Fig. 6.1
Mechanisms of action of MSCs. MSCs promote benefitial effects through cell-to-cell interactions and through secretion of soluble mediators, microvesicles and whole organelles, that can directly affect many cells, regulating, for example, the innate and adaptative immune system
The mechanisms by which MSCs might alleviate inflammation and injury are not completely understood and, as in other organ systems, likely involve multiple pathways including release of soluble mediators and/or microsomal particles as well as cell–cell contact. Importantly, the mechanisms of MSCs actions are different in different lung diseases and reflect the ability of the MSCs to sense and respond differently to different inflammatory environments (Weiss 2014; Weiss et al. 2013a, b). Much current interest in MSCs has focused on soluble factors due to their ability to secrete multiple paracrine factors such as growth factors, factors regulating endothelial and epithelial permeability, factors regulating innate and adaptive immunity, anti-inflammatory cytokines, and more recently, antimicrobial peptides. Some of the soluble mediators implicated in the different model systems include IL-6, IL-10, indoleamine 2,3-dioxygenase (IDO), Nitrous Oxide (NO), hepatocyte growth factor (HGF), and transforming growth factor (TGF)-β (Lotfinegad et al. 2014). Transduction or transfection of the MSCs to over-express secreted mediators including angiopoietin-1 or keratinocyte growth factor (KGF) further decreases endotoxin-mediated lung injury presumably through abrogation of endotoxin-mediated endothelial injury (Xu et al. 2008; Chen et al. 2013). While native MSCs are effective, MSCs transduced to over-express eNOS, IL-10, KGF, or a CCL2 inhibitor were found to be more effective in preventing monocrotaline-induced pulmonary hypertension, ischemia-reperfusion-induced lung injury, or bleomycin-induced pulmonary inflammation and subsequent fibrosis, respectively (Prockop and Oh 2012a, b; Keating 2012; Weiss 2013; Antunes et al. 2014; Kanki-Horimoto et al. 2006). MSCs appear also to act in part by decreasing the increased endothelial permeability found in acute lung injury, by secreting antibacterial peptides, by promoting an anti-inflammatory M2 phenotype in alveolar macrophages, by increasing monocyte phagocytic activity, and by reducing collagen fiber content associated with increased metalloproteinase-8 expression and decreased expression of tissue inhibitor of metalloproteinase-1 (Weiss et al. 2013a, b). However, MSCs may not always ameliorate lung injury with some pre-clinical data suggesting that MSCs may contribute to established lung fibrosis (Epperly et al. 2003; Yan et al. 2007; Weiss and Ortiz 2013).
In addition, the ability to secrete microparticles that contain not only proteins but RNA or miRNA species which can modulate the expression of multiple genes make these packaging vesicles an attractive and quite plausible means for MSCs to regulate multiple pathways and produce a robust therapeutic effect in different lung injury models (Lee et al. 2012; Aliotta et al. 2012; Zhang et al. 2012a, b; Thebaud and Stewart 2012; Islam et al. 2012). Besides this, direct mitochondrial transfer from MSCs to ATII cells through connexin 43-mediated cell–cell bridges has been demonstrated to replenish endotoxin-depleted ATP stores and restore surfactant secretion (Islam et al. 2012).
Importantly, MSCs can also exert effects on lung inflammation and injury through primary interactions with the immune system rather than through direct actions in lung. For example, available information demonstrates that MSCs alleviate endotoxin-induced acute lung injury in mouse models inhibiting Th1 response through release of soluble anti-inflammatory, anti-bacterial, and angiogenic substances, including IL-10, angiopoietin 1, KGF, and others (Mei et al. 2007; Lee et al. 2009a, b; Danchuk et al. 2011; Gupta et al. 2012; Ionescu et al. 2012a, b). In contrast, MSC administration in mouse models of asthma (allergic airways inflammation) ameliorates airways hyper-responsiveness by reducing Th2/Th17-mediated inflammation through effects on antigen-specific T lymphocytes and by up-regulating T-regulatory cells (Cho et al. 2009; Park et al. 2010; Nemeth et al. 2010; Firinci et al. 2011; Goodwin et al. 2011). As such, as MSC-based therapies are developed for lung diseases, the specific disease pathogenesis in the context of the known actions of the MSCs must be carefully considered (Fig. 6.2).
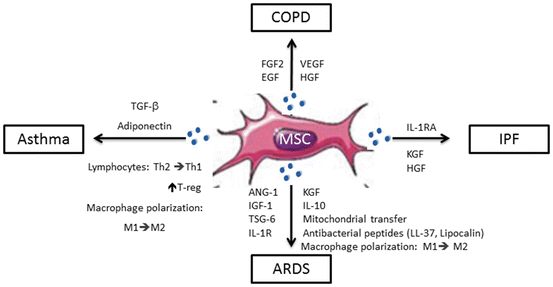
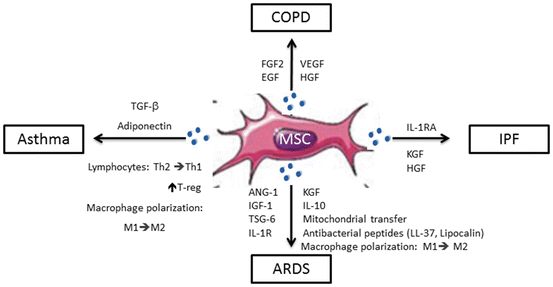
Fig. 6.2
MSCs trigger different responses according to each lung pathological environment. MSCs can secrete different soluble mediators depending on the lung disease microenvironment they get exposed to
6.4 Localization of MSCs in Lung After Systemic MSC Administration
Following systemic administration of MSCs isolated from bone marrow, adipose, placenta, or cord blood, a number of studies demonstrate that the cells initially localize in the lung vascular bed and that lung injury results in increased localization and/or retention of marrow-derived cells in lung (reviewed by Weiss 2013; Antunes et al. 2014). Whether this represents formation of cell emboli in the lung vasculature or specific adherence to pulmonary vascular adhesion or other molecules remains unclear. Further, the source of the MSCs may influence retention in the lung. For example, MSCs derived from human umbilical cord blood are cleared more rapidly from the lungs than are human bone marrow-derived MSCs (Nystedt et al. 2013). This reflects both differences in size of the MSCs from different sources as well as differential expression of specific integrin and proteoglycan patterns. Retention in the lung may also trigger the MSCs to have functional effects. For example, embolization of systemically administered MSCs in lung was felt to result in secretion of an anti-inflammatory protein, TSG-6 (Lee et al. 2009a, b). However, although bone marrow- or adipose-derived MSCs can be induced in vitro to express phenotypic markers of alveolar or airway epithelial cells, retention of MSCs in the lung is generally transient. Structural engraftment of MSCs as lung epithelium is a rare event of uncertain physiologic significance in lung (Loi et al. 2006; Sueblinvong et al. 2008; Ma et al. 2011; Maria and Tran 2011; Li et al. 2012; Yan et al. 2012; Baer 2011). However, some available data suggests that systemically administered MSCs can engraft as fibroblasts or myofibroblasts under certain fibrosing injury conditions, further discussed below (Antunes et al. 2014; Kanki-Horimoto et al. 2006). This is a potential undesirable effect of the MSCs.
6.5 Use of MSCs in Lung Diseases
A steadily increasing number of articles demonstrate efficacy of either systemic or intratracheal administration of MSCs obtained from bone marrow, adipose, cord blood, or placenta in a growing spectrum of lung injury models in mice and in a slowly growing number of clinical investigations in lung diseases (reviewed by Weiss 2013; Antunes et al. 2014). This includes mouse models of acute lung injury and bacterial lung infection (Gupta et al. 2012; Ionescu et al. 2012a, b; Danchuk et al. 2011; Kim et al. 2011; Sun et al. 2011a, b; Xu et al. 2012; Zhang et al. 2013), asthma (Firinci et al. 2011; Goodwin et al. 2011; Kapoor et al. 2011; Kavanagh and Mahon 2011; Lee et al. 2011; Ou-Yang et al. 2011; Ionescu et al. 2012a, b; Lathrop et al. 2014), bronchopulmonary dysplasia (Chang et al. 2011; Pierro et al. 2013; Zhang et al. 2010, 2012a, b; Tropea et al. 2012; Sutsko et al. 2013), COPD (Hoffman et al. 2011; Katsha et al. 2011; Schweitzer et al. 2011; Ingenito et al. 2012; Kim et al. 2012), ischemia re-perfusion injury (Yang et al. 2009; Manning et al. 2010; Sun et al. 2011a, b), post-inflammatory lung fibrosis (Ortiz et al. 2003, 2007; Rojas et al. 2005; Zhao et al. 2008; Aguilar et al. 2009; Kumamoto et al. 2009; Moodley et al. 2009; Cargnoni et al. 2010; Cabral et al. 2011; Lee et al. 2010; Saito et al. 2011), pulmonary hypertension (Lee et al. 2012; Baber et al. 2007; Umar et al. 2009; Kanki-Horimoto et al. 2006; Hansmann et al. 2012; Liang et al. 2011), sepsis and burns (Gonzalez-Rey et al. 2009; Nemeth et al. 2009; Iyer et al. 2010; Mei et al. 2010; Yagi et al. 2010a, b; Krasnodembskaya et al. 2012), and other critical illness or autoimmune-related lung injuries including hemorrhagic shock, lupus, pancreatitis, silicosis, and ventilator-induced lung injury (Shi et al. 2012; Pati et al. 2011; Wang et al. 2012; Lassance et al. 2009; Chimenti et al. 2012; Curley et al. 2012). Systemically administered MSCs can also home to tumors, through as yet unclear chemotactic mechanisms, and have been utilized for delivery of chemotherapeutic and other anti-tumor agents in mouse lung tumor models. This may provide a viable therapy for lung cancers, particularly with MSCs engineered to express anti-tumor compounds such as tumor necrosis factor-related apoptosis-inducing ligand (TRAIL) or Interferon beta (IFN-β) (Kanehira et al. 2007; Rachakatla et al. 2007; Stoff-Khalili et al. 2007; Xin et al. 2007; Zhang et al. 2008; Matsuzuka et al. 2010; Loebinger et al. 2009a, b; Heo et al. 2011; Hu et al. 2012). MSC administration has also been demonstrated to alleviate inflammation and injury produced by intratracheal instillation of either endotoxin or bacterial in human lung explants (Lee et al. 2009a, b, 2013).
In parallel with robust pre-clinical data, a slowly growing number of clinical investigations of MSC-based therapy in different lung diseases including ARDS, COPD, IPF, and silicosis are occurring (Table 6.1). In the following sections, the rationale for potential MSC effects, available pre-clinical data, and considerations of clinical trials of MSCs in ARDS, COPD, IPF, and silicosis will be considered.
Table 6.1
Worldwide stem cell clinical trials on lung diseases. Brief description of study location, number of enrolled patients, source and dose of MSCs, frequency of injections, route of administration, follow-up length, study status and clinical trials registration number in clinicaltrials.gov
Disease | Location | Patients | Cell type | Dose | Frequency | Delivery | Follow-up | Status | ClinicalTrials.gov |
---|---|---|---|---|---|---|---|---|---|
ARDS | USA | 69 | BM-MSC | 1–10 × 106/kg | Single dose | Intravenous | 12 months | Recruiting | NCT01775774 |
USA | 60 | BM-MSC | 1 × 107/kg | Single dose | Intravenous | 12 months | Recruiting | NCT02097641 | |
China | 12 | AD-MSC | 1 × 106/kg | Single dose | Intravenous | 28 days | Recruiting | NCT01902082 | |
China | 20 | MEN-MSC | 1 × 107/kg | Twice weekly for 2 weeks | Intravenous | 14 days | Recruiting | NCT02095444 | |
Sweden | 10 | BM-MSC | a | a | a | 12 months | Recruiting | NCT02215811 | |
COPD | Brazil | 4 | BMDMC | 1 × 108/ml | Single dose | Intravenous | 12 months | Completed | NCT01110252 |
USA | 62 | BM-MSC | 1 × 108 | Four monthly | Intravenous | 2 years | Completed | NCT00683722 | |
Brazil | 10 | BM-MSC | a | Single dose | Endobronchial | 4 months | Recruiting | NCT01872624 | |
Netherlands | 10 | BM-MSC | a | Twice weekly | Intravenous | 8 weeks | Completed | NCT01306513 | |
Russia | 30 | BM-MSC | 2 × 108 | Every 2 months for 1 year | Intravenous | 2 years | Recruiting | NCT01849159 | |
Iran | 12 | BM-MSC | 6 × 107 | Single dose | Endobronchial | 1 year | Not recruiting | NCT01758055 | |
Mexico | 30 | AD-MSC | a | Single dose | Intravenous | 6 months | Recruiting | NCT01559051 | |
IPF | USA | 25 | BM-MSC | 2 × 107 | Single dose | Intravenous | 60 weeks | Recruiting | NCT02013700 |
Spain | 18 | BM-MSC | Escalating doses | a | Endobronchial | 12 months | Recruiting | NCT01919827 | |
Australia | 8 | PL-MSC | 1–2 × 106/kg | Single dose | Intravenous | 6 months | Not recruiting | NCT01385644 | |
Silicosis | Brazil | 5 | BM-MSC | 2 × 107/kg | Single dose | Endobronchial | 6 months | Recruiting | NCT01239862 |
6.6 Acute Respiratory Distress Syndrome
The immunomodulatory and reparative potential of MSCs makes them potential therapeutic tools for the acute inflammatory response to infection and pulmonary injury seen in ARDS. Several pre-clinical studies on ARDS have demonstrated that MSCs may improve the pulmonary and systemic inflammation characteristic of the disease (Rojas et al. 2005; Nemeth et al. 2009; Mei et al. 2010; Gupta et al. 2007). In models of endotoxin- or bacterial-induced ARDS mice and in explanted human lungs, MSC administration not only attenuates inflammation by decreasing several inflammatory mediators, including tumor necrosis factor-alpha (TNF-α), macrophage inflammatory protein 2-alpha (MIP-2), IFN-γ, IL-1β, MIP-1α, IL-6, IL-8, and keratinocyte-derived cytokine in plasma and bronchoalveolar lavage fluid, but it is also able to rescue epithelial cells with mitochondrial dysfunction by mitochondria transfer (Islam et al. 2012; Spees et al. 2006). In addition, MSCs favorably influence the host response to bacterial infections, the commonest and most severe cause of ARDS. MSC therapy can reduce bacterial counts via a number of mechanisms, including increased antimicrobial peptide secretion, such as lipocalin-2 (Gupta et al. 2012), and enhanced macrophage phagocytosis (Krasnodembskaya et al. 2012; Nemeth et al. 2009). MSCs also enhance repair following lung injury, as evidenced by the findings that both intravenous (Curley et al. 2012) and intratracheal (Curley et al. 2013) MSC therapy restore lung function following ventilator-induced lung injury via a KGF-dependent mechanism. Based on these promising preclinical findings, a number of early-phase clinical trials have begun to investigate the potential of MSC therapy for severe ARDS.
Currently, five studies of MSC therapy safety in patients with ARDS are listed in ClinicalTrials.gov. At the University of California, San Francisco, a phase I, multicenter, open-label dose escalation clinical trial is in progress to assess the safety of intravenous infusion of allogeneic bone marrow-derived human MSCs in ARDS (NCT01775774) and a phase II, multicenter study was initiated in March, 2014, to assess the safety and efficacy of a single dose of allogeneic bone marrow-derived human MSCs infusion in patients with ARDS. In Sweden, a phase I, multi-center, open-label, non-randomized controlled trial is also testing the safety of bone-marrow-derived MSCs in ARDS (NCT02215811). Two phase I, randomized, double-blind, placebo-controlled trials are also taking place in China to test the safety of systemic infusion of allogeneic human adipose MSCs (NCT01902082) and of MSCs derived from menstrual blood (NCT02095444) in ARDS patients.
6.7 Chronic Obstructive Pulmonary Disease
In several preclinical studies, MSC administration has been demonstrated to attenuate inflammation by decreasing levels of inflammatory mediators, such as IL-1β, TNF-α, IL-8, as well as decrease apoptosis (Huh et al. 2011; Zhen et al. 2010), improve parenchymal repair (increased levels of KGF, HGF, and epidermal growth factor), and increase lung perfusion (Huh et al. 2011; Shigemura et al. 2006; Guan et al. 2013). Based on these preclinical findings, several groups are investigating the therapeutic potential of MSC therapy in COPD patients.
The first safety trial registered in ClinicalTrials.gov (NCT01110252) assessed systemic administration of autologous bone marrow mononuclear cells in four Brazilian patients/volunteers with advanced COPD (stage IV dyspnea) and found no obvious adverse effects after 1 year (Ribeiro-paes et al. 2011). In a recent trial carried out in the United States (NCT00683722), using non-HLA matched allogeneic bone marrow-derived MSCs obtained from healthy volunteers (Prochymal®; Osiris Therapeutics Inc), sixty-two patients were randomized to double-blinded intravenous infusions of either allogeneic MSCs or vehicle control. Patients received four monthly infusions (100 × 106 cells/infusion) and were subsequently followed for 2 years after the first infusion (Weiss et al. 2013a, b). This trial demonstrated that use of MSCs in COPD patients may be considered safe, as there were no infusion reactions and no deaths or serious adverse events deemed related to MSC administration. However, no significant differences were observed in the overall number of adverse events, frequency of COPD exacerbations, or severity of disease in patients treated with MSCs. A significant decrease was observed in circulating C-reactive protein in MSC-treated patients giving a potential mechanistic clue of MSC actions.
A phase I, non-randomized, open-label study in Brazil is currently recruiting patients diagnosed with severe heterogeneous emphysema to evaluate the safety of one-way endobronchial valves combined with bone-marrow MSCs (NCT01872624). Another phase I, non-randomized, non-blinded, prospective study to test the safety and feasibility of administration of bone-marrow MSCs before and after lung volume reduction surgery for severe pulmonary COPD has been concluded in the Netherlands (NCT01306513). Results for this study are pending. An open-label, non-randomized, multicenter study is currently underway in Mexico to evaluate the safety and efficacy of autologous adipose-derived stem cell transplantation in GOLD moderate-severe patients (NCT01559051).
6.8 Idiopathic Pulmonary Fibrosis
When administered early after injury is instituted, MSCs attenuate inflammation and prevent development of bleomycin-induced lung fibrosis in mice, the most commonly utilized experimental model. However, administration of MSCs at time intervals longer than 7 days after bleomycin administration had no effect on established fibrotic changes in either mouse or pig lungs (Ortiz et al. 2003). Further, using a different model of lung fibrosis induced by radiation exposure in rodents, MSCs administered at time points at which established fibrotic changes were present, MSCs were detected in the interstitium as myofibroblasts suggesting that fibroblastic differentiation of MSC occurred in response to mediators produced in the injured tissue (Epperly et al. 2003; Yan et al. 2007). These data suggest that MSC administration in the setting of an established or ongoing fibrotic response may worsen the disease process and augment scarring in injured tissue rather than reversing it. As such, available data only supports a potential ameliorating effect of MSC administration in fibrotic lung diseases if administered early in the disease course during active inflammation (Weiss et al. 2013a, b). At present, there is no data to support an ameliorating effect of MSCs on established lung fibrosis. Thus, careful consideration must be given to clinical investigations of MSCs in fibrotic lung diseases.
Despite these concerns, there are three trials listed in ClinicalTrials.gov that are taking place to evaluate the safety and feasibility of MSC therapy in IPF patients. In the United States, a phase I/ll, randomized, blinded, and placebo-controlled trial is recruiting 25 IPF patients to investigate the safety, tolerability, and potential efficacy of intravenous infusion of allogeneic human MSCs (NCT02013700). Another phase I, open-label, multicenter, non-randomized study will evaluate the safety and feasibility of the endobronchial infusion of autologous bone-marrow MSCs at escalating doses in patients with mild-to-moderate IPF at Navarra University in Spain (NCT01919827). A third phase I, open-label, single-center, non-randomized dose-escalation study in Australia evaluated the safety and feasibility of placental-derived MSC infusion in IPF patients (NCT01385644). Initial results from this trial demonstrate no adverse effects over the 6-month follow-up period. A fourth trial, not listed in clinicaltrials.gov, reported no adverse effects of endobronchial administration of autologous adipose-derived MSCs over a 1-year follow-up period (Tzouvelekis et al. 2013).
6.9 Silicosis
Preclinical studies using an experimental model of silicosis demonstrated that both systemic and intratracheal administration of autologous BMMCs reduce inflammation and fibrosis (Lassance et al. 2009; Lopes-Pacheco et al. 2013). These positive effects encouraged a non-randomized, phase I trial of endobronchial administration of autologous BMMCs in patients with chronic and accelerated silicosis in Brazil (NCT01239862). In this study, three patients each received 2 × 107 bone marrow-derived cells labeled with 99mTc. The cellular infusion procedure was well tolerated by the patients, and no respiratory, cardiovascular, or hematological complications were observed. Scintigraphy showed an increase in lung perfusion in the basal region up to day 180 after the infusion, while the apex and midzone areas presented reduced perfusion at day 180 (Loivos et al. 2010; Souza et al. 2012). However, no subsequent clinical study of MSCs in silicosis has occurred.
6.10 Conclusions and Future Directions
Cell therapy approaches for lung diseases and critical illnesses including ARDS, COPD, IPF, and silicosis continue to evolve at a rapid pace. Pre-clinical studies with MSCs have generated a great amount of enthusiasm as a beneficial therapy for lung diseases and critical illnesses. Initial clinical trials have demonstrated that MSC administration is safe, with few adverse effects, but substantial challenges still have to be overcome before MSCs can be used for clinical practice. As such, further studies focusing on understanding the mechanisms of action of MSCs must be more investigated in order to continue to develop rational approaches for clinical trials. Nonetheless, cell-based therapies with MSCs and other cell types offer potential hope for these devastating and incurable pulmonary diseases.
References
Aguilar S, Scotton CJ, McNulty K et al (2009) Bone marrow stem cells expressing keratinocyte growth factor via an inducible lentivirus protects against bleomycin-induced pulmonary fibrosis. PLoS One 4:e8013PubMedCentralPubMed
Aliotta JM, Lee D, Puente N, Faradyan S, Sears E, Amaral A, Goldberg L, Dooner MS, Pereira M, Quesenberry PJ (2012) Progenitor/stem cell fate determination: interactive dynamics of cell cycle and microvesicles. Stem Cells Dev 21(10):1627–1638PubMedCentralPubMed
Antunes MA, Laffey JG, Pelosi P, Rocco PR (2014) Mesenchymal stem cell trials for pulmonary diseases. J Cell Biochem. doi:10.1002/jcb.24783 PubMed
Baber SR, Deng W, Master RG, Bunnell BA, Taylor BK, Murthy SN, Hyman AL, Kadowitz PJ (2007) Intratracheal mesenchymal stem cell administration attenuates monocrotaline-induced pulmonary hypertension and endothelial dysfunction. Am J Physiol Heart Circ Physiol 292:H1120–H1128PubMed
Baer PC (2011) Adipose-derived stem cells and their potential to differentiate into the epithelial lineage. Stem Cells Dev 20(10):1805–1816PubMed
Baer PC, Geiger H (2012) Adipose-derived mesenchymal stromal/stem cells: tissue localization, characterization, and heterogeneity. Stem Cells Int 2012:812693PubMedCentralPubMed
Bi Y, Ehirchiou D, Kilts TM, Inkson CA, Embree MC, Sonoyama W et al (2007) Identification of tendon stem/progenitor cells and the role of the extracellular matrix in their niche. Nat Med 13(10):1219–1227PubMed
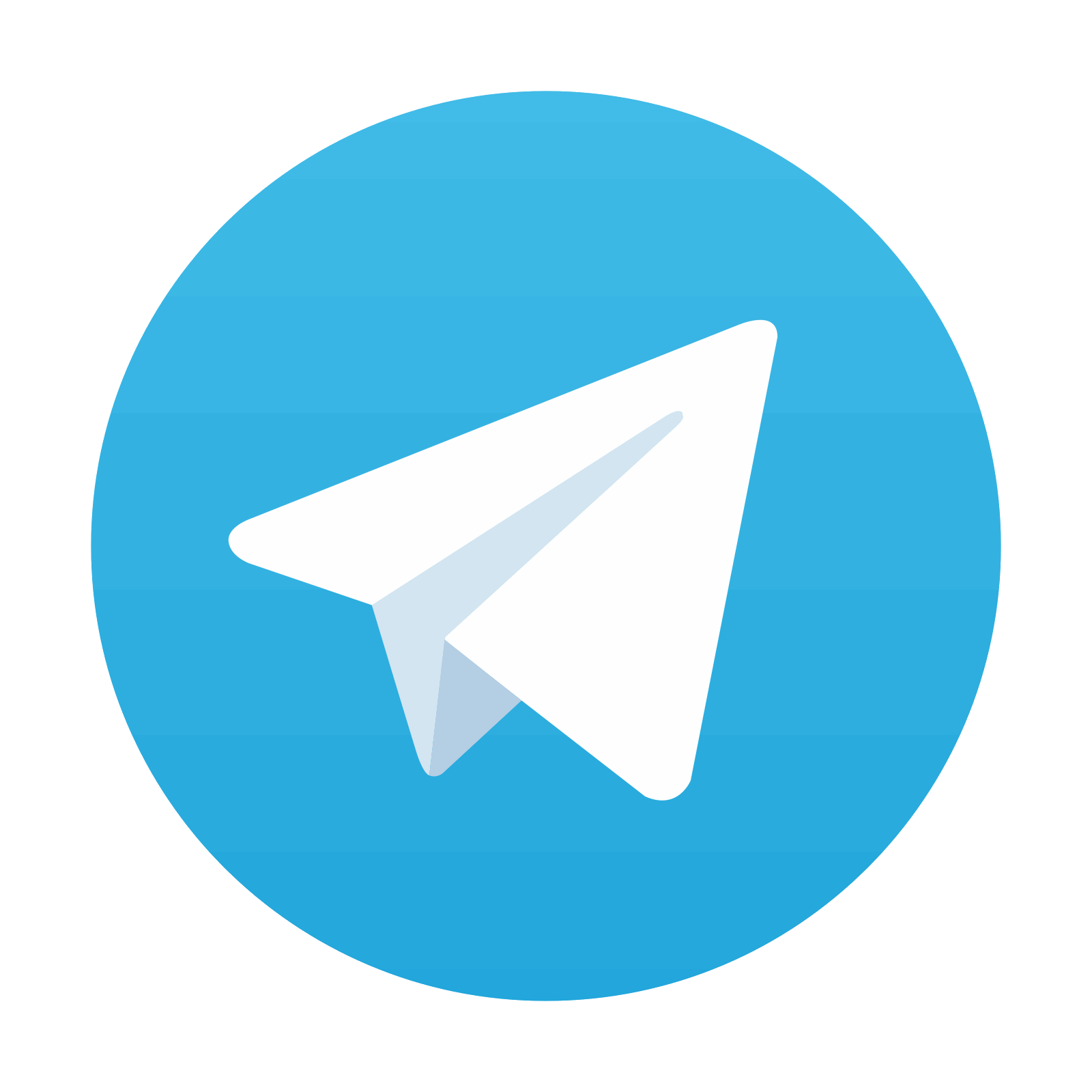
Stay updated, free articles. Join our Telegram channel

Full access? Get Clinical Tree
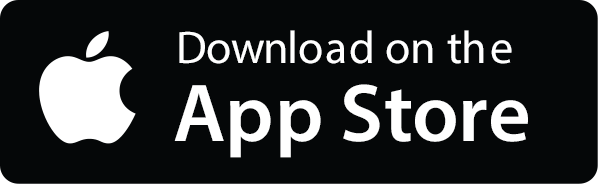
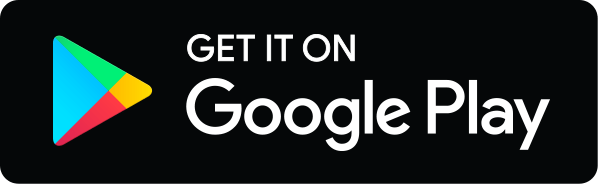