Keywords
chemoprevention, CT screening, early detection, lung cancer, smoking cessation
Summary of Key Points
- •
Most chemoprevention research is focused on natural products and is not amenable to traditional pharmaceutical development.
- •
Robust research and development for lung cancer chemoprevention by the pharmaceutical industry is largely lacking.
- •
The traditional model of developing drugs for advanced disease and then backing successful agents into early disease application is not working.
- •
Safety is coequal to efficacy in developing a successful chemopreventive agent.
- •
Lung cancer screening with spiral computed tomography is being implemented, and as a result more stage I curative cases will be found.
- •
Individuals followed after successful management of their initial lung cancer will experience a high rate of metachronous second primary lung cancer (1% to 3% cumulative), and this will be a growing new cohort of patients that would benefit from successful chemoprevention management.
- •
Locoregional delivery of potential chemoprevention drugs by “aerosolized approaches” may reduce cost and improve safety.
- •
Systematic study of resected early-stage lung cancers and surrounding injured bronchial tissues may provide insight into the molecular drivers of (early) lung cancer using the precedent of Vogelstein and coworkers’ mapping of colon carcinogenesis.
In a recent editorial published in the Lancet , advances in lung cancer treatment outcomes were reviewed and it was concluded that simple preventive measures, such as banning advertising, blank cigarette packaging, and increasing awareness of the harms of smoking, might result in more reductions in mortality than all new promising agents combined. For the tens of millions of tobacco-exposed individuals, including former smokers, who will remain at increased risk of lung cancer for most of their remaining life, this is a grim message. At the same time, there is clear progress in early detection of lung cancer and computed tomography (CT) screening, which has been endorsed and is being implemented in the United States, and endorsed in Canada and China with pilot screening evaluations being implemented in a number of other countries. Screening will result in finding more early-stage cancers, which in many instances can be cured by surgery, but as outlined in the discussion of field carcinogenesis reviewed in this chapter, many of the surgically cured individuals at a frequency of 1% to 3% per year will manifest a second primary lung cancer. The net effect of this lung cancer screening dynamic is that before too long, we will have tens of thousands of people in follow-up for metachronous primary lung cancer. In a perfect world that would mean the pharmaceutical industry would be focusing on developing drugs that would target the mechanisms driving field carcinogenesis and short circuit the development of these metachronous lung cancers. A comprehensive recent review of chemoprevention relates that for lung cancer there are no new (approved) products and the studies that are being done in this space are generally small, investigator-driven studies of plant-derived agents with little prospect for evolution to a full-fledged pharmaceutical product. Although many studies continue to demonstrate the importance of anti-inflammatory agents, the lung cancer prevention field is remarkably free of major pharmaceutical investment. In this most innovative time in the history of biomedicine, for the world’s most lethal cancer, we have no lead compound and no obvious serious, commercially viable effort to find one. Publications have noted for decades that the incentives for major pharmaceutical involvement in the field of chemoprevention are inadequate, but no structure fix has been implemented.
In a recent chemoprevention review it has been suggested that the process to develop chemopreventive agents mirrored the steps in developing drugs for advanced lung cancer. This position seems to reflect conventional wisdom, but is that really the optimal way to develop a drug to stop or delay the occurrence of early lung cancer? Late drug development failures are often the consequence of off-target drug effects. In considering the application of chemoprevention drugs in asymptomatic target populations, the issue of off-target toxicity is vitally important and more an issue than off-target effects of drugs for symptomatic late-stage lung cancer. Chemopreventive agents have to be effective and safe at the same time. Further, target identification of potential drugs to arrest carcinogenesis of lung cancer has to be derived from tissue that is representative for early carcinogenesis. A model for this would be the comprehensive mapping that Vogelstein and colleagues have conducted in mapping molecular events with colon carcinogenesis. Finally, lung cancer arises principally from the aerosolized delivery of tobacco combustion products to the airway. The epithelial lining of the airways represents a remarkably small volume of cells that can be targeted economically and safely using established methods. Many successfully approved aerosolized products exist for obstructive pulmonary and infectious disease. Chemoprevention for lung cancer presents an exceptionally strategic opportunity for making a major impact in the War on Cancer and generating a new class of lung cancer drugs. It is time to get serious about bringing such tools into being.
Lung cancer is by far the leading cause of cancer-related deaths worldwide. In the United States alone, 158,080 deaths were projected for 2016, accounting for about 27% of all cancer deaths. Median survival in all stages of disease has improved during the past decades, but the likelihood of obtaining a cure, even with optimal modern therapy, is low because most cases are detected after the development of symptoms from respiratory cancer, and these occur when the disease is in a regional or distant metastatic stage. Lung cancer is usually related to exposure to carcinogens that occurred over 10 years to 20 years and requires multiple molecular genetic changes before the development of invasive lung cancer. Consequently, a strong rationale exists for detecting lung carcinogenesis before the development of invasive metastatic cancer. This detection includes prudent implementation of lung cancer screening as a high-quality service to identify early, curable lung cancer.
After an exhaustive review of the evidence, the US Preventive Services Task Force (USPSTF) endorsed low-dose lung cancer screening as an early-detection approach with the potential to significantly reduce deaths from lung cancer. Prospective detection of lung cancer in high-risk cohorts in the absence of symptoms mandates a clinical management approach that differs significantly from the routine management of lung cancer detected on the basis of symptoms. As with other cancer screening services, this screening service must be delivered with high quality, low cost, and ready accessibility. This chapter reviews strategies that contribute to routinely achieving that level of proficiency, and this information may constitute a useful reference for clinicians communicating about the merits and limitations of this service to high-risk individuals who are considering participation in lung cancer screening. As early detection becomes more successful, more patients will survive lung cancer. As a by-product of this success, more patients with cured lung cancer, especially those with heavy exposure to tobacco products, will have a high risk of the development of new primary lung cancers. This outcome produces a strong demand for new tools to manage this earliest form of lung cancer. Chemoprevention is the term used for the process of implementing drugs to interfere with carcinogenesis.
Tobacco smoking is estimated to account for at least 80% of the attributable risk for lung cancer. Smoking cessation, especially before middle age, is associated with significantly lower tobacco-attributable cancer risk. Clearly, we must continue our efforts in tobacco control, which have substantially reduced smoking prevalence in several countries. However, 1.3 billion people worldwide still smoke, and the multinational tobacco companies continue to introduce cancer-causing products designed to entice teenagers into a lifetime of nicotine addiction. In addition, despite a significant reduction in the prevalence of smoking among US adults, from 42% to 19% between 1965 and 2011, lung cancer incidence has not decreased because of the growing number of aging people. Despite smoking cessation, lung cancer risk remains elevated and never reaches the level of risk among nonsmokers. A significant percentage of newly diagnosed lung cancer occurs in former smokers. Thus in certain individuals, the carcinogenic process elicited by tobacco smoke continues to evolve despite smoking cessation. These observations underline why strategies to slow, halt, or even reverse carcinogenesis continue to gain great interest and why it is of interest to explore the evolution and progress of chemoprevention efforts.
In early chemoprevention studies, researchers evaluated vitamins or micronutrients in an attempt to reduce cancer incidence as the primary end point. A challenge with this approach was cost, because such studies required thousands of participants and many years of follow-up to detect a significant chemoprotective benefit. Interpreting results from these trials may also have been complicated by the comingling of study participants who continued to smoke with individuals who had stopped smoking. More recently, study designs have focused on cancer incidence as the primary end point in populations who are at high risk for lung cancer. An example is to exclude individuals with a 20-pack/year history who do not have more than a 10% to 15% lifetime risk of lung cancer. Intermediate marker end points can be used to quickly designate risk strata to select trial populations in whom lung cancer is more likely to develop so that smaller trials can be more informative. Chemoprevention of lung carcinogenesis remains a highly attractive concept, especially in targeting trials on agents that are involved in downstream effects of multiple carcinogens, tumor promoters, and inflammatory compounds in cigarette smoke.
Lung cancer is strongly associated with direct consumption of tobacco products, and 87% of all lung cancers are detected in individuals who are active or former smokers. An additional 6% to 7% of lung cancer occurs in partners of smokers or their offspring. The second and third most common known causes of lung cancer are radon and asbestos, and epidemiologic evidence from the 2000s points to a clear association between lung cancer and air pollution. Despite the high degree of certainty that a link exists between tobacco smoke and lung cancer, establishing the causal link between tobacco exposure and lung cancer took several decades. The discovery that tobacco smoke was capable of producing identical p53 mutations both in patients with lung cancer and in mice exposed to tobacco smoke added incontrovertible evidence for a strong epidemiologic association.
The original description of so-called field of cancerization dates back to the pioneering work of Slaughter et al., who showed that multiple foci of epithelial hyperplasia, hyperkeratinization, atypia, dysplasia, and carcinoma in situ occurred in otherwise normal-appearing epithelium adjacent to cancers of the oral pharynx in smokers. This empiric evidence suggested that carcinogen exposure has widespread effects throughout the epithelial field subjected to carcinogen damage. Auerbach et al. reported that the same pattern of heterogeneous, multifocal histologic changes occurred throughout the bronchial epithelium of smokers who had lung cancer, consistent with the concept of field of cancerization. Translated into contemporary biologic terms, field of cancerization means that areas of histologically normal-appearing tissue adjacent to neoplastic lesions display molecular abnormalities, some of which are the same as in the tumors. Several studies have shown the field of injury created by cigarette smoke, and molecular studies support the stepwise model of lung carcinogenesis, with genetic and epigenetic alterations occurring in the cells. Field carcinogenesis also forms the basis for the observation that among individuals who survive a first cancer, a second malignancy is likely to develop in the region of the tobacco-exposed epithelium.
Among the earliest molecular findings in the aerodigestive tract of chronic smokers are loss of heterozygosity in chromosomes 3p, 9p, and 17p; p53 mutations; and changes in promoter methylation and in telomerase activity of noncancerous epithelial cells. Loss of heterozygosity is a crucial event. For example, loss of the short arm of chromosome 3, which is often the earliest loss and is demonstrated occasionally even in early hyperplasia, results in the loss of a region rich in tumor suppressor genes. Evidence also indicates loss of the short arms of chromosomes 9 and 17, resulting in loss of tumor suppressor genes in p16 and p53, both of which are important to the cell’s ability to repair DNA damage elicited by tobacco smoke. Gene p53, in particular, acts as a transcription factor in the control of G 1 arrest and apoptosis, or programmed cell death, thereby allowing the cell to repair any existing DNA damage or induce apoptosis once the cell is too far damaged for repair. The gene p16, found on chromosome 9p, negatively controls cyclin-dependent kinase–cyclin activity by overexpression of cyclin-D1. Inhibiting cyclin-D1/cyclin-dependent kinase prevents the damaged cell from entering into mitosis and proliferating with damaged DNA. Studies have proved that methylation of the p16 gene in the oral epithelium induced by tobacco smoke is an independent risk factor for lung cancer in current and former smokers.
The loss of these and other tumor suppressor genes in lung cancer seems to be augmented by the activation of several crucial proto-oncogenes. For example, Ras, myc, and the epidermal growth factor receptor (EGFR) are all tumor-promoting genes that are activated progressively during lung carcinogenesis. Mutations of Kirsten rat sarcoma (KRAS) are particularly common in adenocarcinomas of the lung; some studies have shown that 30% of lung adenocarcinomas in smokers contain KRAS mutations.
Other mechanisms of lung carcinogenesis are also relevant, such as amplification of EGFR. Mutations have been detected in the EGFR tyrosine kinase (TK) binding domain, which confer sensitivity to small-molecule EGFR TK inhibitors (TKIs). Both KRAS mutations and EGFR mutations have been found in the airways of smokers and nonsmokers in regions distant from the primary tumor, suggesting that both play a role in field carcinogenesis.
Gene mutations and rearrangements have also been mapped to specific histologic and clinical characteristics among the lung cancer subtypes. This discovery has led to the development of target-specific chemotherapeutic drugs, which have revolutionized the treatment of lung cancer.
Rearrangements of the anaplastic lymphoma kinase (ALK) gene, which result from an inversion in the short arm of chromosome 2, have been identified in a subset of patients with nonsmall cell lung cancer (NSCLC). This subset causes distinct clinical and pathologic features and usually affects younger patients with no history or a light history of smoking. ALK TKIs such as crizotinib and alectinib have been shown to increase progression-free survival rates. Similarly, rearrangements of the c-ros oncogene 1 (ROS1) and ret proto-oncogene (RET) genes have been isolated in adenocarcinoma and in some subsets of advanced NSCLC. These patients, too, are mostly young with little or no history of smoking.
Some mutations are mutually exclusive of each other, such as EGFR and KRAS mutations. Amplification of fibroblast growth factor receptor 1 is seen in patients with squamous cell lung cancer. This mutation is associated with cigarette smoking in a dose-dependent fashion and is an independent negative prognostic factor.
Global messenger RNA and microRNA (miRNA) expression profiles have been described in the bronchial epithelium of so-called healthy smokers, and cancer-specific gene expression profiles have been reported in smokers with and without lung cancer.
Gene Silencing
Another mechanism of lung carcinogenesis is loss of function of tumor suppressor genes. A growing body of evidence suggests that gene silencing through epigenetic means is crucial in lung carcinogenesis. For example, the tumor suppressor gene in NSCLC known as RASSF1A, which is also located in the region of chromosome 3p rich in tumor suppressor genes, encodes a protein that heterodimerizes with Nore-1, an important RAS effector with a proapoptotic effect. Evidence suggests that in NSCLC, RASSF1A can be inactivated by hypermethylation.
Another important gene that can be inactivated by epigenetic means is the retinoic acid receptor-β (RAR-β), which also maps to chromosome 3p. RAR-β is a nuclear retinoid receptor with vitamin A–dependent transcriptional activity. The RAR-β gene is gradually lost during lung carcinogenesis, and this may be caused by loss or hypermethylation. The maintenance of RAR-β in mature tumors is a risk factor for poor prognosis, and the RAR-β gene is differentially regulated in current as opposed to former smokers. For example, a retrospective study on methylation status and the occurrence of second primary tumors (SPTs) in patients with completely resected NSCLC showed an association between RAR-β2 hypermethylation in the development of SPTs only in former smokers. In current smokers, hypermethylation was associated with a protective effect, pointing to the value of context with regard to smoking status in understanding the biologic effects of retinoid receptors in lung cancer.
The role of miRNAs may also prove to be crucial. These small noncoding RNA molecules have been recognized as important in epigenetic control by altering the translation of proteins from messenger RNAs. miRNAs are able to target a multitude of messenger RNAs and have emerged as key posttranscriptional regulators of gene expression involved in cell proliferation, apoptosis, and stress resistance. miRNAs are also located in cancer-associated genomic regions or in fragile sites, suggesting that differences in miRNA expression may be induced by genomic alterations and play either a tumor suppressor or oncogenic role.
Cigarette smoke is a potent source of such molecular injury, and recent studies have shown that the components in cigarette smoke affect miRNA levels in the respiratory tract. An emerging notion is that smoking may lead to downregulation of miRNAs in the airway. The mechanistic understanding of the link between these miRNA changes and early lung carcinogenesis is just beginning to emerge. The fact that miRNA dysregulation is an early event in lung carcinogenesis may offer opportunities for chemopreventive approaches (i.e., reversing aberrant miRNA expression induced by smoking). For example, miRNA changes were found in biopsy specimens obtained in a phase II trial comparing iloprost (a prostacyclin analog) with placebo in high-risk patients, and these changes were related to histologic findings at baseline. Proteomic analyses of preinvasive lesions in the bronchial tree have uncovered patterns clearly different from those in normal epithelium, supporting the rationale for chemopreventive strategies.
Susceptibility to Lung Cancer and Balancing of Antagonistic Pathways
The risk of the development of lung cancer, even among the heaviest smokers, varies widely, perhaps because of genetic and even dietary factors. In fact, 85% of heavy smokers will not develop lung cancer, which suggests important differences among individuals in susceptibility to lung cancer. Several prediction models for lung cancer have been proposed. The Bach model uses variables related to age, smoking, and asbestos exposure in black populations, whereas the Spitz model and Liverpool Lung Project include family history and other occupational exposures in addition to age and smoking history in white populations. Although these models are easy to administer and calculate, their discriminatory power is only moderate, which is not surprising because the models fail to account for many comorbidities and other epigenetic factors. In 2012, Hoggart et al. proposed a prediction model that includes more variables, including biomarker exposure; socioeconomic status; comorbidities including pneumonia, chronic obstructive pulmonary disease (COPD), and emphysema; and body mass index for current smokers, former smokers, and nonsmokers. Despite researchers’ best attempts, a prediction model cannot assimilate all possible epigenetic and genetic factors to make consistently accurate predictions.
Accumulating evidence suggests that genetic and epigenetic factors are crucial in modulating individual susceptibility to lung cancer. An example is the identification of a susceptibility locus for lung cancer at 15q25 in a large genome-wide association study. Locus 15q25 contains nicotinic acetylcholine receptor subunit genes and seems to be involved in carcinogen metabolism. The two major carcinogens from cigarette smoke, benzo[a]pyrene and 4-(methylnitrosamino)-1-(3-pyridyl)-1-butanone, require metabolic activation before they can exert full carcinogenic effects.
Various activation pathways compete with detoxification pathways, and the balance between the two is crucial in modulating cancer risk. Cytochrome P450s serve as carcinogen-metabolizing enzymes, whereas glutathione transferases serve as detoxification enzymes. Both sets of these important genes are known to have significant polymorphisms that correlate with variations in lung cancer risk. Gene polymorphisms in microsomal epoxide hydrolase and their patterns of expression in the respiratory epithelium also influence the activity of antioxidant enzymes, skewing the balance between the two redox pathways.
Moreover, many researchers believe that DNA repair capacity may play an important role in susceptibility to lung cancer. CYP1A1, an enzyme of the cytochrome P450 family, plays a role in the development of EGFR mutations, hence altering the risk profile of lung cancer among smokers. Other modulators of risk in lung cancer include diet and gender. Dietary factors function as epigenetic modulators of lung cancer susceptibility. In several case–control studies, defective detoxification and defective repair of genetic damage were associated with increased individual susceptibility to lung cancer. Food constituents, such as those found in fruits and specific vegetables, appear to afford marked protection to individuals with limitations in their detoxification capacity. This relationship between diet and lung cancer has been explored extensively and is being used as a basis for the development of preventive approaches to lung cancer.
Vitamins and Micronutrients
Specific micronutrients have been associated with a lower risk of lung cancer, including vitamin E, selenium, isothiocyanates, polyphenols, betaine, and choline. A number of large randomized trials have sought to delineate which compounds in the human diet may be responsible for the protective effects. Among heavy smokers, a diet high in red meat consumption and with low adherence to the so-called Mediterranean diet has been found to be associated with increased risk of lung cancer. Conversely, a diet high in vegetable fats and fiber was shown to reduce lung cancer incidence among heavy smokers. The role of selenium has been investigated for preventing lung cancer among patients with selenium deficiency. A trial that sought to prevent SPTs after primary skin cancer in a selenium-poor population in Arizona showed a 34% reduction in lung cancer incidence, and because of this success, the role of selenium as a chemoprotective agent was explored in other malignancies. The Selenium and Vitamin E Cancer Prevention Trial was conducted to study the effect of selenium on prostate cancer incidence. In this trial, 32,400 men were randomly assigned to receive oral selenium, vitamin E, or selenium plus vitamin E as compared with placebo in a double-blind fashion and were monitored for a median of 5.46 years. The trial showed no benefit of selenium or vitamin E, or the combination, to confer any protection against prostate cancer. A phase III prevention study was published in 2013 by the Eastern Cooperative Oncology Group in which patients with resected stage I NSCLC were randomly assigned in a 2:1 ratio to receive selenium methionine at 200 μg/day or placebo. In this trial, selenium failed to demonstrate any objective benefit compared with placebo in protecting against the development of lung SPTs.
However, the Eastern Cooperative Oncology Group study was initiated without preliminary studies, and the recommendation must be to continue to build biomarker-driven, targeted, phase II approaches that appropriately pursue modulation of a crucial biomarker, followed by confirmation, before proceeding with large-scale studies.
Initially, researchers found considerable epidemiologic evidence for the potency of carotenoids and retinoids in reducing cancer risk in general, and lung cancer risk in particular. In fact, the original definition of chemoprevention as “attempts to reverse, suppress, and prevent carcinogenic progression to overt cancer” was based on experimental work showing that vitamin A analogs were capable of reversing or preventing epithelial carcinogenesis in animal experiments.
Early chemoprevention trials included broad populations of individuals who were at risk because of their exposure to tobacco and/or asbestos. Others trials focused on specific high-risk populations, such as uranium miners. Thus, with several decades of epidemiologic and dietary investigation augmented by various experimental systems, a number of compounds, including retinoids and carotenoids, were found to be capable of reversing cell damage. Considerable effort was invested in testing this approach in human populations. The trials focused on broad patient populations, including individuals at very high risk. In other words, studies included individuals with known premalignancy of the airway and patients who already had a primary tobacco-related cancer, because these cohorts were known to have extraordinary risk for developing an SPT and therefore offered a more efficient approach for evaluating candidate chemoprevention agents.
Status of Lung Cancer Screening and its Relationship to Chemoprevention
After decades of disappointing results, a robust approach to detect and cure early lung cancer has finally emerged. The National Lung Screening Trial (NLST)—the most expensive screening trial ever conducted by the National Cancer Institute—found that the use of low-dose CT (LDCT) in at-risk populations was associated with a significant reduction in lung cancer mortality. Based on this finding and related data, LDCT screening is now recommended by the USPSTF. Humphrey et al. outlined the underpinnings of the USPSTF draft statement on lung cancer screening in individuals at high risk of lung cancer and summarized the benefits and potential harms of this new screening approach. From a review of this synthesis, a number of evidence-based conclusions emerge that clarify important issues about this new public health service as we begin national dissemination of LDCT screening.
To begin, no strict definition of LDCT exists; it is typically considered to use 10% to 30% of the dose of radiation applied in a standard, noncontrast CT. LDCT has been demonstrated to be as accurate as standard-dose CT for detecting solid pulmonary nodules in most adults. In the NLST, acceptable chest CT screening was accomplished at an overall average effective dose of less than 2 millisievert (mSv), which is considerably less than the average effective dose of 7 mSv used for a typical standard-dose chest CT.
The use of low-dose radiation for screening reflects the context of this imaging application. In a physician’s office, an individual presenting with the appropriate history combined with signs or symptoms of lung cancer is much more likely to have lung cancer than is an asymptomatic individual participating in a lung cancer screening effort. With screening, the balance of risks and benefits is much narrower than in the standard setting of symptom-detected cancer, and so defining the optimal approach to the LDCT process with the greatest efficiency and quality while minimizing costs and harms is the fundamental challenge as we move toward national implementation of lung cancer screening.
The National Comprehensive Cancer Network (NCCN) has provided a useful source of information about how to approach this LDCT screening process. An example of the management and follow-up for one set of findings after LDCT screening is shown in Fig. 9.1 .

NCCN proposes that LDCT screening requires sophisticated multidetector CT scanners and analytic software, professional physicists and staff who can certify equipment and perform studies to a consistent standard at acceptable radiation exposures, qualified radiologists who use standardized terminology and standardized interpretation, appropriate guidelines, reliable communication with primary care physicians, medical environments that can absorb patients who require ongoing management, and the responsibility of tracking screened individuals and documenting outcomes.
NCCN has stratified risk groups that may be eligible for LDCT screening ( Table 9.1 ). The highest-risk group mirrors the eligibility criteria of the NLST, but the lower-risk strata still include people who may develop lung cancer but in whom the cost-to-benefit ratio may be narrower. NCCN also has a patient summary for lung cancer screening, which is an excellent source of objective information for the clinician to guide discussions with potential screening candidates on topics such as who is an appropriate candidate for LDCT screening and what the likely risk is relative to benefit.
Risk Level | Risk Factors | ||
---|---|---|---|
High risk b | Age 55–74 years and 30-pack/year history of smoking and smoking cessation <15 years (category 1) c | or | 20-pack/year history of smoking and one additional risk factor (including radon exposure, cancer history, family history of lung cancer, history of lung disease, and occupational exposure to lung carcinogens such as asbestos and diesel fumes; category 2B) c |
Moderate risk d | Age 50 years and 20-pack/year history of smoking | or | Second-hand smoke exposure (no additional risk factors) e |
Low risk d | Age <50 years | and/or | <20 pack/year history of smoking |
a Moderate-risk and low-risk patients are not recommended for screening.
b Screening is recommended for the high-risk groups in the NCCN Guidelines for Lung Cancer Screening.
c Category 1: Based on high-level evidence, there is uniform NCCN consensus that the intervention is appropriate. Category 2B: Based on lower-level evidence, there is NCCN consensus that the intervention is appropriate.
d Screening with LDCT is not recommended for the moderate-risk and low-risk groups in the NCCN Guidelines for Lung Cancer Screening.
e Risk factors include radon exposure, cancer history, family history of lung cancer, history of lung disease, and occupational exposure to lung carcinogens (e.g., asbestos, diesel fumes).
An important topic that frequently arises when considering the benefits of screening is the concept of overdiagnosis. Overdiagnosis occurs when a cancer detected during screening does not behave in a lethal fashion, such that an individual may die with, not from, a screen-detected cancer. The USPSTF recently commented on the extent of overdiagnosis with chest x-ray screening in the Prostate, Lung, Colorectal, and Ovarian (PLCO) screening trial. Chest x-ray screening was the initial basis of concern for overdiagnosis in lung cancer screening. In the PLCO trial, among participants at risk for lung cancer due to heavy tobacco exposure, the cumulative incidence of lung cancer after 6 years of follow-up was the same in both the chest x-ray and usual-care groups (606 per 100,000 person-years vs. 608 per 100,000 person-years, respectively; relative risk, 1.00; 95% confidence interval [CI], 0.88–1.13). These results indicate a very small influence of overdiagnosis in this setting, and the PLCO findings suggest that overdiagnosis is not a major confounder in evaluating the benefit of lung cancer screening.
However, many other questions remain unanswered as we consider the implementation of LDCT screening because many misconceptions exist about this new service. One of the most important concepts is understanding the difference between providing screening care for a patient at risk for lung cancer and the more typical situation of treating a symptomatic patient suspected to have lung cancer. The risk–benefit considerations in these two settings are very different, and these differences mandate distinct management approaches.
Regarding other misconceptions, the core design assumption of the NLST, which evaluated more than 53,000 current or former smokers at a cost in excess of $250 million, was widely vetted. The consensus that emerged was that a target reduction in mortality of 20% with LDCT compared with chest x-ray outcomes would constitute compelling evidence of an objective screening benefit. An analysis of the full benefit of LDCT would have been much more expensive and require considerably more time to complete. Therefore it is not surprising that a more recent analysis of NLST outcomes with a rederived eligibility risk model constructed from PLCO case outcomes resulted in a more efficient rate of lung cancer detection. In that report, the mortality reduction was 30%, compared with the 20% benefit reported in the NLST. This example demonstrates how the LDCT screening process can be improved, and the reanalysis of the NLST data set underscores how valuable this data resource is in allowing process improvement for LDCT screening. As screening becomes implemented, it will be essential to continue to build screening registries from the data of as many screening individuals as possible so that continuous process improvement can be sustained.
LDCT screening does entail a range of possible harms. A 2012 review article from a joint society initiative communicated reservations about the balance of risks and benefits of lung cancer screening. The investigators reported that “uncertainty exists about the potential harms of screening and the generalizability of results.” By contrast, a comprehensive synthesis of the issues with screening management was published by the USPSTF in 2013 and conveyed a more optimistic perspective regarding how refined management approaches have improved the process of lung cancer screening. The USPSTF provides an excellent resource for guiding discussions to inform potential screening individuals about risks and benefits of this service.
Results of the NLST and Other Clinical Trials Are Not Equal
Although disparate views exist regarding the strength of the evidence supporting LDCT, data synthesis from the comprehensive USPSTF analysis helps clarify this dialogue with its clear support for the strengths of this new approach. At the time of publication, the NLST was the only completed, fully powered, randomized, lung cancer screening trial reported, and no comparable, adequately powered trial was currently under consideration. Comparisons of the mortality reduction reported in the NLST relative to the results of two small, randomized, European trials are not helpful because the latter studies were not adequately powered to give a reliable assessment of the mortality reduction of LDCT. Although ongoing trials will generate interesting and important cost-related and other data that are complementary to those of the NLST, no existing trials will have sufficient study power to supersede the positive conclusion of the NLST relative to the end point of mortality reduction. The largest of the ongoing trials is the Dutch-Belgian Randomized Lung Cancer Screening Trial (Dutch acronym NELSON), a study being conducted by a consortium of Dutch and Belgian investigators. Preliminary data from NELSON can be compared with follow-up data from the NLST91 ( Table 9.2 ). As shown, the cancer detection rates are comparable and the stage I detection rates are similar as well, so a major reduction in benefit with LDCT in the NELSON trial is not expected.
Study | No. of Cancers/Total Screened (%) | Stage I/All Detected Cancers (%) | ||
---|---|---|---|---|
Round 1 | Round 2 | Round 1 | Round 2 | |
NLST | 168/24,715 (0.67) | 211/24,102 (0.87) | 104/165 (63) | 141/204 (69) |
NELSON | 40/7289 (0.5) | 57/7289 a (0.8) | 42/57 (73.7) | — |
a NELSON round 2/3 data were presented together, reflecting the study design.
From the perspective of US national policy, the NLST data represent the standard criterion showing that LDCT screening can reduce mortality from lung cancer. In the wake of the additional NLST follow-up results, the most relevant contribution of the NELSON trial may be the quality of the ancillary studies, such as the eventual economic analysis. The NELSON investigators embedded more advanced clinical screening management provisions because this trial started several years after the start of the NLST.
Meeting or Exceeding the Favorable Outcomes Reported in the NLST
Going forward, a crucial issue is whether the reported benefits of LDCT screening can be met or exceeded when LDCT is implemented nationally as a routine clinical service. This is a complex issue involving a number of variables that are all crucial in determining the overall benefit of this approach. For example, the risk of lung cancer after heavy smoking persists, despite smoking cessation, and remains elevated as long as the former smoker lives. Therefore ongoing screening beyond the two rounds evaluated in the NLST could further improve the mortality benefit of lung cancer screening beyond the 20% threshold. Two reports have suggested that sustained annual screening may reduce mortality from lung cancer by 40% to 60% under different screening scenarios. LDCT has several robust features that enhance its performance as a cancer screening tool. Indeed, the rapid refinement of CT image resolution has resulted in routine detection of progressively smaller primary lung cancers. This evolution improves patients’ outcomes for two reasons. First, smaller tumors are associated with better cancer-specific outcomes. Second, smaller tumors are likely to be amenable to treatment with minimally invasive thoracic surgery, a new surgical approach associated with a better quality of life, better compliance with adjuvant therapy, and fewer postoperative complications.
In the NLST, surgical clinical care was not specified by any optimized protocol and was typically not delivered in a center selected for excellence in thoracic surgical care. At the time of the NLST, minimally invasive surgery was used in only a minority of the cancers detected during the study. For these reasons, thoughtful national implementation coupled with current best practice could mean that LDCT screening meets or even exceeds the favorable results reported in the NLST. Definitive data for the optimal approach to surgical management in the screening setting do not exist. However, many centers of excellence have reported favorable surgical outcomes using minimally invasive approaches, generally with video-assisted thoracoscopic surgery. A retrospective review of 347 thoracic resections performed in the setting of a lung cancer screening program demonstrated that long-term (10-year) results of sublobar resection were equivalent to those of lobectomy for clinical stage 1A lung cancers. In addition to these excellent results, sublobar resection preserves greater amounts of well-functioning lung tissue.
The Lung Cancer Alliance, an advocacy group, has proposed the mechanism of the Lung Cancer Screening Framework, which encourages institutions providing screening services to employ best practices for screening, including minimally invasive surgical techniques, so that the quality of screening services is maintained at a high level. To validate that institutions are successful in their screening management services, the framework process also mandates that participating institutions routinely report relevant screening outcomes and complication rates, so that potential screening individuals can make informed decisions about where they choose to receive their screening care.
Screening Interval and Selection of Candidates
Questions regarding the optimal interval for the frequency of LDCT screening have been cited as a justification for delaying national implementation. A more efficient approach for defining candidates for LDCT would address much of the uncertainty cited with regard to candidate selection while providing timely access for patients most likely to benefit from LDCT screening. Given the complexity of predicting lung cancer risk, it is unlikely that a one-size-fits-all screening recommendation would suffice moving forward; however, new risk-stratification tools have been shown to provide more robust discrimination of lung cancer risk.
The context for lung cancer screening is unique because tobacco exposure is such a powerful determinant of risk. In 2012, a large British meta-analysis that included data from more than 250,000 individuals resulted in the development of a tool that was very robust in stratifying risk using only the patient’s history of tobacco exposure. This approach is consistent with a report by Tammemägi et al. that modeled a better screening outcome when using a more refined tool for cohort identification. Although more comprehensive molecular or genetic models are being developed, the risk tool based on tobacco exposure history would be a logical candidate to use when starting the national screening process and evaluating the generalizable benefit of LDCT screening. Professional societies have suggested that it would be logical to extend LDCT screening to other target cohorts whose level of risk is similar to that of the NLST target population. For example, screening could be recommended for individuals based on age, history of tobacco exposure, and other factors as determined by that risk tool, all of which might define a screening cohort equivalent to the validated risk strata found among NLST participants. Perhaps further work with the PLCO risk model could prospectively classify risk of lung cancer relative to the risk strata studied in the NLST as a normative tool for comparing new risk-stratifying tools going forward.
Opportunities to Improve LDCT Screening
Since the start of the NLST in 2002, substantial improvements have been made in several areas, including the imaging resolution of LDCT, tools to discriminate high-risk populations, efficiency of diagnostic workup algorithms, and the morbidity of curative thoracic surgical procedures. The dose of medical radiation required for LDCT has also decreased. The LDCT screening setting, which, for now, involves annual follow-up, provides an opportunity to manage tobacco cessation at each annual encounter. The intensity of the cessation strategy can be tailored for the persistent smoker, and this new screening management setting therefore offers a new platform for personalized efforts at smoking cessation. More effective integration of screening and smoking cessation could enhance the public health benefit of this new service. The strength of this growing body of information supporting the safety and effectiveness of LDCT screening, as well as the growing number of endorsements by professionals, has led insurers, such as WellPoint and Anthem, to provide LDCT as a covered service. Third-party coverage through Medicare in the United States was adapted in 2015.
Improvements in the Approach to Screening and LDCT Imaging
Since the inception of the NLST a decade ago, the approach to lung cancer screening that was built into the NLST protocol has improved substantially, yet these improvements—which could facilitate LDCT implementation by reducing potential harms and costs—have not been considered in evaluating the efficacy of CT screening. For example, multidetector scanners were used in the NLST because they are faster than earlier scanners and allow full visualization of lung fields within a single breath hold. The use of such scanners results in fewer CT image artifacts and allows more comprehensive analysis of the lung than that achievable with chest x-rays. However, recently developed CT scanners offer faster image acquisition, which may translate into technically better-quality studies with less motion artifact, and the image quality has continued to improve. These improvements lower both the risks and costs of imaging. All of these factors are pertinent in the public health implementation of LDCT as a national screening resource.
Changes in Nodule Evaluation and Diagnostic Workup
Awareness is variable on the importance of the new findings regarding the approach to nodule evaluation. Published reports have outlined considerable refinement in the clinical management of the screening process since the initiation of the NLST, which did not mandate a specific approach to nodule evaluation. Yankelevitz et al. were the first to report that clinically important lung nodules could be identified by restricting the diagnostic workup to suspicious nodules that showed significant growth over a defined period. This interval-growth approach to the diagnostic workup was incorporated into the design of the NELSON trial and resulted in a favorable workup rate of 12% for the diagnosis of invasive nodules, with a diagnostic sensitivity of 95% and a specificity of 99% for LDCT. More recently, this interval-growth criterion for suspicious nodules was used prospectively in a cohort of 4700 patients undergoing screening at the Princess Margaret Hospital; only 3% of the patients were required to undergo an invasive workup, and the false-positive diagnostic rate was 0.42%. The validity of these findings is supported by a recent retrospective analysis of baseline lung cancer cases accrued in the International Early Lung Cancer Action Project. In this analysis, changing the threshold of nodule size for a lung cancer diagnostic workup from 4–5 mm to 7–8 mm was associated with timely diagnosis of early-stage lung cancer while significantly reducing the frequency of false-positive results. Implementation of this approach could improve the efficiency of LDCT screening by reducing the costs and harms of invasive diagnostic workups within the baseline screening process. The NCCN has been integrating the evolving research information into its management recommendations for screening (see Fig. 9.1 ). This information will allow more consistent management of LDCT, with expectations of more favorable outcomes.
Further Opportunities Associated With LDCT Screening
A crucial opportunity to optimize the benefit of screening is to integrate smoking cessation into lung cancer screening. Although integration of LDCT screening into smoking cessation has been reported with mixed success, to date little research has been done to optimize smoking cessation within the setting of recurrent screening. Some authors have characterized the screening encounter as a so-called teachable moment for a dialogue about smoking cessation, and the cost efficiency of this integration is projected to be extremely favorable. Furthermore, every subsequent annual LDCT screening for a persistent smoker is an opportunity to explore more personalized or intensive measures for smoking cessation. Increasing success with smoking cessation would not only improve the inherent cost efficiency of the LDCT screening process relative to lung cancer outcomes, but would also accrue to the other well-validated health and economic benefits related to smoking cessation. Through new mechanisms required by the Centers for Medicare and Medicaid Services, reimbursement for LDCT screening services could be linked to demonstrating compliance with national quality measures for these services, especially with regard to risk stratification and smoking cessation.
A related consideration with LDCT is the unprecedented potential to evaluate the status of other tobacco-related diseases. For example, recent reports have shown that coronary calcium analysis can be derived from LDCT images and may be a useful stratification tool for the risk of coronary artery disease. Furthermore, an LDCT assessment of lung injury could serve as a metric of risk for progression of COPD. Indeed, patients with tobacco exposure participating in lung cancer screening are known to experience a significant comorbid risk of COPD and cardiovascular disease. With further research, the opportunity exists to simultaneously evaluate for the three major host consequences of tobacco exposure—coronary artery disease, COPD, and cardiovascular disease—with only LDCT. These diseases represent three of the leading causes of premature death in our society. The emerging setting of LDCT screening can therefore provide a useful window into the preclinical phase of three major chronic diseases, so the time is ripe to explore this important opportunity.
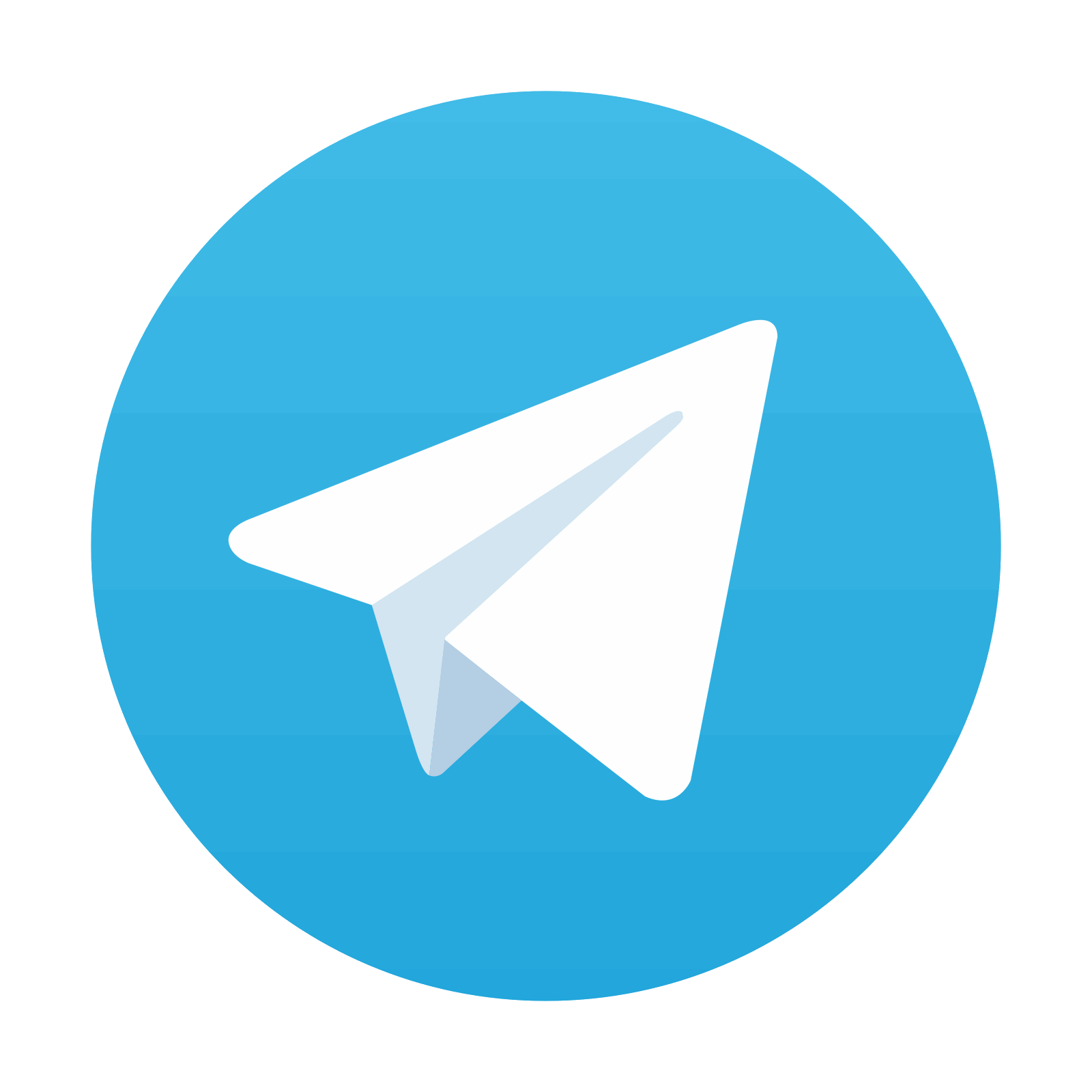
Stay updated, free articles. Join our Telegram channel

Full access? Get Clinical Tree
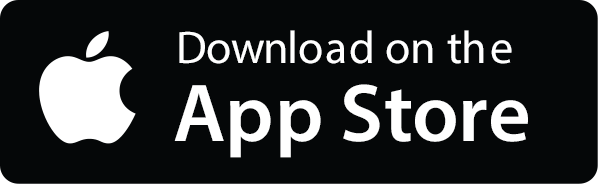
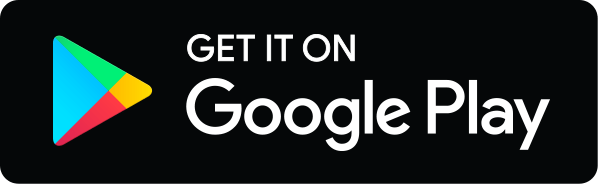