei in the hypothalamus to play a critical role as “master oscillator” or pacemaker [17]. The view originally held that all circadian rhythms are obliterated after bilateral suprachiasmatic enucleation [18] had to be revised [19], however, and it is now recognized that clock genes are found in almost every cell [20]. Early confirmed work by Franz Halberg [9, 21] had shown the critical role of the adrenal cortex in coordinating circadian rhythms in blood eosinophils, but not in serum iron [22]. Evidence is available, however, showing that some circadian rhythms can persist in one or the other variable after removal of either the suprachiasmatic nuclei [23], and even of the brain as a whole [24], or of both the adrenals [25].
4.2 Rhythms of Different Orders
The lawfulness of the circadian variation, determined by the application of statistical methods, became apparent from the ubiquity of prominent circadian rhythms at different levels of organization. A series of experiments in Franz Halberg’s laboratory investigated the effect of a single physical stimulus under rigorously standardized laboratory conditions. Whether the stimulus was audiogenic [3] or due to exposure to an endotoxin [26], or to a drug such as ouabain [27], or to whole-body irradiation [5], predictable changes were found as a function of the circadian stage at which the stimulus was applied, the timing of these susceptibility-resistance rhythms differing from one kind of stimulus to another. This work led to the concept of the hours of changing resistance, maps being prepared to indicate the times of overall largest response by the organism to a fixed stimulus applied at different rhythm stages. Prominent susceptibility rhythms were documented in the experimental laboratory, notably in relation to anticancer drugs [28, 29], Fig. 4.4.


Fig. 4.4
Acrophase chart illustrating the circadian susceptibility-resistance cycle to different anticancer drugs tested in the experimental laboratory
Using staggered lighting regimens, it was possible to examine how neuroendocrine and peripheral systems intermodulate by means of feedsidewards mechanisms [14]. Feedsidewards are interactions among three or more periodic entities. One example is the modulation by the pineal of pituitary-adrenal interactions [30]. Periodically recurring sequences of stimulation, no effect, and inhibition by a modulator (in this case, the pineal) upon the interaction of an actor (in this case, pituitary ACTH) with a reactor (in this case, the adrenal) are observed, Fig. 4.5. Chronomodulation (feedsidewards) was also observed for the effect of ACTH upon metaphyseal bone DNA labeling in the rat [31]. Feedsidewards are thought to coordinate the network of spontaneous, reactive, and modulatory rhythms into a broad multifrequency time structure, the chronome.


Fig. 4.5
Example of feedsideward-pineal homogenate modifies the effect of ACTH-1-17 on corticosterone production by the adrenals
The chronome, as the backbone of life itself, thus recognizes that physiological mechanisms follow rhythmic changes of several frequencies, their response to a fixed stimulus changing predictably as a function of when it is applied. This view, slowly being embraced [32], is at variance with the concept of homeostasis, defined as a regulation for a putative constancy, presuming that the organism responds in a similar way to a given stimulus at all times. Partly endogenous rhythms include a preparatory feature that allows organisms to anticipate changes in demand to occur at different rhythm stages. The chronobiologic view also yields a positive definition of health, by contrast to seeing health merely as the absence of disease. The characteristics of rhythms and trends and the coordination resulting from relations within chronomes provide a way to quantify health. Chronome alterations, in turn, provide harbingers of increased risk of developing disease, prompting the early institution of prophylactic intervention for a truly primary prevention, while rhythm characteristics also serve for secondary prevention and for the optimization of timing treatment administration when treatment is needed.
4.3 Circadian and Extracircadian Oscillations in Heart Rate
Beyond circadian rhythms are many other oscillations of different frequencies. Corresponding periods range from fractions of seconds in single neurons to seconds in the cardiac and respiratory cycles and a few hours in certain endocrine functions in the ultradian domain. In the infradian domain, about-weekly (circaseptan) and half-weekly (circasemiseptan) changes are also ubiquitous, as are about-monthly (circatrigintan) and circannual variations. Other cycles with periods of about 5 and 16 months and about 5, 10.5, 21, and 50 years and even lower-frequency changes have also been found in biology. Because their environmental counterparts are less stable, additional methodologic tools are needed to study them that can handle nonstationarities. These components will be reviewed in more detail in the chronomics section.
4.3.1 The Cardiac Cycle
The electrocardiogram of a clinically healthy subject reveals a relative complex waveform that repeats itself with some regularity. This basic cycle is composed of several waves, corresponding to the conduction of electrical signals allowing the heart to pump blood into the circulation. An automaticity, outside the body, of the heart as a whole, deprived of its whip and break (its sympathetic and vagal efferents), was found by Aztec priests who sometimes observed beats of the organ after it was ripped out of the body for divination [33]. Their finding has been carried from the single organ to the single cell [34].
4.3.2 Respiratory Sinus Arrhythmia
Respiratory sinus arrhythmia is a naturally occurring cardiopulmonary reflex, the heart rate varying with the phase of the respiratory cycle. Inspiration increases and expiration decreases the rate via vagally mediated reflexes [35]. Expiration favors left ventricular filling by increasing the return to the left heart of blood that was stored in the lungs during inspiration. Respiratory sinus arrhythmia is very noticeable in infants and in children [36]. During voluntary cardiorespiratory synchronization, it becomes less prominent with advancing age. It is estimated to decrease by about 10 % for each decade [37]. Spectral analyses of heart rate variability at rest indicate that sinus arrhythmia declines less with age than lower (vasomotor) frequencies, suggesting that the decline in heart rate variability as a function of age may not be predominantly associated with a decline in frequencies related to respiration [38]. A central cholinergic receptor is thought to be involved in the respiratory modulation of heart rate [39]. Influence of the arterial baroreceptors has been suggested in the generation of respiratory sinus arrhythmia in conscious humans [40]. Heritability of respiratory sinus arrhythmia has been estimated to range from 28 % to 43 % [41].
4.3.3 REM/NREM Cycle
Relying on sleep polygrams, the sympatho-vagal balance, quantified by the spectral power around 10.5 s and 3.6 s (LF/HF), was found to decrease the more so the more synchronized sleep was, whereas this ratio was increased during REM sleep, suggesting a sympathetic predominance during REM sleep [42]. Increases in HF and decreases in LF spectral power of heart rate variability across NREM sleep stages and opposite changes in REM sleep and wake were corroborated in other studies [43, 44]. About-90-min components in heart rate variability observed in our own studies [45, 46] are likely related to the sleep-wake (REM/NREM) cycle.
4.3.4 Other Ultradian Components
Longitudinal recordings of neonatal heart rate during the first weeks of life have indicated the presence of ultradian changes likely related to the feeding schedule. Babies monitored in Florence (Italy), who were fed every 3 hours, have a prominent spectral peak at a frequency of one cycle in 3 hours. A prominent spectral peak at a frequency of one cycle in 4 hours is found in heart rate records from babies in Germany fed every 4 hours [47]. In adults, some neuropeptides can have more prominent ultradian than circadian changes. This is, for instance, the case of endothelin-1, which undergoes a pronounced about-8-hour variation [48–50], Fig. 4.6. An 8-hour rhythmic variation was also found to characterize the urinary excretion of norepinephrine in women with Bartter’s syndrome and in clinically healthy volunteers [51].


Fig. 4.6
An 8-hour variation characterizes circulatory endothelin-1 (ET-1) in healthy adults, whereas cortisol, determined concomitantly, undergoes a large-amplitude circadian rhythm
4.3.5 Circadians
Day-night differences not fully accounted for by the sleep-wake cycle are already demonstrable in early extrauterine life [52]. During the first week of life, a circadian rhythm is detected on a population basis, peaking around 01:00, in almost antiphase with the mother’s circadian rhythm [53]. Changes in heart rate within a single day usually exceed the range of change in this variable as a function of age in adulthood (between 20 and 60 years of age) [54]. Under habitual conditions, heart rate usually assumes higher values during the daily active span and lower values during the daily rest span. Following a change in schedule, this pattern adjusts gradually rather than immediately to the new routine [55]. The circadian rhythm in heart rate persists during complete bedrest for 36 hours and a 4-hourly hypocaloric diet, indicating that it is not fully dependent upon the rest/activity cycle [56]. In the absence of time clues, the persistence of a circadian rhythm with a period near 24 hours but differing from exactly 24 hours with statistical significance (free-running) is another indirect line of evidence documenting a partly endogenous component. A free-running, presumably partly endogenous circadian heart rate component has been demonstrated to persist under conditions of isolation from the usual physical as well as societal environment [57, 58].
Non-chronobiologic studies on twins accounted by heredity for 54 % of the interindividual variation in ventricular rate and for 34 % in the case of atrial depolarization [59]. A statistically significant heritability of 60 % or more has also been reported for resting heart rate [60] as well as for the activity of heart rate during mental activities [61]. A genetic background of the circadian rhythm in human heart rate was proposed by Barcal et al. [62]. Heritability not only of the MESOR but also of the circadian amplitude was confirmed in studies of twins reared apart [63]. Differences in 24-hour amplitude of heart rate are much smaller within twin pairs than among twin pairs. Intra-class correlation analyses on results from 35 monozygotic and 22 dizygotic twin pairs reared apart document a genetic basis for the circadian rhythm of human heart rate [64]. After adjusting for gender and age, the difference in intra-class correlation coefficient between monozygotic and dizygotic twin pairs remains statistically significant, Fig. 4.7.


Fig. 4.7
Heritability of the circadian amplitude of heart rate examined in monozygotic twins reared apart
4.3.6 Circaseptans and Circasemiseptans
The biologic week is also ubiquitous and partly endogenous, being amenable to synchronization by social schedules, as evidenced from several lines of research. Free-running was originally shown by Franz Halberg in a 15-year-long record of daily determinations of urinary excretion of 17-ketosteroids. Whereas urine volume remained synchronized for 7 days, 17-KS started to free-run after 12 years, in association with self-administration of testosterone [65], Fig. 4.8. Free-running of the about-7-day and about-3.5-day components has also been observed in isolation studies [66, 67]. Amplification, if not induction of circaseptans after a single stimulus, is another source of indirect evidence for a partly endogenous biologic week. The stimulus can be as mild as balneotherapy [68] or as severe as organ transplantation [69]. Birth is such a single stimulus to which babies respond with a prominent circaseptan component in blood pressure and heart rate [70–73]. When babies are monitored around the clock for several weeks, a circaseptan component is detected with statistical significance on an individualized basis, the circaseptan period, estimated by nonlinear least squares, differing from precisely 7 days as well as among individual babies [72]. In this study, when results are pooled from a large group of babies, analyses of variance indicate that the circaseptan component is synchronized by the time of birth (developmental age) rather than by the societal schedule. In a small group of neonatal twins, the circaseptan period was more similar between twins in a pair than among different pairs of twins, as assessed by an intra-class correlation coefficient [74], Fig. 4.9. A circaseptan response to a single stimulus (release into continuous darkness from an LD12:12 regimen) was also observed in a unicell [75]. Additional indirect evidence stems from several studies in Acetabularia [76], face flies [77], and springtails [78], where the response variable (growth or lifespan) followed an about-7-day pattern in relation to the interval between successive shifts they were submitted to [for review, see 79]. Circaseptans and circasemiseptans have been particularly prominent in relation to growth, development, and immunology [80].



Fig. 4.8
Free-running of the weekly component in the urinary excretion of 17-ketosteroids, but not of urine volume of a clinically healthy man

Fig. 4.9
Heritability of the circaseptan component of heart rate examined in neonatal twins
While circadians are much more prominent in adulthood, circaseptans and circasemiseptans usually remain demonstrable, with a peak usually around mid-week [81–83]. The relative prominence of circaseptans and circasemiseptans as compared to circadians varies as a function of age, regaining importance in the elderly [84, 85]. Circaseptans are also more pronounced in the presence of a depressed mood [86, 87], as it is also the case for negative affect, as compared to positive affect [88]. The merits of chronotherapy along the scale of the week, achieved by optimizing the circaseptan schedule of treatment administration, have already been demonstrated for several drugs [89–91], while still awaiting clinical applications.
4.3.7 Circatrigintans
In one isolation study, the circaseptan component of heart rate, which was free-running, did not immediately resynchronize upon return to a societal routine, while the circadian system quickly adjusted to the 24-hour schedule. The circaseptan system failed to do so for weeks, until menstruation was re-induced by hormones [58]. Neurohormonal or other effects of menstruation may be a condition for synchronization of a built-in circaseptan component with societal schedules [58]. About-monthly cycles are observed not only in relation to menstruation. By 1657, Santorio’s aphorisms comment on changes in body weight of one or two pounds recurring about once a month in a mature healthy man [92]. The unusual case of a man who bled from his thumb about once a month is recorded [93], as is a purpura of the calf recurring for 6 years at intervals of about 4 weeks in a 60-year-old man with Morbus maculosus Werlhofii [94]. Circatrigintans characterize the frequency of neutrophil leukocytes with androgen-induced nuclear appendages [95]. In a variety of life forms, variables related not only to the reproductive tract but also to other organ systems of individuals of both genders show overt cycles in the range of about 30 days [96]. The about-monthly component of breast surface temperature discriminates between women with breast cancer who will die or survive [97]. The incidence of tachyarrhythmia has also been reported to vary as a function of lunar stage [98]. Differential effects of space and/or terrestrial weather with respect to suicide and other deaths associated with the nervous and sensory systems vs. death from cardiac or respiratory disease as well as overall death by differences in the phase of a common 27-day cycle characterizing these mortality patterns [99].
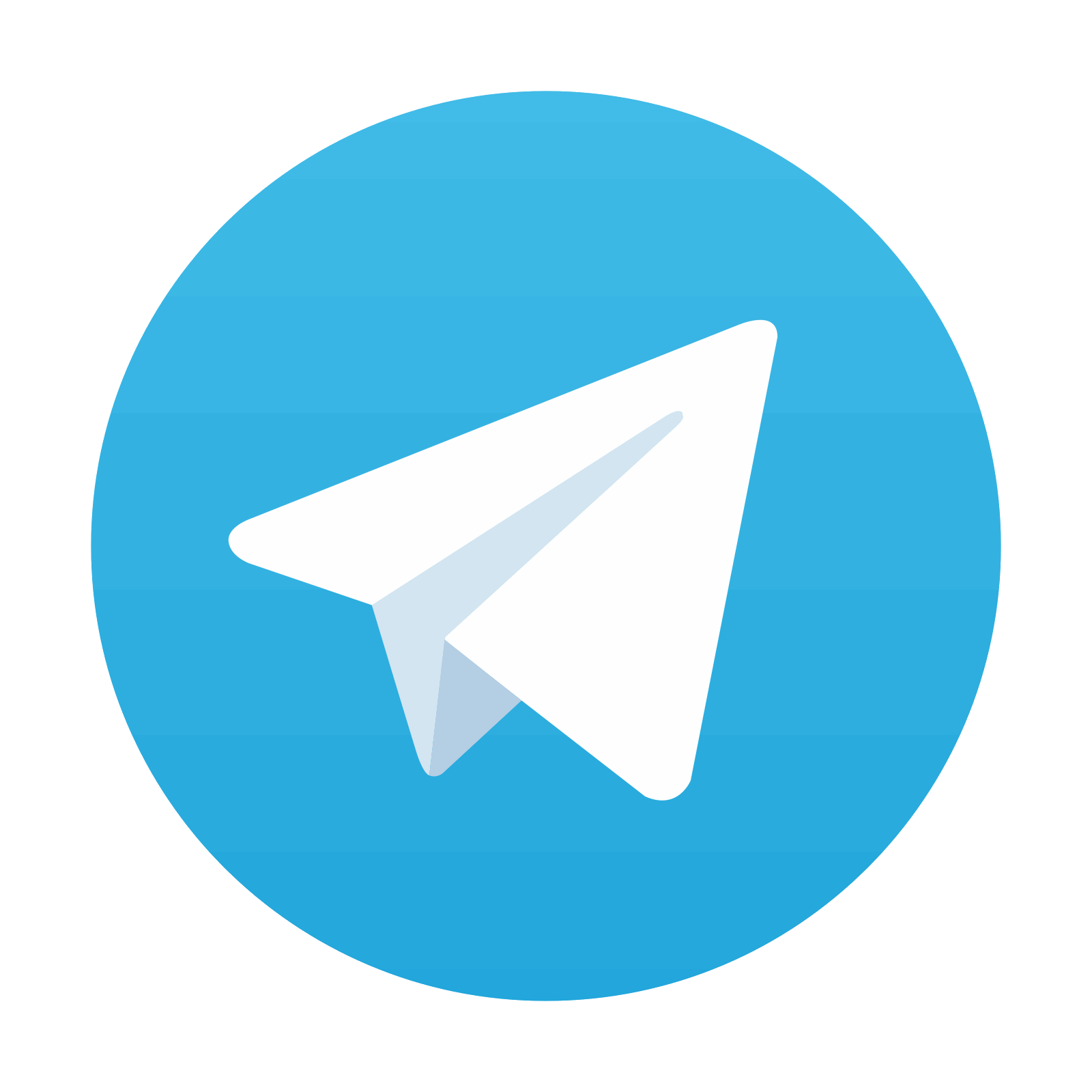
Stay updated, free articles. Join our Telegram channel

Full access? Get Clinical Tree
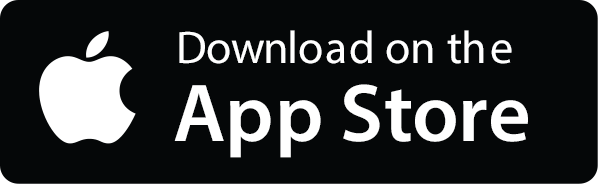
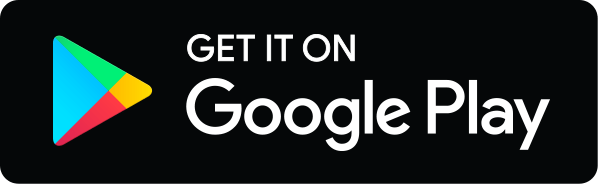