Background
The aim of this study was to evaluate changes in three-dimensional (3D) speckle-tracking–derived myocardial strain during ischemia.
Methods
Twenty patients referred for percutaneous coronary intervention were studied (mean age, 65 ± 11 years; mean left ventricular ejection fraction, 56 ± 7%). Of the 20 study patients, 12 had severe left anterior descending coronary artery stenosis. A full-volume apical view using multibeat 3D modality and two-dimensional (2D) apical views (four chamber, two chamber, and three chamber) were recorded during coronary occlusion before wall motion abnormalities. After percutaneous coronary intervention, ultrasound contrast agent was selectively delivered through the target lesion to delineate ischemic and peri-ischemic segments (ischemia-adjacent segments). Strain values derived from 2D and 3D speckle-tracking echocardiography were compared in ischemic and nonischemic segments.
Results
Despite no changes in wall motion and 2D left ventricular ejection fraction (56 ± 7% vs 56 ± 7%), global longitudinal strain by 2D imaging was impaired during percutaneous coronary intervention (−16 ± 3% vs −14 ± 3%, P = .01). Similar changes were observed for all 3D strain components: −11 ± 16% for longitudinal (−15 ± 4% vs −13 ± 4%, P = .03), −13 ± 25% for circumferential (−15 ± 4% vs −12 ± 4%, P = .02), −12 ± 16% for area (−25 ± 5% vs −22 ± 6%, P = .009), and −12 ± 25% for radial global strain (39 ± 12% vs 33 ± 12%, P = .04). During coronary occlusion, 2D longitudinal peak strain and all 3D peak strain components decreased in ischemic segments, while no changes was observed in nonischemic segments. In peri-ischemic segments, only 3D longitudinal and area strain were impaired during ischemia.
Conclusions
Changes in myocardial deformation related to ischemia can be characterized by 3D speckle-tracking echocardiography before the stage of wall motion abnormality.
Wall motion analysis is determinant for the decision to rule out coronary artery disease in chest pain units. In clinical practice, two-dimensional (2D) echocardiography is used to identify high-risk patients with wall motion abnormalities at rest and/or during stress echocardiography. However, the accuracy of visual wall motion analysis depends on operator experience. In addition, visual analysis is based mainly on changes in radial motion that may lack sensitivity for detecting ischemia compared with longitudinal motion. Several authors have suggested that speckle-tracking echocardiography (STE) might be used in addition to wall motion analysis to evaluate ischemia during stress echocardiography. STE provides a robust assessment of myocardial deformation, highly reproducible for longitudinal strain, that correlates with cardiac magnetic resonance imaging and sonomicrometry. In a recent study, Ng et al . demonstrated that global longitudinal strain by STE in combination with expert wall motion interpretation improves the accuracy of stress echocardiography in patients with normal ejection fraction. However, 2D strain is inherently limited by 2D imaging, which prevents visualization of the whole myocardium, and in patients with resting wall motion abnormalities, assessment of changes in strain value is challenged by the difficulty of acquiring similar apical views during stress echocardiography. Recently, three-dimensional (3D) STE has been proposed to overcome 2D limitations, but its accuracy and feasibility for identifying myocardial ischemia in human have not been evaluated. In the present study, we assessed changes in myocardial deformation evaluated using 2D and 3D STE during percutaneous coronary intervention (PCI).
Methods
Study Population
We considered 23 patients with severe proximal single-vessel coronary disease who were referred for PCI between November 2011 and June 2012. Patients with histories of acute coronary syndromes and chronic coronary occlusion were not considered. Of the 23 patients, two were excluded for poor 3D acoustic windows while lying in the supine position, and one patient was excluded for PCI-related wall motion abnormality during echocardiographic acquisition. The 20 remaining patients were included, and all had one proximal severe coronary stenosis with myocardial ischemia supported by stress testing. All patients provided informed written consent, and our local ethics committee approved the study.
Data Acquisition during PCI
Coronary angiography was performed using radial access, and all patients received dual-antiplatelet therapy (aspirin and clopidogrel) before PCI and a bolus of unfractionated heparin during PCI. Hemodynamic conditions were carefully monitored during the procedure to avoided load-related strain changes. All echocardiographic data were synchronized to the electrocardiogram, and acquisition was performed during breath hold. Comprehensive 2D and 3D echocardiographic data were acquired before and during PCI using a Vivid E9 system (GE Vingmed Ultrasound AS, Horten, Norway). Before and during PCI, 2D grayscale apical views (four chamber, two chamber, and three chamber) using high frame rates (>60 frames/sec) and a 3D full-volume data set from the apical view using multibeat modality (mean, 20 ± 2 volumes/sec) were successively acquired using the same matrix-array transducer (4V-D 4D Cardiac Probe; 1.5–4 MHz) to shorten the recording time. The order of recording was standardized (2D, then 3D) and not randomized. To assess changes in strain before wall motion abnormality, echocardiographic data were acquired 10 seconds after the first balloon inflation. After PCI, 2 mL of contrast ultrasound agent (SonoVue; Bracco SpA, Milan, Italy) was selectively delivered through the PCI site using an over-the-wire balloon to delineate the corresponding vascular territory. This territory was considered as ischemic during coronary occlusion and referred to as “ischemic -segments.”
Echocardiographic Data Analysis
Two-dimensional and 3D data were analyzed offline using EchoPAC version 110.1.2 (GE Vingmed Ultrasound AS) by an experienced investigator blinded to the distribution of ischemic segments. Three-dimensional acquisition with contrast injection used to defined ischemic segments was analyzed by an independent investigator blinded to previous results. Left ventricular volumes and left ventricular ejection fraction (LVEF) were quantified from 2D and 3D data. Wall motion at rest and during PCI was visually assessed using the nine-slice short-axis views obtained from the 3D volume data set. Patients with changes in wall motion score were excluded from analysis ( n = 1). Speckle-tracking analysis was performed using dedicated wall motion tracking software: Automated Function Imaging for 2D imaging and 3D Auto Left Ventricular Quantification for 3D imaging (both from GE Vingmed Ultrasound AS). Briefly, automatic endocardial delineation was obtained after manual positioning of the end-systolic endocardial border mitral plane and left ventricular apex. The region of interest position and size were adjusted manually to provide optimal frame-by-frame wall tracking throughout the cardiac cycle. For strain processing, the peak of the R wave on the electrocardiogram was used as the reference time point for end-diastole. Segments with poor-quality tracking or providing aberrant curves despite manual adjustment were discarded from analysis. Longitudinal strain was computed from 2D and 3D STE and circumferential strain from the 3D data set. Radial and area strain were calculated from circumferential and longitudinal 3D strain. Global longitudinal strain was obtained by averaging regional strain curves (16-segment model for 2D STE and 3D STE). For all strain components, peak systolic and time to peak strain were defined using regional strain curves. For the regional analysis, segments were analyzed as ischemic, peri-ischemic, or nonischemic according to contrast ultrasound injection. Segments with complete or partial ultrasound contrast enhancement on visual analysis were considered to depend on the vascularization of the dilated coronary artery and were referred to as ischemic. Adjacent segments surrounding ischemic segments without contrast enhancement were defined as peri-ischemic, and the remaining segments were defined as nonischemic.
Statistical Analysis
Changes in both 3D and 2D strain by STE during PCI were analyzed. Continuous variables with normal distributions are expressed as mean ± SD. Dichotomous data are expressed as percentages. A paired comparison was used to evaluate changes in strain values during PCI. Nominal variables were compared using either χ 2 or Fisher’s exact tests. Reproducibility for 2D longitudinal strain and all 3D components was performed 2 months after the first measurement by a second independent observer in 10 random patients. Reproducibility was expressed as the absolute difference expressed as the percentage of the mean of repeated measurements and using interclass correlation coefficients. Two-tailed P values < .05 were considered statistically significant.
Results
Of the 20 patients included in the analysis (mean age, 65 ± 11 years; 80% men), seven had histories of angina with previous PCI, and none had valvular disease ( Table 1 ). All patients were in sinus rhythm, 85% were treated with β-blockers, and no changes in load conditions were observed. PCI was performed in the left anterior descending coronary artery in 12 patients, and balloon inflation duration averaged 51 ± 14 sec (range, 30–60 sec).
Variable | Value |
---|---|
Age (y) | 65 ± 11 |
BMI (kg/m 2 ) | 28 ± 4 |
Men | 16 (80%) |
Smokers | 12 (60%) |
Diabetes | 9 (45%) |
Hypertension | 13 (65%) |
Dyslipidemia | 11 (55%) |
β-blocker use | 17 (85%) |
SBP (mm Hg) | 129 ± 18 |
HR (beats/min) | 63 ± 8 |
Creatinine (μmol/L) | 82 ± 21 |
Occlusion duration (sec) | 51 ± 14 |
LAD coronary artery PCI | 12 (60%) |
Non-LAD coronary artery PCI | 8 (40%) |
Feasibility
Two-dimensional frame rate (53 ± 3 frames/sec) and 3D volume rate (20 ± 2 volumes/sec) were unchanged during PCI ( Table 2 ). Analyzable segments before PCI accounted for 97% (311 of 320) by 2D STE and 94% (320 of 340) by 3D STE and 98% and 90%, respectively, during PCI. Nonanalyzable segments were located mainly in the anterior wall (45%).
Variable | Baseline | During PCI | P |
---|---|---|---|
2D global parameters | |||
LVEF (%) | 56 ± 7 | 56 ± 7 | NS |
Global longitudinal strain (%) | −16 ± 3 | −14 ± 3 | .01 |
Heart rate (beats/min) | 63 ± 8 | 61 ± 11 | .42 |
Temporal resolution (images/sec) | 53 ± 3 | 53 ± 3 | 1.00 |
3D global parameters | |||
LVEF (%) | 52 ± 6 | 49 ± 4 | .002 |
Global longitudinal strain (%) | −15 ± 4 | −13 ± 4 | .03 |
Global circumferential strain (%) | −15 ± 4 | −12 ± 4 | .02 |
Global area strain (%) | −25 ± 5 | −22 ± 6 | .009 |
Global radial strain (%) | 39 ± 12 | 33 ± 12 | .04 |
Heart rate (beats/min) | 63 ± 8 | 61 ± 11 | .42 |
Temporal resolution (images/sec) | 20 ± 2 | 20 ± 2 | 1.00 |
Global Changes in Myocardial Function during PCI
Left ventricular ejection fraction by 3D imaging decreased during PCI (52 ± 6% vs 49 ± 4%, P = .002), while no change was observed for 2D LVEF ( Table 2 ). Changes in global longitudinal strain during PCI averaged −10% (−16 ± 3% vs −14 ± 3%) for 2D and −11% for 3D longitudinal strain (−15 ± 4.0% vs −13 ± 4.3%). A good correlation was reported between global longitudinal strain by 2D and 3D ( r = 0.70, P < .0001; Figure 1 ). Changes in global strain averaged −13% for circumferential strain and −12% for radial and area strain ( Figure 2 ).


Regional Strain during PCI
Overall, 100 of 320 segments were defined as ischemic, 109 segments surrounding ischemic segments as peri-ischemic, and 111 as nonischemic segments (remote). Ischemic segments were supplied mainly by the left anterior descending coronary artery (79% [79 of 109]). During PCI, wall motion score index calculated from the nine-slice short-axis view of the 3D acquisition was unchanged (1.09 ± 0.2). In ischemic segments, peak longitudinal strain by 2D STE was impaired (−14 ± 8% vs −17 ± 7, P = .004), and time to peak strain was prolonged (422 ± 96 vs 387 ± 113 msec, P = .0001) during PCI, while no change was observed in remote and peri-ischemic segments ( Table 3 ). Interestingly, longitudinal and area peak strain by 3D STE were impaired both in ischemic and peri-ischemic segments, while circumferential and radial peak strain decreased only in ischemic segments during PCI ( Figure 3 ). In contrast to 2D STE, no 3D speckle-tracking echocardiographic strain components showed changes in time to peak strain during PCI. Typical changes in 3D strain curves are showed in Figure 4 .
Variable | Before PCI | During PCI | P |
---|---|---|---|
Ischemic segments | |||
2D peak longitudinal strain (%) | −17 ± 7 | −14 ± 8 | .004 |
Time to peak strain (msec) | 387 ± 113 | 422 ± 96 | .0001 |
3D peak longitudinal strain (%) | −16 ± 9 | −14 ± 8 | .02 |
Time to peak strain (msec) | 400 ± 123 | 403 ± 152 | .9 |
3D peak circumferential strain (%) | −17 ± 8 | −14 ± 7 | .02 |
Time to peak strain (msec) | 406 ± 109 | 388 ± 145 | .33 |
3D peak area strain (%) | −27 ± 11 | −23 ± 13 | .01 |
Time to peak strain (msec) | 401 ± 98 | 399 ± 1118 | .85 |
3D peak radial strain (%) | 41 ± 19 | 36 ± 20 | .01 |
Time to peak strain (msec) | 420 ± 135 | 400 ± 122 | .26 |
Peri-ischemic segments | |||
2D peak longitudinal strain (%) | −17 ± 6 | −15 ± 8 | .14 |
Time to peak strain (msec) | 402 ± 117 | 392 ± 89 | .19 |
3D peak longitudinal strain (%) | −17 ± 9 | −15 ± 9 | .01 |
Time to peak strain (msec) | 436 ± 152 | 409 ± 148 | .15 |
3D peak circumferential strain (%) | −15 ± 6 | −14 ± 6 | .15 |
Time to peak strain (msec) | 416 ± 164 | 398 ± 146 | .82 |
3D peak area strain (%) | −27 ± 9 | −24 ± 11 | .009 |
Time to peak strain (msec) | 401 ± 99 | 387 ± 114 | .57 |
3D peak radial strain (%) | 44 ± 30 | 38 ± 27 | .11 |
Time to peak strain (msec) | 400 ± 87 | 427 ± 156 | .12 |
Remote segments | |||
2D peak longitudinal strain (%) | −15 ± 9 | −15 ± 9 | .30 |
Time to peak strain (msec) | 398 ± 137 | 415 ± 93 | .39 |
3D peak longitudinal strain (%) | −15 ± 8 | −14 ± 8 | .30 |
Time to peak strain (msec) | 411 ± 161 | 411 ± 166 | .90 |
3D peak circumferential strain (%) | −15 ± 7 | −14 ± 7 | .70 |
Time to peak strain (msec) | 380 ± 118 | 383 ± 121 | .97 |
3D peak area strain (%) | −25 ± 10 | −24 ± 10 | .70 |
Time to peak strain (msec) | 404 ± 108 | 398 ± 106 | .40 |
3D peak radial strain (%) | 38 ± 20 | 35 ± 22 | .47 |
Time to peak strain (msec) | 413 ± 134 | 401 ± 108 | .35 |
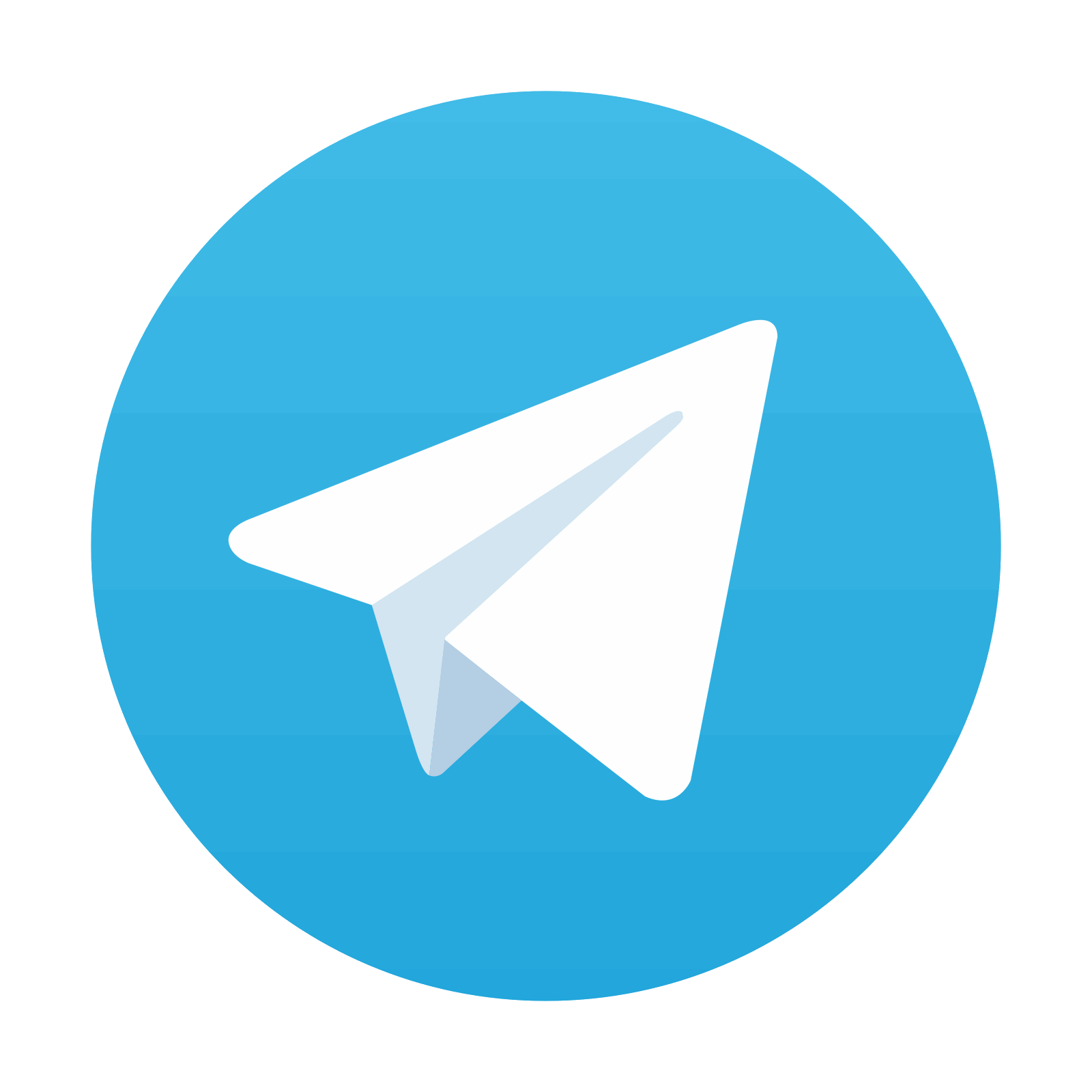
Stay updated, free articles. Join our Telegram channel

Full access? Get Clinical Tree
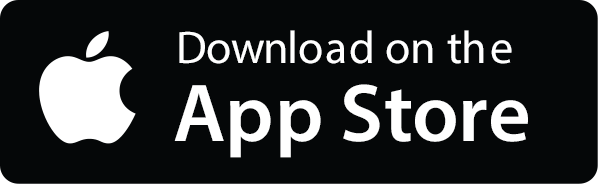
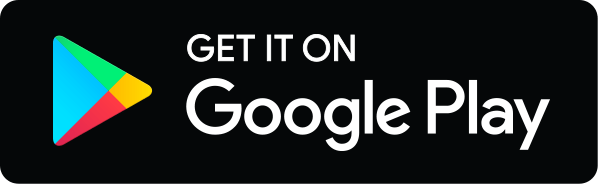
