Chest Radiography
Jonathan Alis
Linda B. Haramati
INTRODUCTION
The chest radiograph is one of the most frequently performed imaging tests. Its wide availability, relative low cost, and minimal radiation exposure (0.01-0.02 mSv) result in it being the most common initial imaging test in a patient presenting with chest pain or dyspnea with a suspected cardiac origin. More sensitive modalities such as echocardiogram, computed tomography (CT) and magnetic resonance imaging (MRI) have changed the role of the radiograph to a gateway to further appropriate diagnostic imaging. Guidelines almost exclusively recommend chest radiography as the initial imaging test in a symptomatic patient, while acknowledging that radiographs cannot exclude cardiovascular disease.1 This chapter focuses on the chest radiograph’s dual roles in either supporting a cardiac diagnosis and providing information regarding cardiac function or identifying alternative pathology as the cause of the patient’s symptoms.
INDICATIONS
The American College of Radiology’s appropriateness criteria recommend chest radiography in patients with acute chest pain from suspected acute coronary syndrome, aortic dissection, or pulmonary embolism. Similarly, in patients with suspected new-onset or unstable heart failure or dyspnea of suspected cardiac origin, a chest radiograph is considered appropriate as initial imaging.1
ANATOMIC CONSIDERATIONS
General Anatomy
The right lung has three lobes, and is slightly larger compared to the two-lobed left lung. The right major fissure divides the right upper and middle lobes from the lower lobe, and the left major fissure divides the left upper lobe from the left lower lobe. Typically, the major fissures are only seen on lateral radiographs. The right lung has a minor fissure that separates the right middle lobe from the right upper lobe, and can be seen on a frontal radiograph as a horizontal linear opacity at the level of the fourth anterior rib. The tracheal air column is seen both on the frontal and lateral radiographs. The right- and left-sided bronchi vary by laterality and are visible centrally on a frontal chest radiograph.
The hila comprise a composite of pulmonary arteries, veins, central bronchi, and lymph nodes, with the radiographic appearance predominantly attributed to the pulmonary arteries and superior pulmonary veins. The right and left hila differ depending on pulmonary artery anatomy, which varies by laterality, and the relationship between the vasculature and bronchi. The left hilum is normally cephalad compared to the right hilum, and should never be inferior, relative to the right. On a lateral radiograph, the right pulmonary artery courses anterior to the bronchus, which is eparterial, whereas the left pulmonary artery arches over the hyparterial left main bronchus and courses posteriorly.
On the frontal view, the right mediastinal border is created by, from superior to inferior, the superior vena cava (SVC), paratracheal stripe, azygous vein, right atrium, and the inferior vena cava (IVC). The left mediastinal border is created by, from superior to inferior, the left subclavian vein, aortic arch and descending aorta, pulmonary artery, left atrial appendage (when enlarged), and the left ventricle. On the lateral radiograph, the mediastinal border is formed anteriorly, from superior to inferior, by the ascending aorta, pulmonary artery, and right ventricle; and posteriorly, aortic arch, descending aorta, left atrium, left ventricle, and IVC2 (Figure 32.1).
Cardiac Position/Visceroatrial Situs
The heart is normally positioned to the left of the sternum, with the cardiac apex pointing toward the left, termed levocardia. Malpositioning of the heart can be either dextrocardia (within the right hemithorax) or mesocardia (midline), and can be either primary or secondary. Primary malpositioning is a congenital cardiac anomaly affecting both the positioning and the axis, often associated with other congenital cardiac anomalies. Extracardiac causes, such as hypoplastic lung, or bony abnormalities, such as scoliosis or pectus excavatum, can secondarily impact cardiac position, termed dextroposition or mesoposition. In dextrocardia, the position of the heart and the direction of the cardiac apex do not correspond to the expected radiographic anatomy.3 The Van Praagh segmental approach, used in classifying congenital heart disease, takes into account the visceroatrial situs, ventricular looping, and great arteries’ position.3 The pulmonary artery and bronchial anatomy on chest radiographs provide information regarding visceroatrial situs, which, in combination with cardiac position, can suggest the possibility of congenital heart disease.4,5 Abdominal situs is often described by the position of the stomach bubble on the radiograph. The severity and incidence of congenital heart
disease is associated with situs and cardiac position. Situs solitus with levocardia, the normal configuration, has a congenital heart disease incidence of less than 1% (excluding bicuspid aortic valve), whereas situs inversus with dextrocardia has a 3% to 5% incidence. Situs solitus with dextrocardia and situs inversus with levocardia have a congenital heart disease incidence greater than 90%.4
disease is associated with situs and cardiac position. Situs solitus with levocardia, the normal configuration, has a congenital heart disease incidence of less than 1% (excluding bicuspid aortic valve), whereas situs inversus with dextrocardia has a 3% to 5% incidence. Situs solitus with dextrocardia and situs inversus with levocardia have a congenital heart disease incidence greater than 90%.4
![]() FIGURE 32.1 Mediastinal contours are demonstrated in the frontal posteroanterior (PA) radiograph (A) and the lateral radiograph (B). IVC, inferior vena cava. |
Aortic Arch
The aortic knob corresponds to the distal most aspect of the aortic arch as it courses downward to become the descending aorta. At this point, the arch is tangential to the x-ray beam on the frontal view, projecting as a circle that is obscured on the right side by the mediastinum and delineated along its left border by the lucent lung.6
The normal arch courses to the left and displaces the trachea to the right, with the aortic knob positioned on the left side of the mediastinum. In a right aortic arch, the aortic knob is seen in the right paratracheal region, and there is displacement of the trachea to the left (Figure 32.2). There are two types of right aortic arch, which can be determined by the branching pattern of the great vessels.7,8 The right arch with mirror image branching is highly associated with congenital heart disease. In contrast, a right arch with an aberrant left subclavian artery is usually an incidental and benign finding, although the left-sided ligamentum arteriosum typically results in a loose vascular ring.
Valves
In a patient with limited clinical history, understanding cardiac implants and support devices and determining valvular position is extremely important. Using the lateral radiograph, a line is drawn from the hilum to the apex, parallel to the long axis of the heart. The aortic valve is above this line and the mitral valve below. On a frontal radiograph, an imaginary line will be drawn from the right cardiophrenic angle to the inferior of left hilum, perpendicular to the long axis of the heart. The aortic valve is above the line and the mitral valve below. On both views, the pulmonic valve is the most superior valve, and, on the lateral radiograph, the tricuspid is the most anterior and inferior on the frontal radiograph9 (Figure 32.3 A,B). Utilizing this technique on supine anteroposterior (AP) radiographs can be inaccurate, and other methods are recommended. The valve orifice method can be utilized, where an aortic valve will be in profile on the frontal radiograph (visualized as a line) while the mitral will be en face (visualize as the whole ring). The perceived direction of flow is useful, with aortic valves being toward the arch and mitral valves toward the apex.10 The radiographic appearance of prosthetic valves is beyond the scope of this chapter. However, there are multiple online resources with pictorial demonstrations of the appearance of mechanic, bioprosthetic, and transcatheter valves.11
FUNDAMENTALS OF RADIOGRAPHY
Approach to Interpreting Radiographs
There are varied approaches to interpreting chest radiographs, and each individual should determine what suits his/her needs. The common denominator for accurate interpretation is a systematic approach. One should also be vigilant to avoid a common error in diagnostic radiology termed satisfaction of search, defined by prematurely ceasing to search for abnormalities once an abnormality has been identified.12 Following a systematic interpretation scheme ensures that all aspects of the radiograph have been interpreted. The clinical value achieved from comparison with old studies cannot be overemphasized. When interpreting a chest radiograph from a cardiac standpoint, the findings have a dual purpose. One should not only attempt to identify findings associated with cardiac disease but, in addition, noncardiac thoracic pathology, if identified, may explain the patient’s symptoms.
Radiographic Technique
The standard views in chest radiography are posteroanterior (PA), lateral, and AP radiographs. A PA radiograph is obtained with the patient standing up against the detector with the x-ray tube posterior to the patient, at a 6-foot distance, by convention; such that the patient is exposed to the x-ray beam, posterior to anterior. The scapulae are cleared from the lung field by the patient placing their hands on their iliac crests and shoulders rotated forward. The patient’s chin should be raised to ensure the apices are not obscured. The radiograph is obtained while the patient is in breath hold at full inspiration, permitting visualization of the 10th posterior rib above the diaphragm. The patient is positioned so that there is no rotation, with the medial ends of the clavicles equidistant from spinous processes. The entire lungs should be demonstrated including the costophrenic angles and apices. There should be adequate exposure so that the thoracic spine and intervertebral disc spaces are visualized in the retrocardiac region. For a lateral radiograph, the patient is erect, seating or standing, with the left side touching the detector. Careful attention is made to ensure the humeri and the chin are excluded from the field of view. Correct positioning is determined by the sternum being seen in profile, the costophrenic angles included, the intervertebral foramina open, and the bilateral anterior ribs and bilateral posterior ribs are nearly superimposed. Adequate exposure should demonstrate the hemidiaphragms clearly, and the ribs should be visualized through the heart and lung markings. When a patient is unable to perform a PA radiograph, a portable radiograph can be obtained. This is particularly useful for patients who are bed bound and/or immobile, because this can be performed supine or semi-recumbent. Typically, these are performed in an AP projection, with the detector positioned behind the patient’s back and the x-ray tube anterior to the patient. Therefore, the x-ray beam travels from anterior to posterior, usually at a distance of 3 feet.13,14
There are additional chest radiographic views, which were previously used more commonly, but still have a role in troubleshooting. The apical lordotic view is used to visualize the lung apices by projecting the clavicles above the lungs, by either having the patient lean back or angling the x-ray tube cranially if the patient is upright. The lateral decubitus view is useful for evaluation of layering pleural effusion and exclusion of loculation, as well as being useful for demonstration of a small pneumothorax in the nondependent lung. In this view, the patient lies on their side and the radiograph is taken using a horizontal beam.13
CLINICAL APPLICATIONS
Pulmonary Vasculature
Congestive Heart Failure
There is extensive literature that supports the use of chest radiography in the diagnosis of congestive heart failure (CHF) in the emergency setting.15 Chest radiographic findings have been shown to correlate with pulmonary wedge pressures. However, radiographic findings may lag behind the clinical onset of heart failure. Conversely, the radiographic findings can persist after normalization of the pulmonary wedge pressure.16
In the upright position, the lower lungs normally have increased blood flow relative to the upper, mainly due to gravity. This can be appreciated on erect radiographs as increased diameter and number of vessels in the inferior lungs. To compensate for varied cardiac output, the pulmonary vascular bed has the capability to recruit when the cardiac output is increased.17 In acute left-heart failure, there is progressive increase of the pulmonary venous pressure. As a result of increased flow or pressure to the pulmonary vasculature, vascular recruitment takes place with concomitant increase in the diameter and number of vessels in the upper aspects of the lungs. This is termed pulmonary vascular redistribution, which is the earliest sign of physiologic changes that can be seen with pulmonary wedge pressure of greater than 12 mm Hg.
During progression of the increasing flow/pressure to 17 to 20 mm Hg, the plasma oncotic pressure will be overcome, resulting in fluid leaking out of the vessels into the interstitium. This is the first phase of pulmonary edema, termed interstitial edema. The radiographic correlation for interstitial edema is thickening of the interlobular septa, which is called Kerley B lines. Kerley B lines can be seen on the frontal radiograph as peripheral thin (1-2 mm), 1- to 2-cm long, horizontal lines that abut the pleura. Edema involving other pulmonary interstitial structures such as the peribronchovascular and subpleural interstitium results in the chest radiographic findings of peribronchial cuffing and thickening of the fissures. Small pleural effusions are often present18 (Figure 32.4A).
When the pulmonary wedge pressure exceeds 25 mm Hg, the fluid buildup in the interstitium exceeds the capabilities of lymphatic drainage, resulting in fluid seeping into the alveolar spaces, causing alveolar edema. During alveolar edema, there is impairment in gas exchange resulting in hypoxia and dyspnea. Radiographically, alveolar edema appears as nodular opacities that coalesce to form patchy, fluffy consolidations in a bilateral, central distribution with sparing of the periphery (Figure 32.4 B).
These findings are often accompanied by moderate-sized pleural effusions, which are typically bilateral but can sometimes be unilateral, commonly on the right. Evaluation for pleural effusions is more sensitive on the lateral radiograph because blunting of the posterior costophrenic angle requires less than 75 mL pleural fluid, whereas blunting of the lateral costophrenic angles on the front radiographs requires at least 250 mL of pleural fluid.18
These findings are often accompanied by moderate-sized pleural effusions, which are typically bilateral but can sometimes be unilateral, commonly on the right. Evaluation for pleural effusions is more sensitive on the lateral radiograph because blunting of the posterior costophrenic angle requires less than 75 mL pleural fluid, whereas blunting of the lateral costophrenic angles on the front radiographs requires at least 250 mL of pleural fluid.18
When pulmonary wedge pressures are chronically elevated, the lymphatic vessels expand significantly, increasing their drainage capabilities, and the lungs become more resistant to pulmonary edema. Conversely, in cases of sudden high pressures, such as from hypertensive urgency, acute myocardial infarction, or new-onset tachyarrhythmia, flash pulmonary edema can occur. During flash pulmonary edema, interstitial edema is followed in quick succession by alveolar edema.19
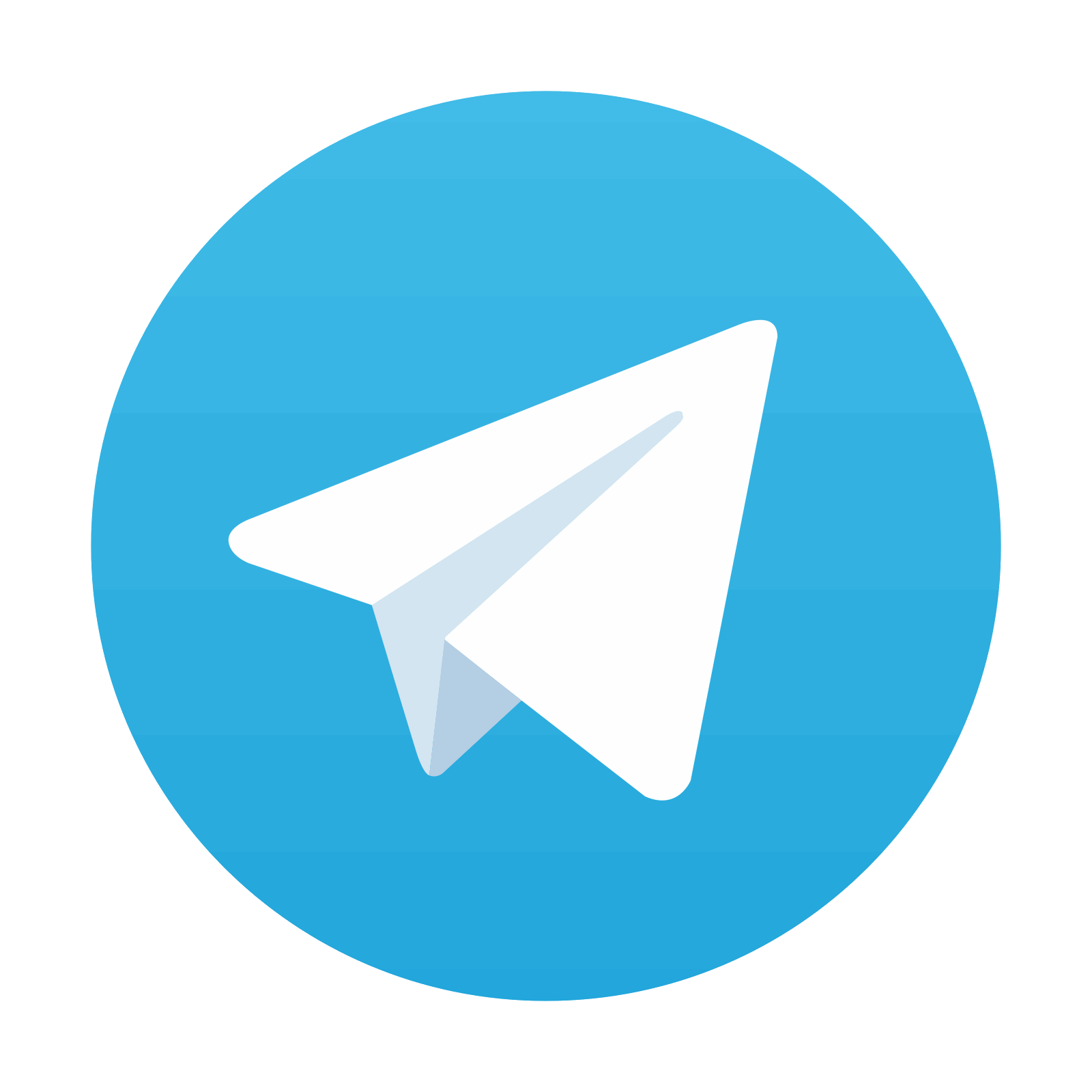
Stay updated, free articles. Join our Telegram channel

Full access? Get Clinical Tree
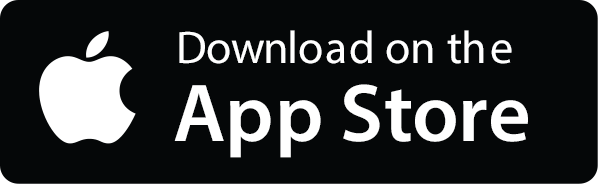
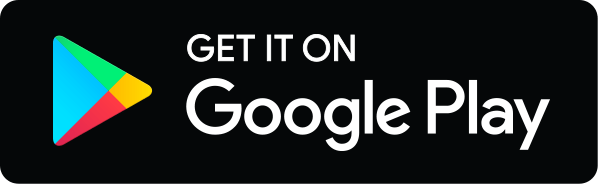
