, Germaine Cornelissen2 and Franz Halberg2
(1)
Department of Chronomics & Gerontology, Tokyo Women’s Medical University Medical Center East, Arakawa-ku, Tokyo, Japan
(2)
Halberg Chronobiology Center, University of Minnesota, Minneapolis, MN, USA
Abstract
Life did not emerge spontaneously. Life was created on this planet so that it “matches” with the natural environment and was further evolved through the physical/chemical processes which reflect the state of the planet, including the atmosphere and water, as well as the magnetic field. The present state of the natural environment of the earth has been accomplished so as to balance with the conditions of the solar system and the rest of the universe.
We must realize that what we can see by our human eyes is limited in scope, wavelength, etc. That we cannot see some things does not mean at all that they do not exist in reality. There is a lot we must learn from nature. Life on the earth’s surface is, directly or indirectly, influenced or, more explicitly, controlled by how this planet was created and how the present environment has evolved. This is one of the messages the aurora in the polar sky is sending us.
From Professor Yosuke Kamide’s words, “Our life is protected by the Earth’s atmosphere and magnetic field: what aurora research tells us” (Biomed Pharmacother 55:21s–4s, 2001).


Humans live on the planet in space according to the law of nature
Keywords
ChronoastrobiologyGeomagnetic activityMagnetoreceptionHeart rate variabilityCardiovascular outcomes13.1 Introduction
The term “chronoastrobiology” is derived from “chronome” (time structure) and “astrobiology” (reciprocal implications of biology and space studies). It was the title of a proposal for the development of a chronoastrobiology research initiative on February 8, 1999, by Franz Halberg, Germaine Cornelissen, and Othild Schwartzkopff of the University of Minnesota in Minneapolis; by Daniel C Holley of San Jose State University, California; and, within the US National Aeronautics and Space Administration (NASA), by Charles M Winget, then of the Ames Research Center, Moffett Field, California [1].
This proposal included four specific goals that remain as important today as they were in 1999:
First, the longitudinal monitoring of psychophysiological variables to compare their time structure with that of space and terrestrial environmental variables. Because some periodic components in biology (such as the circadian rhythm) may be genetically anchored, they may more readily be synchronized and/or resonate with their counterparts in the environment. The sequences of integration of rhythms into the genome, possibly revealed by their relative prominence in ontogeny and phylogeny, may shed light on the sites and time course of life on earth.
Second, data analyses by complementary approaches for examining associations between biological and environmental chronomes. The extent of concordance between results as a function of time and of their reproducibility across several geographic locations in a glocal approach should lead to a better understanding of environmental influences on biota.
Third, the mapping of cycles characterizing important physiological functions in humans and other selected species at different stages of development. Such a comparative physiological approach can be followed to examine the extent to which the evolution of chronomes may lead to testable hypotheses concerning the origins of life.
Fourth, as a dividend from the establishment of reference databases resulting from the above basic work, to identify disease risk syndromes. A better understanding of underlying triggers and the development of countermeasures to lower risk by timely and timed intervention (chronotherapy) aim as much at primary as it does at secondary prevention.
From early in Franz Halberg’s career, the mapping of rhythms proved to be very useful as a way to defining health and learning about the origins of life. His work on the circadian cell cycle provides the best illustration of how the study of rhythms may shed light on internal as well as external evolution. Halberg deserves great credit for disproving the then prevailing dogma that the nucleic acid was the most constant feature of the body with his demonstration in the 1950s of a circadian periodic cell cycle [2–4]. Not only did he show that DNA and RNA were characterized by a robust circadian rhythm, Halberg also showed that RNA synthesis precedes that of DNA (Fig. 13.1), contrary to the dominating view of a linear biochemical sequence starting from DNA to RNA and protein [2–4]. Halberg’s finding of a lead in phase of the circadian rhythm in RNA formation vs. DNA formation was later reproduced for the case of RNA content, shown to precede DNA content in the unicell Euglena [5].


Fig. 13.1
Circadian rhythm in the formation of phospholipids, RNA, DNA, glycogen content, and mitoses in mouse liver. Note that RNA peaks before DNA (Adapted from Halberg F. The 24-hour scale: a time dimension of adaptive function organization. Perspect Biol Med. 1960;3:491–527. © Halberg Chronobiology Center)
With the qualification that RNA formation also concerns nondividing cells, the circadian mapping of nucleic acids raised the question of a temporal precedence of RNA vs. DNA. Halberg’s finding in growing mouse liver provided a hint that RNA may be more atavistic than DNA, decades prior to the chemical evidence suggesting that an RNA molecule could have existed that “naturally contained the sequence of information for its reproduction through reciprocal-base pairing and could also catalyze a synthesis of more like RNA strands” [6]. For this stage in the development of life, it was hypothesized that “RNA molecules and cofactors sufficed to carry out all the chemical reactions necessary for the first cellular structures” [6]. Along this line of thought, an RNA world may well have occurred before our DNA world. If the relative phases of their circadian rhythms reflect evolutionary changes, the precedence of RNA synthesis before DNA formation in the given cell cycle [2] can be viewed as an early hint of the chain of events in the course of evolution [7].
Along a similar line of thought, it is tempting to speculate about the role of multiseptans throughout evolution. Multiseptans are components with periods of about 7 days and multiples or submultiples thereof, among which the half week and month are also ubiquitous. A biological week is indeed built into bacteria and unicellular organisms, perhaps another chronobiologic contribution to phylogeny [8–18]. As to ontogeny, the about-weekly change can be more prominent than the about-daily change in human neonatal blood pressure and heart rate [13, 19–28], as it is in the early postnatal life of other species [29–31]. An about-7-day pattern was even observed in DNA labeling under daily STH stimulation in mouse tissues [32], and multiseptans were found to modulate circadian cell division and cell settling patterns in Euglena [33]. As in the case of circadians, circaseptan counterparts are present in the environment. Abbot reported an about-6.65-day cycle in the weather [34]. A near match of the week is also found in the index of geomagnetic disturbance Kp [35–38], likely the fourth harmonic of the about-27-day solar rotation period. Notably, if it developed at the bottom of an ocean, in the absence of sunlight, life could have latched onto nonphotic cycles such as the multiseptans when it faced tasks such as those in immunology and repair, which could not be accomplished in a day, but could be accomplished within a week, a hint of an internal evolution complementary to Darwinian evolution [5, 36]. As the period of the biological week differs from precisely 7 days in human isolation, as does the period of Kp [39–44], it is unlikely to be purely anthropogenic, even though human-made contributions to the weather may be overwhelming [45].
Interestingly, an effect of a magnetic storm at the cellular level and possibly also a site of mammalian magnetoreception in the eye was reported [46]. Mitotic rhythms are particularly prominent as mapped in the urodele, mouse, and rat cornea. They were systematically studied by Lawrence E. Scheving in eight separate 24-hour profiles on rats kept in light and darkness alternating at 12-hour intervals (Fig. 13.2) [47]. One of the eight surveys happened to coincide with a magnetic storm. As seen from Fig. 13.3, the circadian amplitude of corneal mitosis on the day of the magnetic storm was below the lower 5 % prediction limit derived from the circadian amplitudes of the mitotic rhythm in corneal mitoses on the seven quiet days [46].



Fig. 13.2
Circadian rhythm in corneal mitoses in rats assessed in eight different studies. One of these studies was performed on a day of a magnetic storm. Quiet conditions prevailed on days of the other seven studies (© Halberg Chronobiology Center)

Fig. 13.3
On the day of a magnetic storm, the circadian amplitude of rats’ corneal mitotic activity was reduced, by comparison with results obtained during quiet days (© Halberg Chronobiology Center)
Work by Faraone who studied seemingly spontaneous and mutagen-induced sectoring in bacteria may also be pertinent. He showed that natural physical influences from the remote environment may be involved in modulating genetic microbial change [48–50]. Any physical environmental associations with the incidence of colonies with one or more differentiated sectors (CSD) were examined for air bacteria and Staphylococcus aureus. Cross-spectral coherence was found between air bacteria and the equatorial index of geomagnetic disturbance (Dst) around one cycle in 18.6 days (P = 0.005) and between S. aureus and both Dst and Kp, the planetary index of geomagnetic disturbance, around one cycle in 161.5 days or about 1 week (P < 0.05) [50].
Herein, investigations of environmental influences on biota rely on methods other than correlation analysis, an approach too often applied inappropriately. Indeed, the correlation coefficient measures the strength of a relation between two variables, but not the agreement between them. As correlation depends on the range of the true quantity in the sample, if that range is wide, the correlation is greater than when it is narrow. Instead, reciprocal cycles, that is, shared periodicities found in biology and its environment, constitute critical information underlying evolution and genetics, whether dealing with the weather and climate in earth’s atmosphere, or with the health of individuals and societies [51]. As reviewed below, there are matching cycles in us and around us. Some relate to heart rate and heart rate variability. Others relate to blood pressure and blood pressure variability. Yet, others deal with the incidence of adverse cardiovascular events, as well as with a host of other variables of broad biomedical interest.
13.2 Solar and Geomagnetic Activity and Heart Rate Variability
Decreased heart rate variability (HRV) has been associated with increased mortality after acute myocardial infarction [52] and after coronary artery bypass grafting surgery [53]. A one-standard deviation (SD) drop in the SD of total normal RR intervals was associated with a hazard ratio of 1.47 (95 % CI: 1.16, 1.86) in the Framingham Heart Study [54]. Together with increased sympathetic tone, it has been related to poor prognosis in several patient populations [55] and an increased risk of sudden cardiac death in patients with myocardial infarction, congestive heart failure, or hypertension [56]. A decreased nighttime HRV was reportedly a strong marker for the development of stroke in apparently healthy subjects [57]. In healthy young adults, reduced HRV indices were independently associated with increased CRP concentrations [58]. Understanding how the natural environment influences HRV is thus important, both on earth and in extraterrestrial space, where crews are spending ever extending journeys on the International Space Station.
In Stockholm, Sweden, the 24-hour SDs of hourly estimates of heart rate were obtained by Holter monitoring from 50 men who had had an acute myocardial infarction or had angina pectoris. Compared with the 24-hour SD of heart rate from 50 clinically healthy men of similar age, it was 20.5 % lower in the patients (P = 0.002) [59]. Not only is HRV decreased in the presence of ischemic heart disease, a low HRV is also a predictor of cardiovascular disease risk. Indeed, in Tokyo, Japan, the heart rate of 121 normotensive subjects and 176 treated hypertensive patients was measured around the clock by ambulatory monitoring for 2 days [60]. At the time of monitoring, all 297 participants were free of heart disease. The incidence of ischemic heart disease was recorded prospectively for 6 years. As compared to participants free of adverse outcome, patients who had developed coronary artery disease within the 6 years of follow-up have a 20.0 % lower HRV (P = 0.04). Results from these two studies were aligned with those of a retrospective analysis of archived data on all crews of the Soyuz spacecraft for 1990–1994. Based on the ECG records from 47 male and 2 female cosmonauts, the SD of heart rate was 30 % lower in cosmonauts examined during a magnetic storm, as compared to cosmonauts monitored on quiet days (P = 0.041) [61], suggesting that exposure to geomagnetic disturbances may increase cardiovascular disease risk (Fig. 13.4).


Fig. 13.4
HRV is decreased in patients with ischemic heart disease (left). A lower HRV is a predictor of coronary artery disease (center). By comparison to an about 20 % decrease in HRV in these two patient populations, HRV is about 30 % lower in healthy cosmonauts who are monitored during a magnetic storm as compared to those studied during magnetic quiet (© Halberg Chronobiology Center)
A reduced HRV on days of high magnetic activity vs. quiet days has also been documented in the longitudinal electrocardiographic (ECG) record of a clinically healthy man, both in terms of the coefficients of variation of R-R intervals and of the total spectral power [62]. In the frequency domain, the decrease in HRV was statistically significant in the very-low-frequency region (periods in the range of 6.5–333 s) but not in the low-frequency (periods of about 10.5 s) or high-frequency (periods of about 3.6 s) region, suggesting an underlying physiological mechanism other than the parasympathetic system as responsible for changes in HRV in response to magnetic activity [62, 63].
To follow up on these results, effects of geomagnetic disturbance on HRV were assessed in adults living at high latitude, in the auroral oval, where magnetic storms are more frequent and more intense than at lower latitudes. Nearly continuous, 7-day beat-to-beat ECG records were obtained between December 1998 and November 2000, on clinically healthy men (N = 15) and women (N = 4) from Finnmark College in Alta, Norway (69°56′ N, 23°22′ E). Their age ranged from 21 to 59 years. A geomagnetic record was obtained from the Auroral Observatory of the University of Tromsø, in Tromsø, Norway (69°39′ N, 18°56′ E), including 1-min data of total intensity (F, in nT), declination (D, angle between geographic and magnetic north, in degrees), inclination (I, angle between horizontal plane and magnetic direction, in degrees), horizontal intensity (H in nT), and vertical intensity (Z in nT). A decrease in HRV (P = 0.002) and an increase in the 24-hour average of heart rate (P = 0.020) were documented on days of high geomagnetic disturbance [64] (Fig. 13.5).


Fig. 13.5
HRV is decreased and heart rate is increased on magnetically disturbed days as compared to quiet days in clinically healthy subjects studied above the arctic circle
As expected, the decrease in spectral power was found primarily at frequencies lower than 0.04 Hz and was not statistically significant around one cycle in 3.6 s. The decrease in HRV was more pronounced in the VLF (21.9 % decrease, P < 0.001) than in the ULF (15.5 % decrease, P = 0.009) region of the spectrum [64–67]. This effect was more pronounced in spring and autumn, in the presence of a daily light-dark cycle, than in summer (continuous light) or in winter (continuous darkness) [64, 68]. This finding is clinically important because a reduction in the VLF range is associated with an increased risk of cardiac and dysrhythmic death [64]. Several mechanisms have been proposed for the VLF component, namely, thermoregulation [69], the renin-angiotensin-aldosterone system, and other humoral factors [70].
Investigation of 7-day ECG records from five clinically healthy young men living above the arctic circle compared HRV measures assessed over separate 24-hour spans among days of low, middle, and high geomagnetic activity, defined as days with values of the geomagnetic index ap < 7, 7–20, and 20–45, respectively. A graded response was observed, the extent of HRV decrease depending on the degree of geomagnetic activity. Specifically, as compared to days when ap < 7, TF was decreased by 18.1 % and 31.6 %, ULF by 18.1 % and 27.5 %, and VLF by 12.9 % and 28.6 % on days when 7 < ap < 20 and 20 < ap < 45, respectively [64, 71–73]. A graded decrease in HRV to geomagnetic activity suggests the existence of human magnetoreceptors. Phillips et al. [74] proposed a light-dependent magnetoreception mechanism and established a link between magnetic field sensitivity and the visual system in eastern red-spotted newts. The results suggest the operation of a light-darkness-alternation-influenced, if not light-dependent, magnetoreception mechanism in humans.
The existence of such a mechanism is supported by results from cross-spectral analyses. Group-averaged coherences at frequencies of 0.001–0.0001 Hz (0.25–5 hour) differed with statistical significance (P < 0.001) among the three lighting conditions, being on the average smaller during long days or long nights than during spring or autumn. Intermittent fields and components in the ultralow-frequency band of the electromagnetic field have been considered to be more bioeffective than the predominantly sinusoidal power line fields in the extremely low-frequency band. The ultralow-frequencies of the electromagnetic field are composed of six kinds of continuous pulsations, including Pc 1 (period range: 0.2–5 s), Pc 2 (5–10 s), Pc 3 (10–45 s), Pc 4 (45–150 s), Pc 5 (150–600 s), and Pc 6 (10 min–5 hours). The cross-spectral analysis results thus suggest that the magnetoreception mechanism may involve the Pc 6 geomagnetic pulsations [64].
Associations between geomagnetic storms and HRV were observed not only on the day of a storm but also for 2 days immediately following a geomagnetically disturbed day. Whereas changes in HR, NN interval, r–MSSD, and pNN50 were found to be statistically significant only during the day of the storm, changes in SDNNIDX (30 min) and in the power-law scaling of the power spectra remained statistically significant for 1 or 2 days following the day with a geomagnetic storm [64]. The 1/f slope was statistically significantly steeper not only on the day of the storm but also on the 2 days following a geomagnetic storm (P < 0.001), suggesting that geomagnetic disturbances have a lasting influence on the fractal scaling of HRV (Fig. 13.6).


Fig. 13.6
The power-law scaling of the power spectra of HRV remains statistically significant for 1 or 2 days following the day with a geomagnetic storm
A decrease in HRV and an increase in heart rate in association with solar activity, as gauged by Wolf numbers, are also observed in a 26-year longitudinal record of around-the-clock data collected mostly at 30-min intervals by a clinically healthy man (Figs. 13.7 and 13.8). Magnetic storms are usually more frequent at times of high solar activity. The data are modeled by the least-squares fit of cosine curves with periods of about 1.0 and 10.5 years. Nonlinearly, the periods and their 95 % confidence limits (CI) are estimated as 1.02 [95 %CI: 1.00, 1.04] and 10.60 [95 %CI: 9.21, 11.98] years for the monthly means and as 0.98 [95 %CI: 0.96, 1.00] and 10.44 [95 %CI: 8.85, 12.03] years for the monthly SDs of heart rate. In both cases, the about-11-year solar cycle length is included in the 95 % CI. An about-10.5-year component in the SD of heart rate had already been detected in the first 11 years of this record [63].



Fig. 13.7
Monthly standard deviations (SD) of heart rate (HR) measured around the clock mostly at 30-min intervals by a clinically healthy man for 26 years are characterized by a prominent about-10.5-year cycle, here shown with the circannual variation. At times of high solar activity, gauged by Wolf numbers (gray curve), the HR-SD is decreased (Data from Y Watanabe. © Halberg Chronobiology Center)

Fig. 13.8
Monthly averages of heart rate (HR) measured around the clock mostly at 30-min intervals by a clinically healthy man for 26 years are characterized by a prominent about-10.5-year cycle, here shown with the circannual variation. At times of high solar activity, gauged by Wolf numbers (gray curve), the HR is increased (Data linearly detrended from Y Watanabe. © Halberg Chronobiology Center)
The mapping of chronomes, and particularly of cycles shared between physiology and the environment, may thus prove to be a valuable approach to address the goals specified at the beginning of this chapter. For this approach to be successful, reference values from womb to tomb are essential, a project started by the International Chronome Ecology of HRV (ICEHRV) Working Group [64]. For this purpose, around-the-clock ambulatory ECG records have already been obtained from infants (25 days–3months of age), children (3–9 years of age), boys and girls (10–14 years of age), pubertal boys and girls (15–20 years of age), adults of both genders (2–92 years of age), and pregnant women in Japan. In addition, 7-day or longer ECG records were obtained from clinically healthy adults in different geographic locations. Similar records from patients with coronary artery disease and with dilated cardiomyopathy have already found marked differences in their time structure [65, 75, 76].
13.3 Solar and Geomagnetic Activity and Blood Pressure Variability
The influence of space weather on blood pressure (BP) was noticed in a record of weekly measurements taken mostly on Sunday mornings by a 71-year-old psychiatrist [77]. His data were characterized by a large-amplitude circannual variation peaking in the summer. Although some studies reported higher BP values in summer than in winter in elderly populations [78], BP is usually higher in winter than in summer [79–81]. It seems likely, however, that circannual changes in BP are partly endogenous, even if they are statistically significantly correlated with weather and temperature [82, 83] or wind [79].
Circannual changes characterize not only BP but also a variety of hormones and their receptors [84–87], electrophysiological and other cardiovascular variables, as well as morbidity and mortality statistics [88–93]. For instance, changes in ventricular cardiac function, both during systole and diastole, exhibit a circannual rhythm in clinically healthy normotensive subjects [84]. The renin-angiotensin system may underlie circannual changes in BP in view of the fact that a very prominent circannual variation characterizes the response of renin release to calcium deprivation in isolated rat glomeruli [85, 86]. The surge in late spring coincides with a time of changes in the human circannual rhythm of circulating aldosterone [85]. A circannual rhythm in circulating aldosterone is a major classifier of risk of developing high BP and/or related cardiovascular disease [85]. Noteworthy is the observation that the circannual MESOR of circulating aldosterone is higher in women at low familial and personal cardiovascular disease risk, as compared to women at high risk [85]. This result is contrary to the view that as an aldosterone-secreting tumor of the adrenal cortex is associated with a high BP, a high cardiovascular disease risk should also be associated with elevated circulating aldosterone [77, 94]. The chronobiologic result in health is in keeping with the report of higher plasma aldosterone concentrations in children of parents with vs. without hypertension [95].
Melatonin may also be involved in the coordination of BP as an endogenous central hypotensive factor [96]. Melatonin administration in the evening has been associated with a decrease in BP [97]. Urinary melatonin excretion was also positively correlated with cardiovascular disease risk assessed by questionnaire [98]. Melatonin has been implicated in a variety of cardiovascular pathophysiological processes, including anti-inflammatory, antioxidant, antihypertensive, and antilipidemic functions [99]. In the circulation, the concentration of melatonin follows a circadian rhythm, with high values at night, thus providing time clues to target tissues endowed with melatonin receptors [99]. It has indeed been suggested that cardiovascular effects of melatonin are at least partly mediated via MT1 and MT2 receptors [100]. It was found that MT1 was expressed in healthy and diseased human coronary arteries and that MT2 was present in human arteries and left ventricles, its expression being altered in coronary heart disease [101]. Reportedly, melatonin is also able to synchronize neuronal firing in the suprachiasmatic nuclei (SCN) via membrane receptors, and clock gene expression is apparently sensitive to melatonin in the pars tuberalis [102].
The pineal being a sensitive receptor that can respond to variations of the order of a few nanoteslas (nT) induced by space weather [103–109] may also be involved indirectly in relation to BP changes and their related cardiovascular complications. In Alta, Norway (70° N), changes in geomagnetic activity above 80 nT/3 hours were associated with a reduced circadian amplitude of salivary melatonin [109]. This result is in keeping with a reduction in a melatonin metabolite excretion in the presence of increased geomagnetic activity, which was greater when the ambient light exposure was also reduced [108]. In this study as well, decreased melatonin secretion was observed when geomagnetic activity exceeded a threshold value of 30 nT. Further evidence in the experimental laboratory supports an effect of magnetic storms on heart function. Indeed, the ultrastructure of cardiomyocytes of rabbits was found to be drastically altered at a time coinciding with strong magnetic storms by comparison with that usually observed during quiet geomagnetic conditions [110].
In addition to the circannual rhythm in BP of the 71-year-old psychiatrist, an about-11-year variation similar to the solar activity cycle was resolved nonlinearly in his 26-year record spanning from 1969 to 1994 [111]. The fit of a model containing both a solar cycle and a 1-year cosine curve was statistically significant for solar cycles 20 (11.6 years), 21 (10.3 years), and 22 (7 years) covered by his record. The solar cycle component was statistically significant during each solar cycle for diastolic BP and during the last two solar cycles for systolic BP, acrophases occurring consistently approximately 2 years after maximal solar activity [111]. These results were compared to about-11-year and about-1-year changes in BP already reported in two other cases by the mid-1990s, one a clinically healthy man, the other an elderly man treated for MESOR-hypertension [111].
Since then, the record of self-measurements from the clinically healthy man was extended to span over four decades, and longitudinal time series from six other subjects covering 10 years or longer became available for analysis. These seven biomedical scientists included five physicians, four of them treated for high BP. While two of the subjects provided self-measurements, around-the-clock measurements were obtained by ambulatory monitoring from the other five researchers. One more subject contributed data on urinary excretion of 17-ketosteroids for 15 years [112, 113]. Nonlinear analyses of the original data and of the circadian (or in one case, circaseptan) MESORs and amplitudes revealed the presence of cycles with periods clustering around 5 and 10 years [114] (Fig. 13.9). These results strongly suggest that human physiology may be influenced by nonphotic environmental cycles related to solar activity.


Fig. 13.9
Histogram of periods resolved nonlinearly in records of systolic (S) and diastolic (D) blood pressure (BP) and heart rate (HR) covering decades. One additional subject provided a 15-year series of urinary excretion of 17-ketosteroids. Note cluster around 10 years (© Halberg Chronobiology Center)
Additional evidence stems from the detection in longitudinal BP and HR records of other nonphotic cycles featured in terrestrial-space weather. One such cycle is the transyear detected in solar wind speed [115], which has a period of about 1.3 years. As nonphotic cycles are wobbly, their periods are not stable [116]. While transyears can have periods in a relatively wise range, they are statistically significantly longer than one year. An about-1.3-year transyear was invariably detected in all 43 time series of BP and HR from 13 subjects [117, 118] (Fig. 13.10). When a gliding spectrum was obtained for the SBP record of an elderly man and compared to that of solar wind speed, similarities in the time course of the transyear could be followed between these two variables [119]. Results showed that the transyear in solar wind speed was expressed prominently between 1989 and 1996, after which it splits into two separate spectral bands until 1997 when the signal fades away and is no longer detectable. In SBP, the transyear remains prominently expressed until 1998, after which its amplitude is decreased but remains detectable until the end of the record in 2005, suggesting that this component, while still resonating with its environment, may also be partly genetically anchored [119].


Fig. 13.10
A transyear with a period of about 1.3 years is invariably detected in all 43 time series spanning 10 years or longer, provided by 13 subjects who monitored themselves around the clock by self-measurement or ambulatory monitoring. Periods estimated nonlinearly with their 95 % confidence intervals (© Halberg Chronobiology Center)
Transyears have also been documented in transverse data series from neonates, where they are more prominent than the circannual component [120]. Whereas the about-yearly rhythm predominates over transyears in adulthood on the average, transyears regain prominence in the elderly, as gauged by amplitude ratios of these two cycles [120]. A similar time course as a function of age was also found for the about-weekly component in BP and HR [121–123]. The circaseptan period of neonatal BP and HR measured around the clock for the first few weeks after birth, estimated by nonlinear least squares, correlated positively with the corresponding period of the local index of geomagnetic disturbance (K) analyzed during spans matching each neonatal record [124]. These hybrid neonatal data, obtained around the clock for several days from 32 babies monitored during the first month of life, also showed cross-spectral coherence (>0.70) with K at periods of about 3.3 and 8.8 days [24].
Cross-spectral coherence was also documented in a baby boy born prematurely and monitored longitudinally between Jan 26, 1989 and Jan 22, 1990. His systolic BP was coherent with Bz, the vertical component of the interplanetary magnetic field, at a period of about 5 days, and his diastolic BP was coherent with Bz at a period of about 3.5 days [125] (Fig. 13.11). In adulthood as well, associations between BP and space weather were observed. In a 3-year record (1988–1990) of around-the-clock BP measurements obtained by ambulatory monitoring from a clinically healthy man, cross-spectral coherence with the planetary index of geomagnetic disturbance (Kp) was statistically significant for systolic and diastolic BP at periods of about 27.7 days (SBP: 0.567, P = 0.010; DBP: 0.516, P = 0.025) and 4.2 days [126] (Fig. 13.12). Associations of the BP and HR of another clinically healthy man with Kp and of HR of a 28-year-old woman who spent 267 days in social isolation with Kp and with cosmic rays have also been found [63].



Fig. 13.11
Cross-spectral coherence between diastolic blood pressure of a baby boy born prematurely and Bz, the vertical component of the interplanetary magnetic field, at periods of about 3.5 days (3.56 days; P = 0.008), 2.9, and 5.0 days (© Halberg Chronobiology Center)

Fig. 13.12
Cross-spectral coherence between systolic (left) and diastolic (right) blood pressure of a clinically healthy man and Kp, the planetary index of geomagnetic disturbance, at periods of about 27.7 and 4.2 days (© Halberg Chronobiology Center)
Effects of space weather were also observed in the experimental laboratory. The circadian rhythm of systolic and mean intracardiac pressure measured around the clock in the left and right ventricles of male Chinchilla rabbits was found to be markedly altered in September 1984 when the study coincided with the occurrence of two strong geomagnetic storms, as compared to three other studies carried out between June 1984 and March 1985, when quiet geomagnetic conditions prevailed [110]. Considering the involvement of melatonin in relation to blood pressure, it may also be pertinent that in rats sampled around the clock for 7 days, alterations in the circadian rhythm of pineal and hypothalamic melatonin were found following the second extremum of a moderate double magnetic storm [127]. As compared to quiet conditions, during the storm, the MESOR and circadian amplitude were reduced in the case of pineal melatonin and increased in the case of hypothalamic melatonin [127].
13.4 Solar and Geomagnetic Activity and Cardiovascular Outcomes
Already by 1922, there was evidence for an adverse effect of space weather on human health. Two physicians, Sardou and Faure, and Vallot, an astronomer, meteorologist, and generous supporter of research, then published their finding that severe and fatal illnesses as well as common head cold and other aches were more frequent when there were many sunspots [128]. Whether all or only severe symptoms were considered, a higher incidence when sunspots were present than absent can be validated statistically. Clinical symptoms considered diseases of the heart, blood vessels, liver, kidney, and nervous system, ranging from mild to severe, including excitability, insomnia, tiredness, aches, muscle twitches, polyuria, digestive troubles, jitteriness, shivering, spasms, neuralgia, neural crises, asthma, dyspnea, fever, pain, vertigo, syncope, high blood pressure, tachycardia, arrhythmia, and true angina pectoris [128].
Much credit is also due to Bernhard and Gertraud (“Traute”) Düll for their pioneering work in documenting about-27-day cycles in human mortality and relating these patterns to space-terrestrial weather [129–135]. Using superposed epoch analysis, they showed how space/terrestrial weather may differentially influence human mortality from various causes, the 27-day mortality pattern being different whether death was from cardiac or respiratory disease or from suicide. Specifically, differences in the phase of a common 27-day cycle characterized these mortality patterns, determined on the basis of thoroughly assembled death certificates covering 68 stacked consecutive 27-day solar rotations from January 1928 to December 1932 (5 years) [136].
An association between the incidence of various diseases, myocardial infarctions and strokes in particular, and geomagnetic storms and other electromagnetic variations has long been claimed by scientists in the former Soviet Union [137–139]. As reviewed by Halberg et al. [140], results of direct experiments in which biological specimens are exposed to artificially induced electromagnetic fields support studies of correlation between geomagnetic activity and human morbidity and mortality [141]. Electromagnetic fields have been reported to affect blood pressure [142], baroreflex function [143], and HRV [144]. Effects of geomagnetic disturbances have also been reported on the capillary blood flow of patients with ischemic heart disease [145].
Such effects upon the cardiovascular system may contribute to observed associations between space/terrestrial weather and the incidence of adverse events, such as myocardial infarction. By superposed epochs, a consistent association of excessive geomagnetic activity with cardiovascular disease and myocardial infarctions particularly was documented in data from the 1960s to 1970s in two geographic locations [146]. Despite other reports of increased incidence of heart attacks in the presence of increased geomagnetic disturbances [147–155], not all studies found such associations [156, 157]. In particular, two large studies in the USA, carried out with impeccable inferential statistics, reported no solar effect in their investigation of associations of solar activity and magnetic disturbances with mortality [158, 159]. While there is no good explanation thus far for the controversy, mostly because underlying mechanisms are not well understood, several possible contributing factors have been proposed. They include the usually higher level of artificial magnetic disturbances from industry and domestic appliances in the USA and the fact that mortality from myocardial infarction does not necessarily coincide with the onset of symptoms, should they have been triggered by the magnetic storm [147]. Not following a sequence of several Horrebow-Schwabe (about 11-year) cycles and studying their variability may be another reason [1]. Lipa et al. [159] cautiously considered the possibility that any causal relationship between geomagnetic disturbances and coronary heart disease may be sensitive to geographic location, to the phase of the solar cycle, or to some other parameter which might distinguish their data from those of Gnevyshev and Novikova [141]. Distinguishing between mortality and morbidity data has also been recommended, as an about 10.5 % increase in mortality from myocardial infarction in association with big geomagnetic storms contrasted with a lack of effect on the incidence of morbid events in one study [152].
In a database of over 6.3 million cases requiring the call for an ambulance during the span from Jan 1, 1979 to Dec 31, 1981 in Moscow, Russia, including 85,819 diagnoses of myocardial infarction, cross-spectral coherence was found in association with the local index K of geomagnetic disturbance and with Bz, the north–south component of the interplanetary magnetic field at the same frequency of one cycle in about 3.15 days [125, 160] (Fig. 13.13). A north-to-south Bz turn triggers aurorae in the upper atmosphere and storms in the magnetosphere, the total current in the magnetosphere increasing up to ten times within a few minutes [161]. Defining a storm as a north-to-south daily mean changing from ≥1nT to < −1.5nT, there were 32 events during the 3-year span. Superposed epochs analysis indicated that the daily incidence of myocardial infarctions increased by 7.6 % after a Bz turn (P = 0.027), followed, however, by a 5.9 % decrease on the following day (P = 0.029) (Fig. 13.14). This result was consistently observed during each year considered separately [162]. Using a different method, the result was independently corroborated on the same data set [150], an even larger effect being documented in relation to two other criteria (antipodal index aa > 60 and Forbush decrease in cosmic ray intensity >1.5 %), with additional evidence stemming from another set of data [151].



Fig. 13.13
Cross-spectral coherence between the daily incidence of myocardial infarctions in Moscow, Russia (Jan 1, 1979–Dec 31, 1981), and Bz (left) and local K (right) at similar frequency of about one cycle in 3.15 days (© Halberg Chronobiology Center)

Fig. 13.14
Superposed epochs analysis indicates an effect of magnetic storms on the incidence of myocardial infarction (N = 85,819) and sudden cardiac death (N = 71,525) (Moscow, Russia; 1979–1981) (© Halberg Chronobiology Center)
In another database of 129,205 deaths from myocardial infarction in Minnesota during the span from 1968 to 1996 (29 years), an about-10.5-year component was detected with statistical significance after removal of a linearly decreasing trend (Fig. 13.15). During years of maximal solar activity, there was an excess of 220 deaths per year (about 5 %) as compared to years of minimal solar activity (P = 0.023) [163]. When the data are analyzed separately for each of the three solar cycles, statistically significant differences are found, however, perhaps providing some answer for the different results reported above [140]. Fitting a 10.5-year cosine curve to the detrended data, the model is statistically significant for the spans from 1968 to 1977 (P = 0.006) and from 1978 to 1986 (P < 0.001), but not for the span from 1987 to 1996 (P > 0.50). Whereas acrophases are very similar, differing by only 10° (2.8 % or 3.5 months), amplitudes vary from 131 and 278 deaths/year during the first two cycles to 25 deaths/year during the third cycle. The about-10.5-year cycle can be resolved nonlinearly overall and for the first two cycles, but not for the third, the period and its 95 % confidence interval being estimated as 10.83 [10.00, 11.66] years overall and as 11.48 [3.23, 19.72] and 10.64 [8.60, 13.28] years during the first two cycles, respectively [163]. As seen from Fig. 13.15, mortality from myocardial infarction in Minnesota is also characterized not only by a prominent annual variation, but also by a transyear and a cis-half year with periods of about 1.3 years and 0.42 year. These nonphotic cycles are resolved nonlinearly. They reflect signatures found in solar wind speed [115] and in the solar flare index [164], respectively.


Fig. 13.15
An about-11-year cycle characterizes the daily incidence of mortality from myocardial infarction in Minnesota (1968–1996). In addition to a prominent annual variation, note the presence of a transyear and cis-half year with periods of about 1.3 years and 0.42 year, signatures of solar wind speed and solar flares, respectively (© Halberg Chronobiology Center)
Other diagnoses in the 1979–1981 Moscow database included strokes, hypertensive crises, arrhythmia, and sudden cardiac death, among others [125]. In contrast to the other cardiovascular morbidity statistics, all showing a very prominent weekly pattern, the circaseptan component characterizing sudden cardiac death, although statistically significant, was much less pronounced. Instead, an about-half-monthly component was detected with a period of about 2 weeks in least squares and population-mean cosinor spectra (Fig. 13.16). Nonlinearly, its period is estimated as 15.2 [95 % CI: 15.15–15.30] days. According to the criterion of a nonoverlap of 95 % confidence intervals, this period is statistically significantly different from the half-monthly period characterizing local K analyzed during the same 3-year span. For K, the corresponding period is estimated to be 14.0 [95 % CI: 13.94–14.17] days. Whereas the about-half-monthly period of both sudden cardiac death and local K is estimated with a relatively tight 95 % confidence interval when analyzed over the entire 3-year span, this component’s period shows great wobbliness when the data are analyzed by moving spectra over a shorter interval of 3 months, progressively displaced by 1.5 weeks throughout the time series. This component is not consistently detected either for sudden cardiac death or for local K (Fig. 13.16). A possible resonance with occasional frequency trapping between the two variables at this frequency is suggested by the fact that the half-monthly component is seemingly more prominently expressed in sudden cardiac death when it is detected in the spectrum of K [37, 165, 166].


Fig. 13.16
The least-squares spectrum of the daily incidence of sudden cardiac death shows a peak at a period of about 15 days (top, left), visualized by stacking the data over an idealized double cycle (right). Gliding spectral windows (bottom, left) indicate that this component is expressed more strongly at times when it is also prominent in the local index K of geomagnetic disturbance (© Halberg Chronobiology Center)
Since the tenth revision of the International Classification of Diseases (ICD10), sudden cardiac death (code I46.1) has been separated from cardiac arrest associated with a myocardial infarction and is defined as unexpected death from cardiac causes within 1–24 hours of the onset of acute symptoms. Daily incidences of sudden cardiac death (ICD10, code I46.1, excluding death from myocardial infarction) in Minnesota during 1999–2003 (5 years) were compared to those of daily incidences of mortality from myocardial infarction during the same span in Minnesota and with those of daily (or monthly) incidences of sudden cardiac death in some other geographic locations.
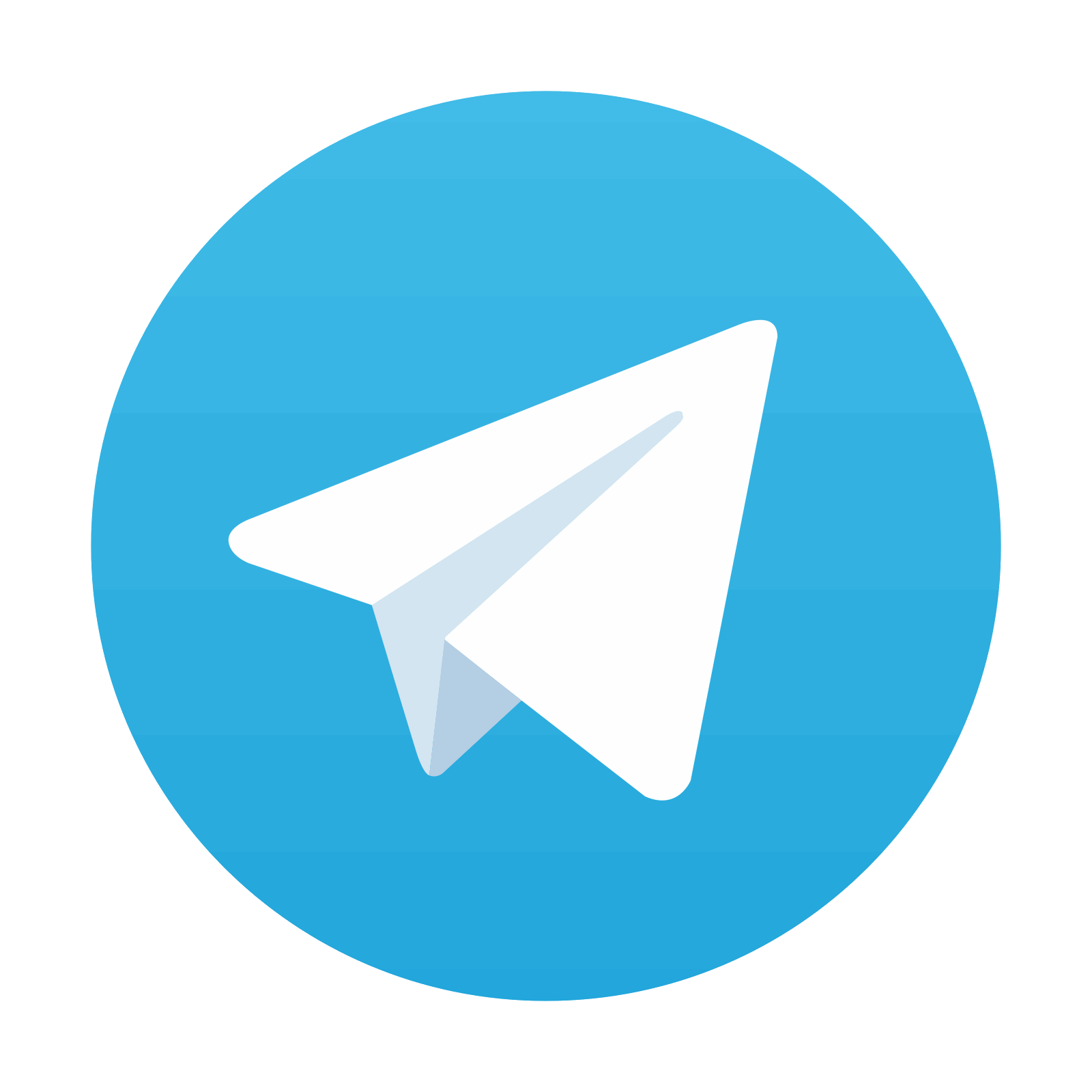
Stay updated, free articles. Join our Telegram channel

Full access? Get Clinical Tree
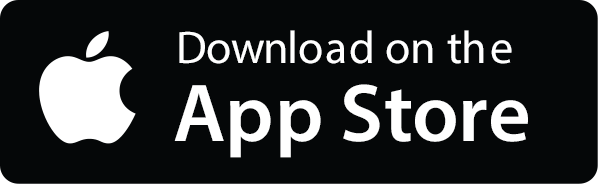
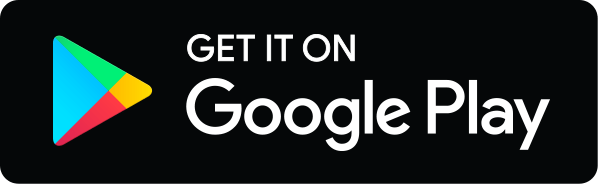