Chest Tubes
Definition
Tube thoracostomy has been described since antiquity, with the earliest accounts of using a tube for drainage of empyema from the pleural cavity from Hippocrates. Original chest tubes were implemented from fashioned reeds or metal tubes. Modern chest tubes are typically latex-free silicone or polyvinyl chloride (PVC) tubes ranging from size 6 to 40 French (Fr), with multiple proximal fenestrations to improve drainage (see Fig. 16.1 ). Multiple differing sizes and shapes such as right-angle or pigtail tubes are available. Additionally, several different insertion methods have been described, including a surgical cut-down approach, trocar insertion, and a percutaneous method. The purpose of all of these tubes is to evacuate air, pleural fluid, blood, or pus from the pleural space to allow apposition of the visceral and parietal pleura and enable normal respiratory mechanics. These tubes also afford the ability to instill fibrinolytics, agents for pleurodesis, antibiotics, or other medications into the pleural space as well.

History
Since the age of Hippocrates, ongoing controversy existed on whether thoracic injuries should be managed in an open or closed method. The first flexible chest tube with use of a water seal was described to treat empyema in 1873 by Playfair. Subsequently, major conflicts and war propelled rapid advancement of surgical techniques to manage blast and penetrating chest injuries. World War I coincided with the influenza epidemic of 1918 leading to the Empyema Commission and increasing recognition of the importance of timely drainage of empyema. At the time, open thoracic drainage with rib resection was associated with high mortality. In the 1920s, closed pleural drainage gained recognition, and by the outbreak of World War II chest tubes were left in place after thoracotomy. Even after World War II, serial thoracentesis was still common as the primary management for hemothoraces. It was not until the Vietnam war when closed pleural drainage gained traction as the primary means to evacuate the pleural space in traumatic injuries such as hemothorax and pneumothorax. Advances in medical and surgical techniques discovered on the battlefield were adopted to hospitals and civilian life and applied in routine clinical practice.
Anatomy and Physiology
With tidal respiration, the diaphragm and external intercostal muscles contract decreasing intrathoracic pressure within the thorax. Intrapleural pressure is typically −5 cm H 2 O at functional residual capacity, slightly subatmospheric due to the recoil of the chest wall and lung elasticity. With tidal respiration, the intrapleural pressure drops to about −8 cm H 2 O as intrathoracic volume increases. These oscillations in pressure are responsible for restful tidal respiration. A defect within the lung or chest wall can cause loss of pressure and inadequate ventilation. Accumulation of fluid or air within the pleural space can compress lung parenchyma and impair respiratory mechanics.
Chest tubes connected to a closed drainage system allow for continuous drainage without influx of air into the chest. Several closed thoracic drainage systems including smaller portable models are available, which follow the same general principles. Most drainage systems utilize a three-chamber closed system (see Fig. 16.2 ). The first collection chamber closest to the patient is a reservoir where pleural fluid collects and can be measured. The second water-seal chamber is connected in-line and functions as a one-way valve. Air escapes out of the first collecting reservoir beyond the water seal but cannot return to the chest. Escaping air that travels through the water seal can be seen as bubbles. The third suction chamber allows regulated negative pressure to be applied, typically −20 cm H 2 O but ranging up to −40 cm H 2 O. If no suction is applied, then the system is said to be on water seal. Because the second chamber functions as a one-way valve, the maximum pressure in the pleural space cannot exceed the height of the column of water in the water-seal chamber, typically 2 cm.

Intermittent or continuous air leaks can be identified by the presence of air bubbles within the water-seal chamber. Note that in some older systems, the third suction control chamber that regulates the amount of negative pressure had water in it, which, when connected to wall suction, would bubble. This is not to be mistaken for the water-seal chamber, which is different. It is air bubbles coming through the water-seal chamber that indicates whether an air leak is present. Air leaks can be caused by leaks at any point in the drainage system, whether they are due to true lung disease or to loose connections, dislodgement of the chest tube, or equipment malfunction.
Air leaks caused by incomplete or loose connections between the chest tube and the closed drainage system or a tube that has migrated out of the chest and is entraining ambient air will generally cause continuous air leaks in the water-seal chamber if the system is on suction. If the system is not on suction (i.e., it is on water seal), such leaks may not be apparent. When a patient is on water seal, tidal variations in pleural pressure can be observed by movement of pleural fluid within the tubing if the system is closed and working properly. Lack of “tidaling” while on water seal without visible bubbles can indicate either an occluded chest tube or evacuation of all pleural fluid such that the lung has expanded completely to cover all fenestrations. Air leaks due to lung disease (such as bronchopleural fistulas) may be intermittent and flow rates may vary both over time and with phases of the respiratory cycle.
Cerfolio devised a classification system to grade the degree of air leaks (see Box 16.1 ). Newer collection systems incorporate digital monitoring that can detect and quantify air leaks more precisely in addition to monitoring fluid output. The magnitude of the airflow through an air leak predicts time to air leak closure, with higher flow rates being associated with longer time until closure. Continuous monitoring, by informing physicians about flow rates, indirectly informs them about the probability of air leak closure, and may facilitate better management decisions and lead to earlier chest tube removal, which in turn may decrease hospital length of stay. For pneumothorax or prolonged air leak not accompanied by blood or pleural effusion, a rubber one-way Heimlich valve can also be considered as a less cumbersome alternative to water seal.
- •
Grade 1, FE. Air leak present during forced exhalation or coughing only
- •
Grade 2, E. Air leak present during expiratory phase
- •
Grade 3, I. Air leak present during inspiratory phase
- •
Grade 4, C. Continuous air leak during both inspiration and exhalation
Chest tube insertion can be a life-saving procedure with several established indications (see Box 16.2 ). In these circumstances, the risk and complications are low, although serious injury and death may occur from accidental complications during placement (see Box 16.3 ). No absolute contraindications exist for this possibly life-saving procedure, although relative contraindications include prior pleurodesis, large pulmonary blebs, severe coagulopathy, or severe thrombocytopenia.
- •
Empyema
- •
Hemothorax
- •
Penetrating chest trauma
- •
Pleurodesis via chest tube (talc slurry)
- •
Pneumothorax
- •
Postoperative in cardiothoracic surgery
- •
Symptomatic or complicated parapneumonic effusions
- •
Fistula formation
- •
Hemorrhage
- •
Infection
- •
Lung laceration and intraparenchymal tube placement
- •
Malpositioning into abdominal cavity
- •
Pneumomediastinum
- •
Pneumothorax
- •
Occlusion, kinking, or fracture of tubing
- •
Vascular or mediastinal injury
Procedural Technique
An anatomic triangle of safety is defined for safe insertion of chest tubes using the perimeter outlined by the lateral edge of the pectoralis major, lateral edge of the latissimus dorsi, and the fifth intercostal space (see Fig. 16.3 ). In emergencies, chest tubes should be inserted within this region to decrease risks of placement complications. Aside from emergent circumstances, ultrasound with Doppler is very useful to confirm underlying pleural fluid or pneumothorax at a selected insertion site, exclude any intervening vascular structures, and also measure the thickness of the chest wall. Review of prior imaging is highly valuable to determine if there is an elevated hemidiaphragm or diaphragmatic hernia as well as loculations of fluid. Generally, lateral insertion sites are preferred over posterior locations to afford patient comfort while in bed or in a chair, although loculated pleural fluid collections may dictate limited options for tube insertion location. Procedure sites should never be selected overlying regions of cellulitis or concerning rashes. Tube insertion sites should be selected immediately above each rib to avoid damage to the intercostal nerve and vasculature, which are typically shielded immediately inferior to each rib. Extremely posterior paraspinal approaches should also be approached with caution given evidence that the intercostal artery often runs within the intercostal space unshielded by the ribs within the first 6 cm lateral to the spine.

After informed consent is obtained, an appropriate site is selected and marked, and a time-out is performed to verify the patient’s identity, laterality of the procedure, and relevant clinical data. The skin is cleansed and prepped with topical antiseptics such as chlorhexidine or povidone-iodine. Proceduralists don sterile attire including a sterile gown, gloves, bouffant cap, mask, and protective eyewear. The site is then draped with a sterile field. Local anesthesia is injected within the skin and deeper levels of the chest wall down to the parietal pleura. Adequate analgesia can be accomplished with local anesthesia alone, although an intercostal nerve block or intravenous sedation and analgesia may be considered in some cases.
For surgical chest tube insertion, scalpel incision is made parallel to the rib through the skin. Subcutaneous fat, fascia, and intercostal muscles of the chest wall are then bluntly divided. The parietal pleura is also entered bluntly immediately over the rib with the closed end of a pair of curved Kelly forceps. This tract is then dilated and a gloved finger is used to enter the rent within the parietal pleura and the finer is then used to sweep circumferentially to ensure there are no pleural adhesions. Next, using curved Kelly forceps, the chest tube is guided through the tract into the pleural space. An alternative method of surgical chest tube placement is to use a preloaded chest tube over a sharp trocar rather than the Kelly forceps.
Once the chest tube has successfully entered the pleural space, it may be directed either apically or toward the base of the thorax depending on indication. Apical chest tubes are warranted for pneumothorax, while a more basal position may be required for pleural effusions and empyema. The chest tube is anchored into position with sutures, covered with gauze, and secured to the chest wall with tape. Attention should be paid to ensure all connections are tight and secured. Chest tubes should be connected to a closed drainage system on either water seal or continuous suction (typically −20 cm H 2 O) initially.
Alternatively, chest tubes can also be placed percutaneously over a wire utilizing a Seldinger technique similar to that used for central venous catheter placement. With this technique, ultrasound use, site location, prepping, draping, and steps for anesthesia remain the same. A needle is then advanced immediately over the rib into the pleural space with confirmation of correct location either by aspiration of pleural fluid or air in the case of pneumothorax. Next, a flexible wire is introduced into the pleural space via the needle and then the needle is withdrawn leaving just the flexible guidewire. One or more dilators are then passed over the wire until the passage is large enough for the chest tube to pass. For a small chest tube that might be used for a pneumothorax, typically only one dilator will be needed, whereas for larger chest tubes, progressively larger dilators would be used. Once the tract is dilated sufficiently, the chest tube is loaded with a stiffening trocar inside of it and the chest tube/trocar unit is passed over the wire into the pleural space. At this point the trocar and wire are removed and the chest tube is secured as described earlier. Proponents of this method favor the ease of insertion, minimally invasive technique, and smaller incision at entrance site.
Selection of chest tube size remains a controversial topic, although use of small-bore (≤14 Fr) chest tubes is increasing. Procedural indication should guide the choice of chest tube size. Larger chest tubes are preferred by some physicians in cases of hemothorax, empyema, or large air leaks in patients on positive-pressure ventilation because they may in theory be less likely to clog, kink, or become dislodged. For these reasons they are often selected in trauma settings. However, small-bore chest tubes are tolerated better by patients, with less pain and splinting. In addition, studies suggest that small percutaneous chest tubes are as efficacious in management of intrapleural infections, including empyema, as larger tubes are. Smaller chest tubes can be flushed routinely with sterile saline to preserve patency.
Once in place, chest x-ray is obtained to verify location. The insertion depth at the skin should be noted in case there is a concern for tube migration or dislodgement. In particular, the distance of the most proximal fenestration to the chest wall should be noted, as any outward migration of the tube can dislodge this fenestration into the tissues of the chest wall. In the case of drainage of infection or instillation of medications into the chest, extrathoracic fenestrations may seed these tissues with infection or allow unintended medication administration. The cumulative fluid drainage through the tube and presence of an air leak should also be charted. A practical step is to mark the date and time next to the fluid level within the drainage system with each nursing shift. In the case of pneumothorax, ongoing air leak is evaluated by the presence of air bubbles during the respiratory cycle in the water-seal chamber ( Fig. 16.4 ).

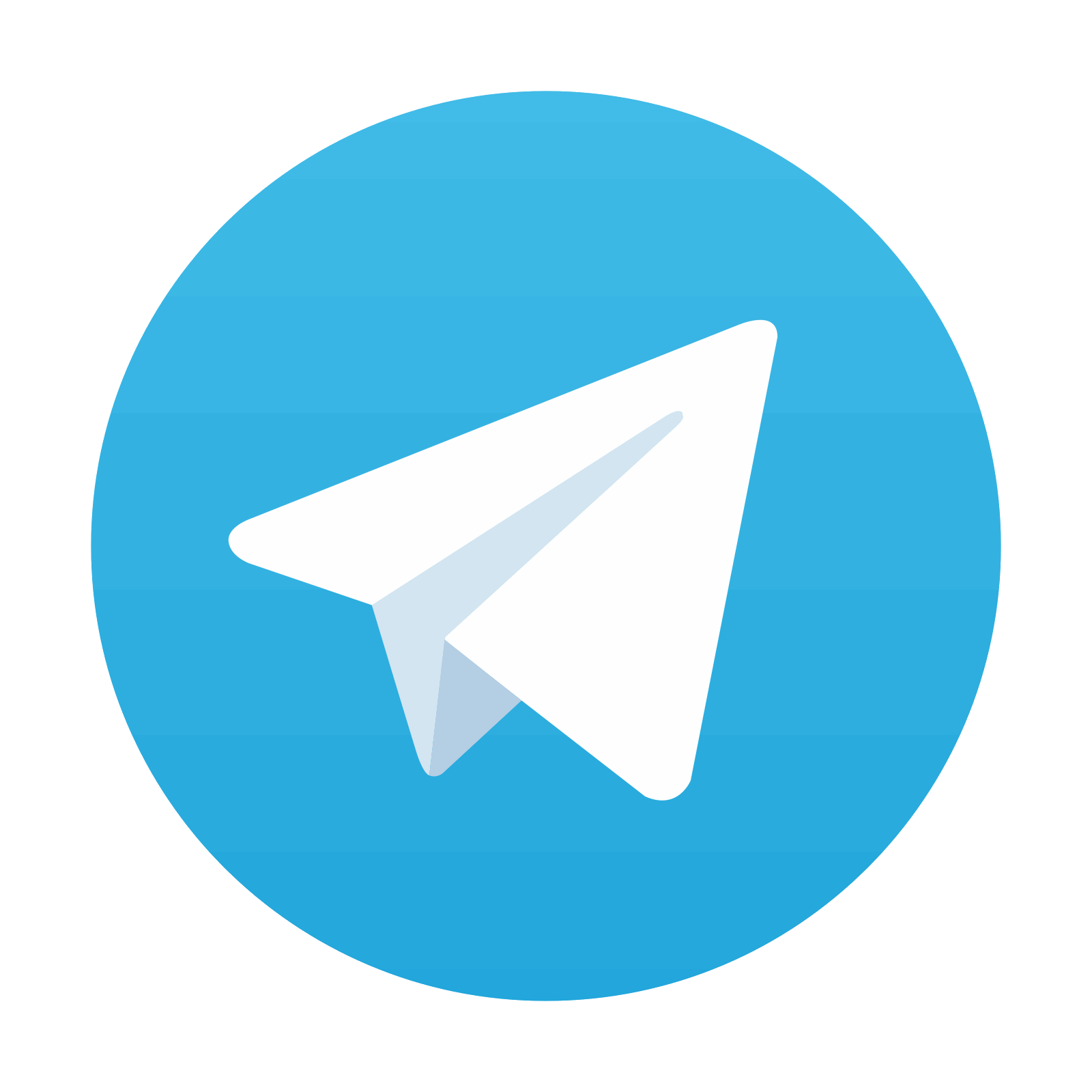
Stay updated, free articles. Join our Telegram channel

Full access? Get Clinical Tree
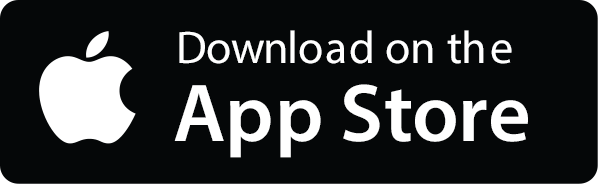
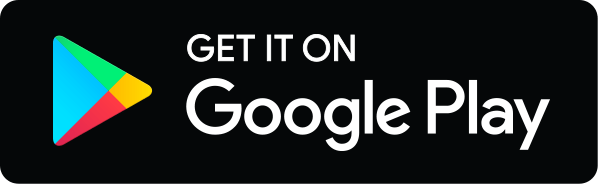
