and Rose Tompkins2
(1)
Echocardiography Lab, Leon H. Charney Division of Cardiology, Department of Medicine, New York University Langone Medical Center, 560 First Avenue, Tisch 11 East, New York, NY 10016, USA
(2)
Department of Cardiology, New York University Langone Medical Center, New York, NY, USA
Keywords
AtherosclerosisAtheromaBlue toe syndromeCholesterol crystalsCholesterol emboliHollenhorst plaquesPlaque ruptureIntroduction
Cholesterol embolization syndrome (CES ) refers to the arterio-arterial embolization of plaque debris (predominantly cholesterol crystals) from an atheroma within proximal large caliber arteries, typically in the aorto-iliac-femoral system, to small, distal arteries and arterioles. This results in nonspecific constitutional symptoms and end-organ damage secondary to mechanical obstruction and a provoked inflammatory reaction [1]. Within the medical literature, several terms are synonymous with cholesterol embolization syndrome including atheroembolism, atheromatous embolization, cholesterol embolization, and cholesterol crystal embolization.
Cholesterol embolization is a rare disease that often presents insidiously secondary to the showering of microemboli into smaller distal arteries. This must be distinguished from the related but more common arterio-arterial thromboembolism that results from the acute embolization of larger fragments originating from thrombus overlying a complex atheromatous plaque, which can result in the sudden occlusion of larger downstream arteries with development of severe, acute ischemia [2].
The pathophysiology of CES consists of six key elements: presence of complex atherosclerotic plaque, plaque rupture, distal embolization of plaque debris, lodging of microemboli within distal arteries, foreign body reaction, and end-organ damage. CES can potentially affect any organ system resulting in a wide array of clinical manifestations. The clinical presentation, diagnosis, and management of CES will be reviewed.
History
The first report of CES is believed to have occurred in 1844 by Fenger and colleagues in the Danish medical brochure Ugeskrift for Læger (Doctors’ Weekly) with the autopsy description of Bertel Thorvaldsen, a Danish/Icelandic sculptor [3]. In 1862, this description was translated into German and made available to a wider medical audience [4]. The first autopsy series to provide a detailed description of the diffuse nature and multi-organ involvement of CES was published in 1945 from New York Hospital [5]. Then in the 1950s, the first observation of cholesterol crystals within the arteries of affected organs from frozen pathological specimens was described using polarized light microscopy [6]. To this day, biopsy remains the gold standard for definitive diagnosis of CES.
In 1957, Thurlbeck and Castleman were the first to report CES as a complication of vascular surgery [7]. This was followed by the classic description of pathognomonic retinal plaques in 1961 by Hollenhorst, an ophthalmologist from Mayo Clinic [8]. The blue toe syndrome was first described in 1973 by Karmody and later became synonymous with cholesterol embolization [9]. It was not until 1990 that the association was established between atheromatous aortic plaque visualized on transesophageal echocardiography (TEE) and clinical manifestations of CES; this significantly evolved our understanding of the underlying pathophysiology of CES [10].
Pathophysiology
The pathophysiology of CES consists of six key elements [1]:
- 1.
The presence of atherosclerotic plaque in a proximal, large caliber artery (this can include the aorta, carotid arteries, iliac arteries, or femoral arteries)
- 2.
Plaque rupture
- 3.
Distal embolization of plaque debris including cholesterol crystals
- 4.
Lodging of emboli into smaller caliber vessels leading to partial or complete occlusion
- 5.
Foreign body inflammatory response to the cholesterol crystals
- 6.
End-organ damage secondary to the combination of mechanical occlusion and the local inflammatory response
Atherosclerotic Plaque in a Proximal Artery
The development of generalized atherosclerosis is a lifelong process that begins in childhood and progresses to more advanced stages later in life. Histologically, atherosclerosis is localized to the arterial intima. In childhood to early adulthood, precursor lesions begin to form in the intimal layer that are characterized by fatty streaks containing layers of macrophage foam cells in combination with intracellular and extracellular lipid droplets. These clinically silent lesions then progress to more advanced stages overtime becoming more prevalent in the middle-aged and elderly. Once a lipid core develops, the lesion progresses through various histological stages of increasing complexity from an atheroma to fibroatheroma to a complex plaque associated with plaque hemorrhage, fissure, and ulceration, as well as development of overlying thrombus. These more advanced plaques can also develop calcifications. It is these advanced lesions that provide the source of cholesterol crystals for embolization in CES [11]. The histopathologic stages of atherosclerotic plaque are summarized in Table 27.1.
Table 27.1
Stages of atherosclerotic plaque
Stage | Lesion classification | Histology | Age at onset | Clinical manifestation |
---|---|---|---|---|
I | Initial | Isolated macrophages, foam cells | Childhood and adolescence | Clinically silent |
II | Fatty streak | Intimal fatty streak, intracellular lipid accumulation | ||
III | Intermediate | Stage II lesion with developing extracellular lipid pools | ||
IV | Atheroma | Developing extracellular lipid core | Fourth decade into advanced age | Clinically silent or overt |
V | Fibroatheroma | Stage IV lesion with overlying fibrotic cap | ||
VI | Complicated plaque | Stage V lesion with evidence of fibrotic cap defect, hematoma/hemorrhage within lipid core, thrombus |
An advanced atheromatous plaque is composed of a necrotic core with an overlying fibrous cap (Fig. 27.1). The necrotic core contains cellular debris, macrophage foam cells, and various lipids. The foam cells contain oxidized low-density lipoprotein that is released into the extracellular space upon cell death. This cholesterol-rich material provides the primary source for cholesterol emboli. Cholesterol within a plaque can exist either in a soluble form or a crystalline form. Cholesterol crystals are typically found deep within the necrotic core of the plaque and represent more advanced atherosclerosis. Crystalline cholesterol can account for more than 40 % of the total cholesterol contained within a plaque [12]. The fibrous cap of a plaque is composed of endothelial cells, smooth muscle cells, and connective tissue.


Fig. 27.1
Gross pathologic specimen of aortic atherosclerosis . The abdominal aorta is cut lengthwise to reveal severe advanced atherosclerosis (arrow)
In the context of echocardiographic and radiologic imaging, atheromatous plaque is often classified as simple or complex. Simple plaques appear as smooth border lesions within the arterial luminal wall that have a wall thickness < 4 mm with absence of any mobile components. Complex plaques have a wall thickness ≥ 4 mm often with irregular, ulcerated luminal borders and evidence of mobile components consistent with overlying thrombus .
Simple plaques have an intact fibrous cap that prevents communication between the cholesterol-rich necrotic core and the arterial lumen. Complex plaques often have disruption of the fibrous cap either by fissuring, ulceration, or rupture that exposes the plaque contents to the arterial lumen, thereby creating a potential source for cholesterol emboli. The aorto-iliac-femoral arterial system is the primary location of atheromatous plaques, and thus CES typically localizes to arterial beds of the abdomen and the lower extremities. Therefore, atheroembolism in the upper extremities is uncommon [13].
The risk of embolization is directly related to the presence, severity, and extent of atherosclerosis. This association was first shown in the previously mentioned autopsy series from the late 1940s. Among patients without significant atherosclerotic disease, no CES was observed. However, in patients with aortic plaques, the risk of atheroembolism increased linearly with increasing severity of atherosclerotic disease; moderately eroded plaques were associated with a 1.3 % incidence of atheroembolism and severely eroded plaques with an increased incidence of 12.3 % [5].
Imaging of the Aorta
Aortic plaques can be visualized, characterized, and quantified by a variety of imaging modalities including transesophageal echocardiography (TEE), computed tomography (CT), and magnetic resonance imaging (MRI) [14]. TEE, predominantly two dimensional (2D), is typically the first-line imaging technique for the detection and measurement of aortic plaque (Fig. 27.2) [1]. The association between clinical embolization and advanced aortic atherosclerotic plaque visualized on TEE was first reported in 1990 [10]. Further studies later confirmed that aortic plaque detected by TEE represents a potential source for systemic emboli [15–17]. Noncalcified complex atheromas in the ascending aorta and aortic arch correlated with a higher risk where a plaque thickness ≥4 mm measured on TEE emerged as a clinically important predictor of increased vascular embolic events [17–19]. These embolic events could have represented both arterial thromboembolism and atheroembolism. However, additional case reports with biopsy-proven CES found an association with complex aortic atheromas visualized on TEE, thereby strengthening not only the role of the aortic plaque in CES but also the role of TEE in detecting these plaques [20]. Real-time three-dimensional (3D) TEE is a newer imaging technique that may provide additional morphological detail of the plaque and could have an increasing clinical role in the future [21].


Fig. 27.2
2D and 3D TEE imaging of aortic plaque. (a, b) 2D TEE comparison of normal aortic arch (a) and severe ulcerated plaque (arrows) in the aortic arch. (c, d) 3D TEE demonstrates absence (c) and presence (d) of severe atherosclerotic plaque (arrows) in the aortic arch
CT and MRI are additional imaging techniques that can be considered for detection and characterization of aortic plaque that may provide a less invasive and more comprehensive evaluation of aortic atherosclerosis relative to 2D and 3D TEE (Figs. 27.3 and 27.4) [22–24]. For imaging of the major branches of the thoracic aorta, CT and MRI are superior to TEE as TEE has a blind spot around the origin of the brachiocephalic artery due to interposition of the air-filled bronchi between the esophagus and the artery. In addition, the abdominal aorta is incompletely visualized on TEE as it is limited to the proximal abdominal aorta between the diaphragm and origin of the superior mesenteric artery. Therefore, for complete visualization of the aorta-iliac-femoral arterial system, CT or MRI is preferred .



Fig. 27.3
CT imaging of aortic plaque. 2D CT (a, b) and 3D CT (c, d) imaging of atherosclerotic plaque in the aorta. (a) Coronal cut demonstrates severe calcified atherosclerotic plaque in the aortic arch (arrow). (b) Severe calcified plaque is seen in the entire abdominal aorta and its iliofemoral branches (arrows). (c, d) 3D-reconstructed CT images show diffuse focal plaque in the thoracic and abdominal aorta (arrows) (Courtesy of Dr. Robert Donnino, Veterans Affairs New York Harbor Healthcare System, New York, NY, and Departments of Medicine and Radiology, New York University School of Medicine, New York, NY)

Fig. 27.4
MRI imaging of aortic plaque . (a) Magnetic resonance angiography (MRA) demonstrates a prominent ulcerated plaque (arrow) in the inferior aspect of the aortic arch in a near-sagittal cut. (b) MRA shows a protruding ulcerated plaque in the descending aorta (arrow) in an axial cut (Courtesy of Robert Donnino, Veterans Affairs New York Harbor Healthcare System, New York, NY, and Departments of Medicine and Radiology, New York University School of Medicine, New York, NY)
Conventional arteriography has a low sensitivity for aortic plaque detection and often fails to detect plaques that are identified on other imaging modalities. In addition, arteriography is invasive and could lead to mechanical disruption of an aortic plaque and subsequent arterial cholesterol embolization or thromboembolism and, therefore, should generally be avoided [25].
Plaque Rupture
Rupture of the fibrous cap is a necessary event in the development of CES. This exposes the cholesterol-rich core of the atherosclerotic plaque to the arterial lumen. Plaque rupture can either be spontaneous or traumatic. Traumatic plaque rupture can be secondary to mechanical disruption from intra-arterial manipulation during catheter or surgical procedures. It remains controversial whether or not thrombolytic or anticoagulant therapy is an independent risk factor for plaque rupture and cholesterol embolization.
Spontaneous Atheroembolism
Spontaneous rupture of an aortic plaque shares similar mechanistic features to plaque rupture in other arterial beds. Plaque composition rather than plaque size is thought to play the principal role in plaque vulnerability. More vulnerable plaques often have larger lipid cores and thinning of fibrotic caps mediated by a complex interaction between inflammatory and extracellular matrix cells. Therefore, inflammation may play a critical role in altering plaque composition and thus increasing a plaque’s vulnerability to spontaneous rupture [26].
The rate of spontaneous aortic plaque rupture in the general population has been extrapolated from older pathoanatomic series that were published in the era before the widespread use of intra-arterial cannulation for diagnostic and therapeutic purposes; this estimated rate ranged from <1 to 3.4 % [5, 27, 28]. However, the incidence of spontaneous plaque rupture leading to CES remains low even among higher-risk populations. One antemortem retrospective study of 519 patients with complex aortic plaque diagnosed by TEE found CES to occur at a rate of 1 % over a 3-year follow-up. For comparison, this CES rate is significantly lower than the 20 % rate of arterial thromboembolism observed in the same cohort [29].
Traumatic Plaque Rupture
Traumatic aortic plaque rupture can occur following intra-arterial manipulation during catheterization or cardiovascular surgery. Although cholesterol embolization has been reported in association with cardiac catheterization, it is considered a rare complication. The reported incidence of clinically apparent CES after cardiac catheterization is <2 % [30–32]. The data remains inconclusive regarding whether or not radial access versus femoral access results in a lower incidence of this complication [30, 33]. CES, particularly cerebral atheroembolism, has been a major concern following transcatheter aortic valve replacement (TAVR) with early registries reporting an incidence of 2.4–4 %. However, the incidence seems to be declining with the use of smaller catheters and more stringent patient selection criteria. The routine use of intra-arterial embolic protection devices may lead to an even further decline in embolization events [34].
CES in association with cardiovascular surgery is also rare. Risk is directly correlated with extent and severity of atherosclerosis in the ascending aorta. CES has been reported more frequently in association with coronary revascularization than valvular operations [35]. Off-pump cardiovascular surgeries may lead to less embolic events compared to the use of cardiopulmonary bypass techniques [36]. CES has also been a rare complication associated with carotid endarterectomy, carotid stenting, and abdominal aorta procedures including endovascular aneurysm repair (EVAR) [37–39].
Thrombolytic and Anticoagulation Therapy
The incidence of intra-plaque hemorrhage and an associated increase in plaque rupture leading to CES as a consequence of thrombolytic or anticoagulation therapy remains controversial. Case reports have suggested that CES can develop subsequent to thrombolytic therapy given for the acute management of various conditions including myocardial infarction and deep venous thrombosis. Although a small prospective trial of post-myocardial infarction patients treated with or without thrombolytic therapy failed to demonstrate a relationship [40], there still exists a controversy [41]. Similarly, case reports have suggested that anticoagulation is also associated with an increase in CES and that discontinuation of therapy can lead to clinical improvement [42, 43].
In addition to warfarin, the novel anticoagulants have also been suggested to play a role in development of CES [44]. However, it is difficult to determine if this is a causal relationship or merely an association. At this time, no randomized trials have specifically evaluated anticoagulation as an independent risk factor for development of CES. There is limited literature to support that the use of anticoagulation in patients with concurrent CES is safe and feasible among patients with a separate indication for anticoagulation [45]. Therefore, per current evidence, the causal relationship between cholesterol embolization and the use of anticoagulation and/or thrombolytic therapy can neither be proven nor refuted. Based on this unresolved controversy, routine use of anticoagulation among patients with CES is not generally recommended. However, use of anticoagulation in CES appears reasonable in patients with a separate indication for anticoagulation including atrial fibrillation, left ventricular thrombus, or mechanical prosthetic valve [1].
Embolization of Plaque Debris
Plaque rupture is thought to lead to a showering of plaque debris including cholesterol crystals to a variety of distal tissue and organs. Showering of microemboli typically occurs slowly overtime and may not become clinically apparent until significant end-organ damage has occurred. This is in contrast to arterial thromboembolism where a large thrombus embolizes all at once. Currently, there are no diagnostic tests to definitively detect cholesterol microembolization in the absence of clinical findings; therefore, it is possible that there are many cases of silent cholesterol plaque embolization that have gone undiagnosed.
Lodging of Emboli into Smaller Caliber Vessels
Once released from a plaque, cholesterol crystals travel through the arterial circulation until they reach smaller caliber arteries or arterioles where they become lodged within their lumens. In routine biopsy specimens, cholesterol crystals are not visualized directly because they are washed away during standard specimen processing. However, characteristic ovoid or crescentic clefts within the lumens of the affected vessels can be seen; they represent voids in which the crystals had previously been located (Fig. 27.5) [46]. Direct visualization of cholesterol crystals is also possible if the biopsy specimen is preserved with liquid nitrogen then viewed with polarized microscopy; the crystals will demonstrate birefringence (double refraction of polarized light) [6].


Fig. 27.5
Cholesterol clefts on histopathology. A hematoxylin-eosin-stained section of a small renal artery demonstrates several pathognomonic empty spaces referred to as cholesterol clefts (arrows) within the partly fibrosed (asterisk) lumen. Magnification 200× (Courtesy of Dr Amy Rapkiewicz, Department of Pathology, New York University Medical Center, New York, NY)
Inflammatory Response
In addition to mechanical obstruction, cholesterol emboli incite an inflammatory reaction. This response has been well documented in an animal model and consists of three phases: acute inflammation, foreign body reaction with intravascular thrombus formation, and endothelial proliferation and fibrosis. The acute inflammatory stage is marked histologically by local infiltration of polymorphonuclear cells and eosinophils. Over the following 24–48 h, the cholesterol crystals elicit a foreign body response leading to infiltration of mononuclear cells and their transformation into giant cells that phagocytizes the cholesterol crystals. Simultaneously, thrombus develops in the arterial lumen. In the final stages, there is a proliferation of endothelial cells within the wall of the affected vessel with an eventual progression to intravascular fibrosis that leads to long-term stenosis or complete obliteration of the artery. This results in tissue ischemia and necrosis manifested as end-organ damage [47].
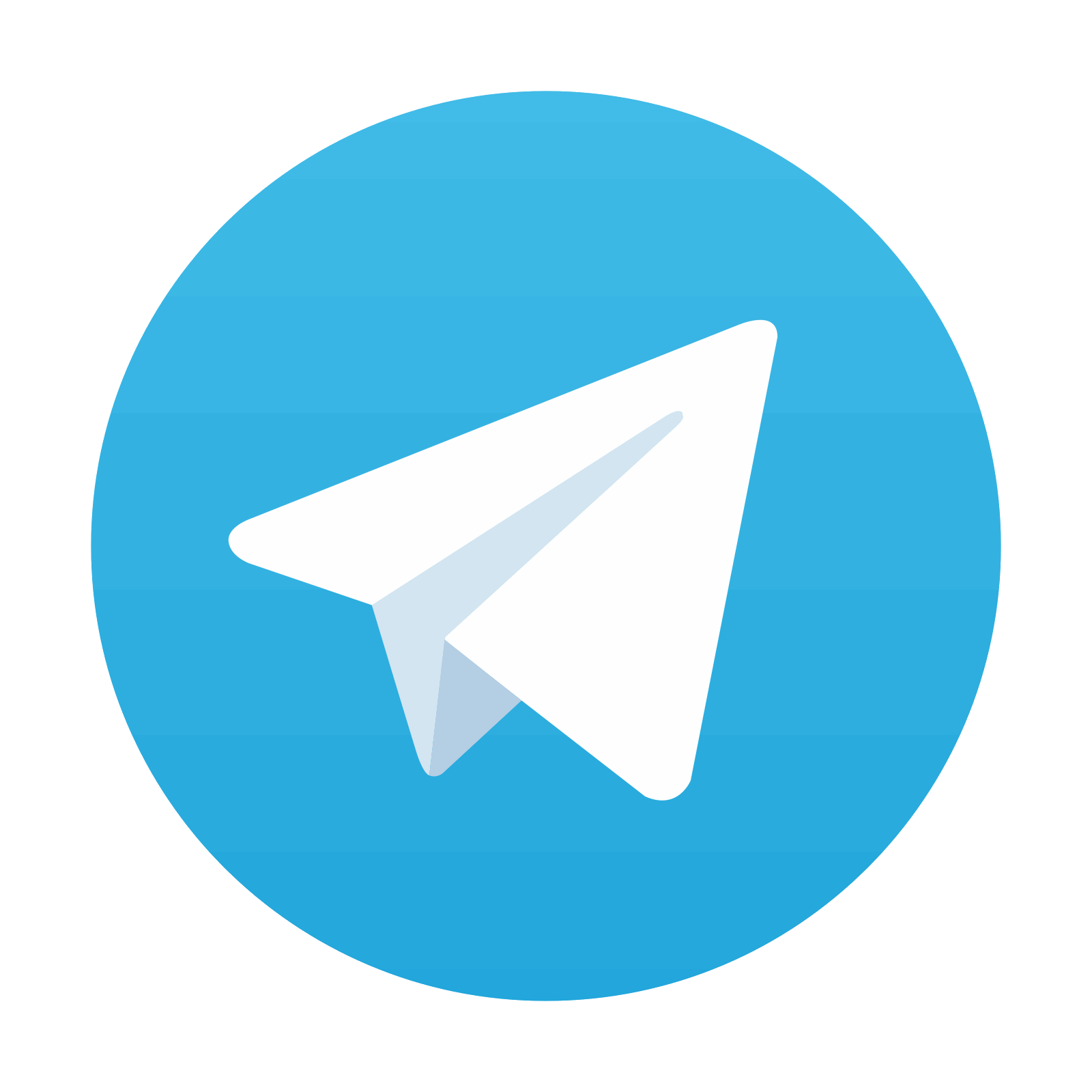
Stay updated, free articles. Join our Telegram channel

Full access? Get Clinical Tree
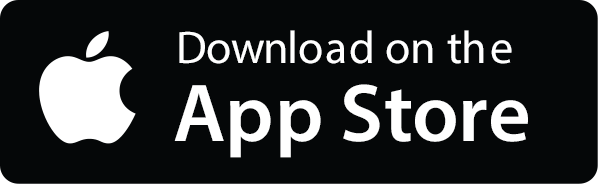
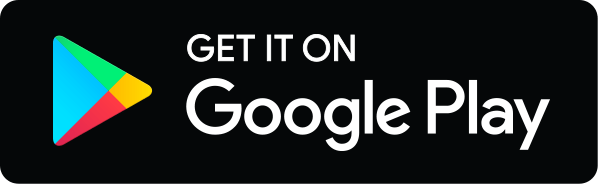