Background
Pediatric cancer survivors who have been exposed to anthracycline (ANT) chemotherapy are an ever increasing population at risk for premature cardiac disease. Studies have shown that ANT is associated with impaired left ventricular (LV) myocardial deformation, but this has not been shown to be associated with traditional echocardiographic measures of LV systolic dysfunction. The aim of this study was to test the hypothesis that changes in LV longitudinal peak systolic strain (LPSS) would correlate with parameters of LV systolic dysfunction.
Methods
This study included 19 prospectively enrolled pediatric patients receiving ANT (mean dose, 296 ± 103 mg/m 2 ) and 19 controls matched for age, gender, and body surface area. For ANT patients, echocardiography was performed at baseline, mid, and final treatment points (0, 4, and 8 months). Standard echocardiographic parameters and two-dimensional speckle tracking–derived longitudinal strain parameters were obtained and compared with baseline measurements in controls. Associations between changes in LV global LPSS and standard echocardiographic indices were explored.
Results
Within the ANT group, the change in LV global LPSS showed a significant decrease compared with baseline at 4 months (8.7 ± 0.2%, P = .033) and 8 months (9.2 ± 0.3%, P = .015), while the percentage change in ejection fraction (EF) showed a statistically significant decrease at 8 months (4.3 ± 0.1%, P = .044). LV global LPSS was decreased in the ANT group compared with controls at 4 months (18.1 ± 2.5% vs 20.5 ± 1.5%, P = .011) and 8 months (18.1 ± 2.8%, P = .032). Segmental changes in mid and apical LV LPSS average were significantly correlated with change in EF (mid: r = −0.49, β = −0.645, P = 0.039; apical: r = −0.48, β = −0.4126, P = .046).
Conclusions
In adolescents who receive ANT therapy, changes in two-dimensional LV global LPSS precede decreases in EF, and segmental changes in mid and apical LV LPSS suggest an increased likelihood that depressed LV EF will be observed later in follow-up. Two-dimensional speckle tracking–derived LV LPSS is potentially useful in the serial clinical monitoring of ANT cardiotoxicity.
Previous exposure to anthracycline (ANT) chemotherapy is common among pediatric cancer survivors, which places this population at an increasing risk for significant cardiac disease. The frequency of late clinical cardiotoxicity after ANT chemotherapy in children varies from 0% to 16%. Late subclinical cardiotoxicity among childhood cancer survivors is more common, reportedly as high as 57%. With many of these patients now surviving into adulthood, a better appreciation of the effects of ANT chemotherapy on cardiac function is important. Endomyocardial biopsy has been reported as a tool in the monitoring of ANT cardiotoxicity in children and adults but has been used less frequently because of its invasive nature. Because of the inherent radiation exposure, radionuclide techniques are also not usually used for serial follow-up. A noninvasive and reliable test for monitoring ANT cardiotoxicity would be preferable, in particular for children. Transthoracic echocardiography is the most widely used modality for the evaluation of early and late ANT cardiotoxicity.
Importantly, a patient may remain asymptomatic for a long period after ANT chemotherapy, because the physiologic reserve and compensatory mechanisms may maintain normal cardiac function until a threshold level is reached. Echocardiographic surveillance of ANT cardiotoxicity has traditionally been based on left ventricular (LV) ejection-phase indices (shortening fraction and ejection fraction [EF]), myocardial performance index, and changes in LV diastolic filling detected by interrogation of transmitral and pulmonary venous inflow Doppler. From studies conducted primarily in adults, it has been shown that conventional two-dimensional (2D) echocardiographic indices may not accurately predict the onset of congestive heart failure in patients after ANT chemotherapy. Moreover, these indices often denote late findings and may not help with the early identification of myocardial dysfunction. Recent studies in this patient group have stressed the clinical importance of assessing regional myocardial function using strain and strain rate imaging.
Transition from a Doppler-based to a 2D speckle-tracking echocardiography (STE) methodology for strain analysis has improved the reproducibility and ease of use of this technology in most echocardiography laboratories. In 2D STE, tracking of natural acoustic markers (speckles) is performed frame by frame throughout the cardiac cycle, allowing angle-independent analysis of myocardial strain. Recent studies indicate that 2D STE–derived strain and strain rate imaging may serve as reliable tools to quantify systolic and diastolic function.
There are limited data on the analysis of 2D strain using STE in the serial quantitative echocardiographic assessment of children and adults after ANT therapy. Recent reports have shown the association of ANT chemotherapy with impaired LV myocardial deformation, but, LV longitudinal strain has not been analyzed prospectively in this group of patients. Therefore, the primary goal of this study was to investigate how changes in LV longitudinal peak systolic strain (LPSS) in pediatric patients after ANT chemotherapy correlate with conventional echocardiographic markers of LV systolic and diastolic function.
Methods
Subjects
Patients scheduled to receive ANT (doxorubicin, daunorubicin, or idarubicin) chemotherapy were prospectively evaluated. All patients had typical adjuvant chemotherapy without any other additional cardiotoxic medications. Patients with previous ANT chemotherapy or radiotherapy were excluded. All patients were asymptomatic, in normal sinus rhythm, and not on any cardiovascular medications during their serial echocardiographic evaluations. Age-matched and gender-matched patients referred for evaluation of a cardiac murmur who were determined to have normal intracardiac structural anatomy and function were selected as controls. In the ANT group, baseline echocardiographic examinations were performed 24 hours before the first dose of ANT. Subsequent echocardiographic examinations were to be performed at 4 and 8 months after the baseline studies. ANT patients were excluded from the study if they did not meet serial echocardiographic measurement time points. Only patients with LV EFs > 50% and those without any cardiac symptoms were included. Standard echocardiographic indices of LV function and LV longitudinal strain parameters were obtained in all patients and controls using 2D STE. The study was approved by the institutional review boards at the Mayo Clinic and the University of Nebraska Medical Center.
Standard Echocardiographic Assessment
Echocardiographic assessments were obtained using Vivid 7 (GE Healthcare, Milwaukee, WI) ultrasound systems following a standard imaging protocol. Patients were examined in the left lateral decubitus position. Measurements were obtained in the apical planes (four chamber, two chamber, and long axis) and in the parasternal short-axis plane at the level of mitral leaflets. Standard M-mode assessment of the LV parasternal short axis was performed to derive R-R duration, LV end-systolic and end-diastolic dimensions, and septal and inferolateral end-systolic and end-diastolic wall thickness. These dimensions were corrected for body surface area. LV end-diastolic volume and LV end-systolic volume (LVESV) were analyzed in the two-chamber and four-chamber views using the biplane method of disks (modified Simpson’s method) for the measurement of LV EF. LV mass was calculated using the American Society of Echocardiography’s recommended formula for 2D echocardiography. LV mass was indexed by dividing by height (meters) indexed to the power of 2.7. Pulsed-wave Doppler was performed to obtain peak mitral inflow velocities at early (E) and late (A) diastole and the E/A ratio. Tissue Doppler echocardiography was performed with the sample volume positioned at the basal LV free wall at the mitral annular junction to obtain lateral and medial mitral annular systolic and early diastolic myocardial tissue velocities.
Two-Dimensional Speckle-Tracking Echocardiographic Assessment
Two-dimensional STE for the determination of LV strain was performed as reported previously. Apical four-chamber, two-chamber, and long-axis images were used to assess LV longitudinal strain, and these images were stored as raw data at an acquisition frame rate of >80 frames/sec. Online analysis software on the Vivid 7 ultrasound system was used to obtain LV LPSS data as previously detailed. Segmental LV myocardial strain was assessed in 17 segments (six basal, six mid, and five apical segments, including the apical cap) per the American Society of Echocardiography’s 17- segment standardized myocardial echocardiographic nomenclature. We included LV LPSS parameters for basal, mid, apical and global averages. Automatic endocardial tracking was used with manual correction of tracing if necessary in end-systole in the apical two-chamber, apical four-chamber, and long-axis views. Aortic valve closure was determined from the long-axis view, and peak strain was measured at end-systole. Interobserver variability for LV global LPSS and EF was assessed in 15 randomly chosen patients on two separate occasions. Intraobserver measurements in these 15 were repeated by a blinded second reviewer (B.W.E.) and by the primary reviewer (R.K.L.) 1 week after the initial measurement.
Statistical Analysis
All data are presented as mean ± 2 SDs. Differences between the ANT group and controls were analyzed using independent t tests. Individual differences within the ANT group at baseline, 4 months, and 8 months were tested using dependent t tests. To compare relative magnitudes between LV LPSS average and EF using paired t tests, percentage changes normalized from baseline, 4-month, and 8-month measurements were determined. P values <0.05 was considered statistically significant. Changes in LV LPSS were incorporated as independent variables in linear regression to assess for any predictive dependent correlation with changes in EF, lateral and medial mitral annular myocardial tissue velocities, lateral and medial E/E′ ratio, mitral E/A ratio, and cumulative dose of ANT. The sample linear regression coefficient ( r ) was determined by determining the square root of coefficient of determination ( r 2 ). Type 1 error was tested using t statistics in the linear regression model, with P values < .05 as rejecting the null hypothesis (95% confidence interval). Interobserver and intraobserver variability was determined using Bland-Altman analysis to identify possible bias (mean divergence) and the limits of agreement (two standard deviations of the divergence). To assess reproducibility, the coefficient of variation was calculated as the standard deviation of the difference divided by the mean. Data were analyzed using Minitab version 16.0 (Minitab Inc., State College, PA).
Results
In the ANT group, 22 patients were evaluated. Three patients were dropped from the serial study (two died, one was lost to follow-up.) The remaining 19 patients (12 male, seven female) with a mean age at cancer diagnosis of 15.3 ± 3 years (range, 13–19 years) were evaluated. Cancer diagnoses in the ANT cohort included osteosarcoma ( n = 7), Ewing sarcoma ( n = 3), Hodgkin lymphoma ( n = 3), B-cell lymphoma ( n = 2), rhabdomyosarcoma ( n = 2), malignant peripheral nerve sheath tumor ( n = 1), and acute lymphoblastic leukemia ( n = 1). The mean cumulative ANT dose was 296 ± 103 mg/m 2 (range, 75–450 mg/m 2 ). ANT agents used were doxorubicin (89%), idarubicin (32%), and daunorubicin (5%). For ANT patients, echocardiographic examinations were performed at baseline, mid, and final treatment points (0, 4, and 8 months). The time after final ANT dose until the 8-month echocardiographic examination was a mean of 86 ± 52 days.
Echocardiographic Evaluation of Systolic and Diastolic Function
Table 1 highlights demographic and standard echocardiographic parameters of systolic and diastolic function of the ANT group and controls at baseline and the ANT group at 4 and 8 months. Both groups had similar age, gender, body surface area, indexed LV mass, and LV EF. Systolic blood pressure was significantly lower in the ANT group compared with controls, while heart rate was elevated in the ANT group compared with controls. Increased indexed LVESV measurements were not significantly different at 4 and 8 months compared with baseline (27 ± 5 mL/m 2 , P = .08, and 28 ± 6 mL/m 2 , P = .11). Significant differences between the ANT group and controls were identified for E/A ratio and mitral A velocity at the 4-month and 8-month time points and lateral and medial mitral annular early diastolic myocardial velocity and end-diastolic interventricular wall thickness at 8 months.
Variable | ANT group | Controls (baseline) ( n = 19) | ||
---|---|---|---|---|
0 months ( n = 19) | 4 months ( n = 19) | 8 months ( n = 19) | ||
Age (y) | 15.3 ± 3 | — | — | 15.3 ± 3 |
Male gender | 12 | — | — | 11 |
BSA (m 2 ) | 1.6 ± 0.2 | 1.4 ± 0.2 † | 1.4 ± 0.2 † | 1.7 ± 0.3 |
BMI (kg/m 2 ) | 20 ± 4 ∗ | 19 ± 3 ‡ | 19 ± 3 ∗ | 22 ± 2 |
Heart rate (beats/min) | 80 ± 14 ∗ | 90 ± 11 ∗ | 85 ± 16 ∗ | 62 ± 10 |
Systolic blood pressure (mm Hg) | 106 ± 10 ∗ | 104 ± 8.4 ∗ | 107 ± 9.2 † | 119 ± 12 |
LVEDD (mm) | 42 ± 4.6 | 43 ± 4.4 | 43 ± 3.3 | 48 ± 5 |
LVESD (mm) | 26 ± 3.3 | 27 ± 4.1 | 28 ± 2.2 | 30 ± 3.4 |
End-diastolic inferolateral WT (mm) | 7.8 ± 1.1 | 8.2 ± 1.4 | 7.8 ± 0.9 | 8.2 ± 1.1 |
End-diastolic IVS WT (mm) | 7.7 ± 0.8 | 7.8 ± 1.3 | 7.5 ± 0.8 ‡ | 8.5 ± 1.3 |
LV EF (%) | 62 ± 3 | 61 ± 5 | 59 ± 3 | 61 ± 5 |
Indexed LVESV (mL/m 2 ) | 25 ± 7 | 27 ± 5 | 28 ± 6 | — |
Indexed LV mass (g/m 2.7 ) | 30 ± 8 | 29 ± 6 | 30 ± 7 | 33 ± 7 |
Mitral E velocity (cm/sec) | 1.0 ± 0.2 | 0.9 ± 0.2 | 0.9 ± 0.2 | 1.0 ± 0.2 |
Mitral A velocity (cm/sec) | 0.5 ± 0.2 | 0.6 ± 0.2 † | 0.6 ± 0.2 ∗ | 0.7 ± 0.4 |
E/A ratio | 2.2 ± 0.9 | 1.8 ± 0.7 † | 1.5 ± 0.3 ∗ | 2.8 ± 0.7 |
E′ lateral (cm/sec) | 0.13 ± 0.02 | 0.12 ± 0.03 | 0.11 ± 0.03 † | 0.14 ± 0.02 |
E′ medial (cm/sec) | 0.18 ± 0.05 | 0.18 ± 0.05 | 0.16 ± 0.04 ‡ | 0.19 ± 0.03 |
S′ lateral (cm/sec) | 0.10 ± 0.02 | 0.09 ± 0.02 | 0.09 ± 0.02 | 0.09 ± 0.01 |
S′ medial (cm/sec) | 0.09 ± 0.01 | 0.08 ± 0.02 ‡ | 0.09 ± 0.02 | 0.10 ± 0.02 |
Speckle-Tracking Echocardiographic Data: ANT Group Versus Control Group
LV LPSS data for the ANT group and the control group are shown in Table 2 . LV global LPSS average was decreased in the ANT group compared with matched controls at 4 months (18.1 ± 2.5% vs 20.5 ± 1.5%, P = .011) and 8 months (18.1 ± 2.8%, P = .032). Significantly decreased regional LV LPSS values in the ANT group compared with controls were observed for basal average, basal anterolateral segment, mid anterior, mid inferior, and mid averages (4 and 8 months). Significantly decreased LPSS was also noted for basal posterolateral, basal anteroseptal (8 months); apical average, apical cap, apical inferior, apical anterior, apical septal, and mid inferoseptum (4 months).
LV LPSS | ANT group | Controls (baseline) ( n = 19) | ||
---|---|---|---|---|
0 months ( n = 19) | 4 months ( n = 19) | 8 months ( n = 19) | ||
Segment | ||||
Basal average | −20.8 ± 2.0 | −17.1 ± 2.4 ∗ | −17.4 ± 3.0 ∗ | −20.4 ± 2.0 |
Basal inferior | −20.4 ± 3.3 | −19.8 ± 4.5 | −18.8 ± 3.3 | −21.7 ± 3.5 |
Basal anterior | −22.8 ± 5.4 | −20.4 ± 4.2 | −18.7 ± 3.3 ‡ | −21.3 ± 3.7 |
Basal posterolateral | −20.5 ± 6.1 | −17.4 ± 4.8 | −16.3 ± 5.2 † | −20.3 ± 4.6 |
Basal anteroseptal | −20.9 ± 3.0 | −17.7 ± 4.4 | −16.9 ± 3.6 ‡ | −19.3 ± 3.2 |
Basal anterolateral | −19.9 ± 5.7 | −16.2 ± 5.4 ‡ | −15.9 ± 3.4 ∗ | −19.9 ± 3.3 |
Basal inferoseptum | −19.3 ± 3.3 | −18.0 ± 2.1 | −18.8 ± 3.3 | −19.2 ± 3.5 |
Mid average | −20.5 ± 2.3 | −18.1 ± 2.1 † | −18.0 ± 3.1 ‡ | −20.7 ± 1.8 |
Mid inferior | −18.0 ± 2.1 | −18.9 ± 3.3 ‡ | −19.9 ± 4.2 | −21.7 ± 2.8 |
Mid anterior | −18.7 ± 4.3 | −19.1 ± 2.9 ‡ | −15.5 ± 6.4 ∗ | −21.7 ± 2.6 |
Mid posterolateral | −20.2 ± 3.2 | −18.1 ± 4.3 | −17.3 ± 3.9 | −18.9 ± 3.4 |
Mid anteroseptal | −20.6 ± 2.9 | −19.7 ± 2.2 | −19.4 ± 5.2 | −21.2 ± 2.8 |
Mid anterolateral | −20.0 ± 3.3 | −17.8 ± 3.6 | −16.9 ± 3.8 | −18.8 ± 2.3 |
Mid inferoseptum | −20.6 ± 2.9 | −19.4 ± 1.9 ‡ | −19.6 ± 3.6 | −21.5 ± 3.5 |
Apical average | −18.5 ± 3.4 | −16.8 ± 3.4 † | −18.6 ± 4.6 | −20.9 ± 2.9 |
Apical cap | −17.7 ± 4.2 | −16.6 ± 4.1 † | −18.6 ± 4.6 | −21.1 ± 2.7 |
Apical inferior | −21.1 ± 4.2 | −18.5 ± 4.2 ‡ | −19.7 ± 4.8 | −21.4 ± 2.9 |
Apical lateral | −18.3 ± 3.8 | −18.1 ± 3.8 | −18.5 ± 5.2 | −20.2 ± 3.3 |
Apical anterior | −14.6 ± 5.4 | −13.8 ± 3.0 ∗ | −16.7 ± 5.9 | −20.1 ± 3.8 |
Apical septal | −18.5 ± 4.0 | −17.2 ± 3.3 ∗ | −19.4 ± 5.2 | −22.0 ± 3.8 |
Global average | −19.9 ± 2.1 | −18.1 ± 2.5 † | −18.1 ± 2.8 ‡ | −20.5 ± 1.5 |
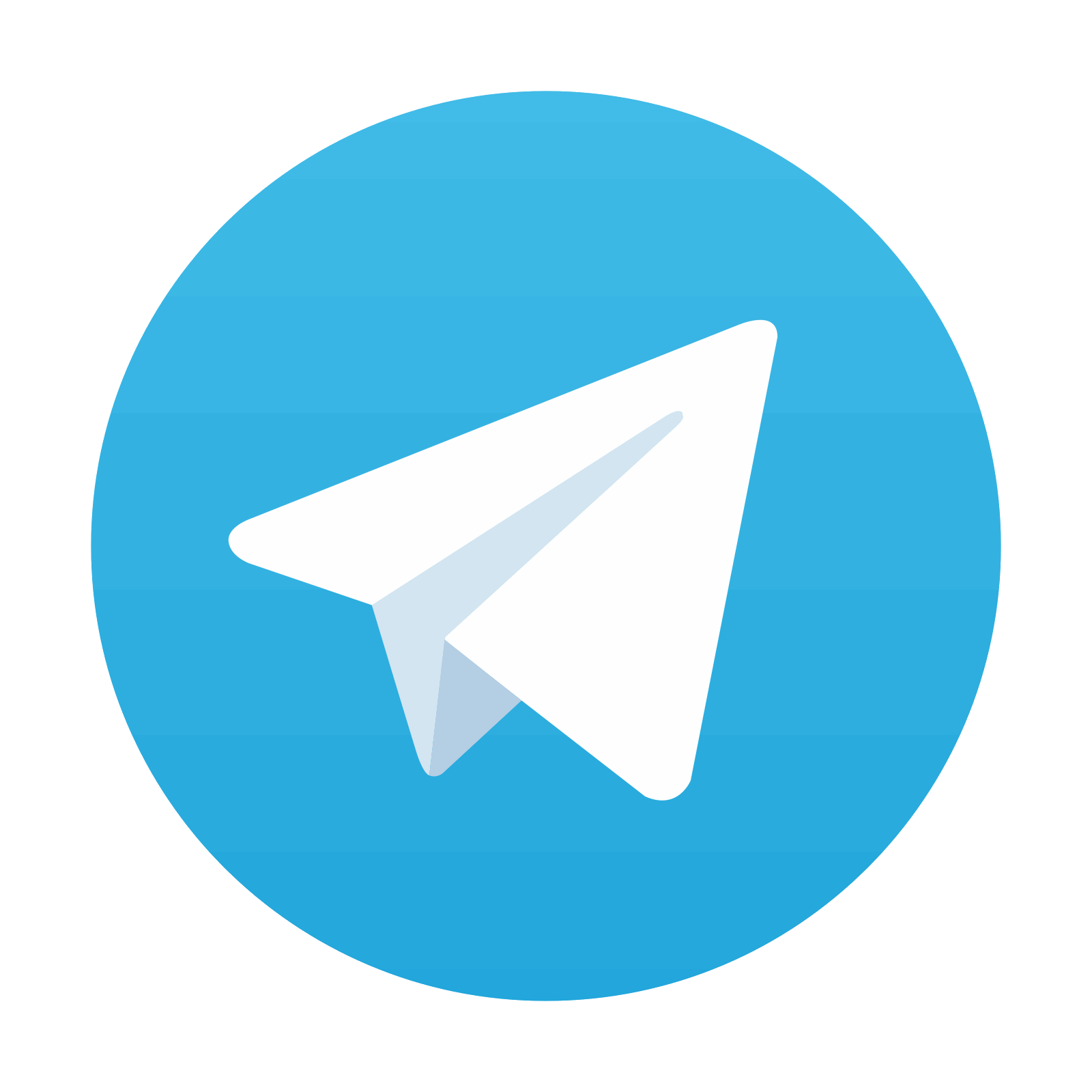
Stay updated, free articles. Join our Telegram channel

Full access? Get Clinical Tree
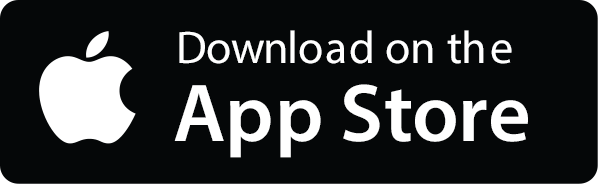
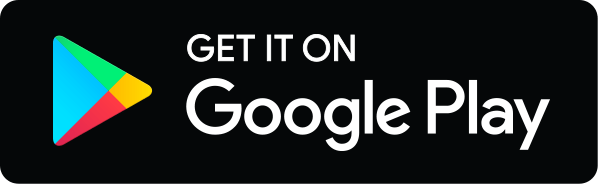
