Background
The generation of velocity-time integrals (VTIs) from Doppler signals is an essential component of standard echocardiographic investigations. The most effective algorithm to compensate for growth in children has, however, not yet been identified. This study was initiated to establish pediatric reference values for VTI and to enhance the interpretability of those values, considering technical and physiological factors.
Methods
The echocardiographic data sets of healthy children and adolescents ( N = 349; age range, 0–20 years) were recorded in a prospective approach and subsequently analyzed. In a pilot study, aortic and pulmonary VTIs were set in relation to the physiologic parameters of heart size as possible influencing parameters in a subgroup of children with comparable physical characteristics. The ratio with the smallest SD was taken as the base to generate centile curves using the LMS method. The clinical utility of the model was tested by examining patients ( n = 80) with shunt lesions such as patent ductus arteriosus and atrial septal defect.
Results
Feasibility was 94.6% for aortic VTI and 92.8% for pulmonary VTI. The pilot study identified ventricular length and heart rate as suitable parameters with the lowest relative SDs and high correlations with VTI. Gender differences were not relevant for children <7 years of age, and with increasing age, SD increased because of higher stroke volume variations. The detection of increased aortic VTI was possible with sensitivity of 73% for patients with patent ductus arteriosus with moderate or large hemodynamically significant ductus arteriosus. Patients with atrial septal defects with enlarged right ventricles could be identified as having increased pulmonary VTI with sensitivity of 84%.
Conclusions
These new reference values for VTI times heart rate as a function of ventricular length may be of specific clinical value to improve the assessment of cardiac function, therapeutic decision making, and follow-up in pediatric patients with heart disease.
Highlights
- •
This publication provides new reference values for pediatric aortic and pulmonary velocity time integral.
- •
The evaluation of VTI related to heart rate and ventricular length increases the informative value of this echocardiographic parameter.
- •
The echocardiographic evaluation of patients with volume overload can be improved by combining the parameters heart rate, ventricular length, and VTI compared to single VTI measurement.
- •
Follow up of pediatric patients with congenital heart disease might be facilitated.
The quantification of cardiac function is essential for therapeutic decision making in patients with heart disease. Especially in patients with congenital heart disease, who often have abnormal cardiac anatomy, data on the noninvasive measurement of cardiac output (CO) are unsatisfactory.
Cardiac magnetic resonance can involve phase contrast, which is currently regarded as the gold standard to measure flow and CO, but it is not commonly available; moreover, it is rather expensive and time intensive, and it requires sedation or even general anesthesia in young children. In addition, serial or daily measurements to assess therapeutic success during treatment are not practicable using magnetic resonance. For longitudinal monitoring of cardiac function, echocardiography remains the method of choice ; it can be applied easily to all patients at the bedside, but conventionally used two-dimensional (2D) methods lack precision in matters of stroke volume (SV) determination. This becomes apparent when using either biplanar quantification of areas or methods including the integration of Doppler waveforms and the determination of cross-sectional areas, because these measurements rely strongly on calculations based on formulas and anatomic assumptions that do not reflect real cardiac anatomy or flow conditions.
The measurement of Doppler waveforms across great vessels is carried out by every cardiologist for the estimation of velocities and gradients; this technique is highly reproducible. Furthermore, it has been shown that measurements of the velocity-time integral (VTI) improve CO estimation as it is “a linear analogue” of SV, or at least proportional to SV. Nonetheless, interpretation of the area under the Doppler waveform (VTI) is often challenging, because it can be influenced by numerous factors, such as heart rate (HR), the configuration of outflow tracts, and chamber size. This aspect becomes even more complicated if children exhibit differences in cardiac size and HR due to growth. This may imply that high ranges of VTI are defined as “normal” despite a potential clinical pathology, which may limit the clinical utility of these reference values.
It was the aim of this study to delineate measures that compensate for these systematic difficulties and to establish reference values for VTI in children that are clinically valuable to distinguish between normal and abnormal SV and CO, without calculating an accurate value for SV or CO.
Methods
Study Description
Using a prospective approach, children and adolescents were investigated with 2D echocardiography during the period from April 2007 to June 2013. Written informed consent was provided by parents or legal guardians, and the study was approved by the institutional ethics committee (64/2014).
Heart disease was ruled out, and only subjects in sinus rhythm were included. Age, height, and weight were recorded; body surface area (BSA) was calculated using the formula published by Du Bois and Du Bois. A Vivid E9, Vivid 7 (GE Medical Systems, Milwaukee, WI), or iE33 (Philips Medical Systems, Best, the Netherlands) ultrasonic device equipped with standard probes was used. The investigation was performed with the patient in the left supine position to collect standard views according to the guidelines of pediatric echocardiography.
Sedation was not needed. For Doppler tracings, only pulsed-wave Doppler was used, with the sample volume positioned just distal to the tips of the valve to be investigated. Aortic VTI was taken from an apical five-chamber view; for pulmonary VTI, a short-axis view was used to provide accurate angulations to get the best signal possible and reduce spectral dispersion.
Echocardiographic data sets were recorded digitally, and measurements were performed on a personal computer with EchoPAC version 6.1.2 (GE Medical Systems) or Xcelera release 4.1L1 – 4.1.1.1133 ( Figures 1–3 ).



Figure 1 illustrates left ventricular (LV) and right ventricular (RV) length measurement: the basal border of the left ventricle was defined as the line connecting the mitral valve annular hinge points; LV length was measured from the basal border midpoint to the apical endocardium at end-diastole, defined as the frame with the maximal chamber intraluminal area. The basal border of the right ventricle was defined as the line connecting the tricuspid valve annular hinge points; RV length was measured from the basal border midpoint to the RV apex at end-diastole, defined as the frame at which the tricuspid valve closes. The mean VTI was determined by planimetry of three sequential waveforms, as shown in Figures 2 and 3 .
Reference Value Study
On the basis of the results of the pilot study (for more information, see the Appendix , available at www.onlinejase.com ), the most suitable parameter on which VTI could be based was defined. Appropriate measurements were carried out in 349 children and adolescents. Reference values were created as percentile curves (Pc) using the LMS method of Cole (LMS version 1.27; Institute of Child Health, London, United Kingdom), which is based on shaping three curves, L, M, and S (L for the box-Cox power, λ; M for the mean, μ; and S for the coefficient of variation, σ), that summarize the measurement’s distribution over the range of the covariate and contain the information needed to draw any centile curve.
Statistical Analysis
Descriptive statistics were assessed using SPSS version 21 (SPSS, Chicago, IL). Normality of distribution was tested using the Kolmogorov-Smirnov test. The Mann-Whitney U test or Student’s t test was used to test the significance of the observed differences ( P values ≤ .05 were considered to indicate statistical significance). Intra- and interobserver variability were tested as in several previous studies but were nevertheless reevaluated. Echocardiographic data sets of 20 children selected by chance were evaluated twice by the same investigator and additionally by a second investigator, each blinded to the results of the first evaluation. Bland-Altman analysis was used to determine intra- and interobserver variability.
Test of Clinical Utility
To evaluate the clinical utility of the centile curves, patients with known shunt lesions leading to aortic or pulmonary hyperperfusion (group A, patent ductus arteriosus [PDA]; group B, atrial septal defect [ASD]) were examined by the same investigator who performed measurements in healthy children before interventional closure of the PDA or ASD. To categorize the severity of the shunt and following volume overload or chamber enlargement, standard echocardiographic measurements as recommended in current guidelines were carried out. An invasive Qp/Qs measurement was not part of the intervention protocol.
The shunt size of the PDA was assessed quantitatively by measuring the left atrium–to–aortic annulus ratio (LA/Ao) and LV end-diastolic diameter on M-mode imaging.
In patients with LA/Ao < 1.5:1, the shunt was considered small or insignificant. LA/Ao between 1.5:1 and 2.0:1 was considered to reflect a moderate shunt size, while LA/Ao > 2:1 was considered to represent large shunts.
In patients with ASDs, RV end-diastolic diameters at the basal and midcavity levels were measured in apical four-chamber views for the quantification of RV dilatation, and values were compared with normative data. Additionally, the maximum ASD diameter was measured by transesophageal echocardiography during the intervention.
Results
Feasibility
In total, 349 data sets were analyzed. Data sets were not excluded if measurements for only one ventricle were missing; the inclusion of data set was carried out separately for parameters related to the right and left ventricles. Feasibility was 94.6% for aortic VTI and LV length measurements ( n = 330) and 92.8% for pulmonary VTI and RV length measurements ( n = 324).
Reference Values
Tables 1 and 2 show the demographic and echocardiographic data of healthy children for the final generation of reference values. The decision to evaluate VTI multiplied by HR (minute distance [MD]) related to ventricular length was taken on the basis of the pilot study (see the Appendix for more information, available at www.onlinejase.com ). Application of the LMS method of Cole resulted in the centile curves presented in Figures 4 and 5 .
Variable | All ( N = 330) | Aged 0–6 y ( n = 114) | Aged 7–20 y, female ( n = 103) | Aged 7–20 y, male ( n = 113) |
---|---|---|---|---|
Age (y) | 0.0–20.0 (9.3 ± 5.4) | 0.0–6.9 (3.2 ± 2.5) | 7.1–18.8 (12.1 ± 3.1) | 7.3–20.0 (13.0 ± 3.5) |
Height (cm) | 42–210 (133 ± 39) | 42–148 (91 ± 29) | 110–181 (151 ± 16) | 119–210 (160 ± 20) |
Weight (kg) | 1.9–120.0 (36.5 ± 22.4) | 1.9–37.0 (13.7 ± 8.0) | 20.0–80.0 (44.4 ± 14.2) | 21.0–120.0 (52.4 ± 19.8) |
BSA (m 2 ) | 0.14–2.65 (1.15 ± 0.52) | 0.14–1.25 (0.58 ± 0.26) | 0.78–1.89 (1.36 ± 0.28) | 0.84–2.65 (1.52 ± 0.37) |
HR (beats/min) | 47–168 (89 ± 23) | 49–168 (110 ± 24) | 54–106 (80 ± 12) | 47–116 (76 ± 13) |
LV length (mm) | 24–108 (68 ± 18) | 24–78 (49 ± 13) | 56–96 (76 ± 9) | 61–108 (80 ± 11) |
Aortic VTI (cm) | 4.9–34.2 (21.2 ± 5.4) | 4.9–30.4 (16.6 ± 5.0) | 14.8–34.2 (23.5 ± 3.9) | 13.5–34.1 (23.7 ± 3.7) |
Variable | All ( N = 324) | Aged 0–6 y ( n = 112) | Aged 7–20 y, female ( n = 99) | Aged 7–20 y, male ( n = 113) |
---|---|---|---|---|
Age (y) | 0.0–19.8 (9.3 ± 5.4) | 0.0–6.9 (3.1 ± 2.5) | 7.1–18.8 (12.2 ± 3.0) | 7.3–19.8 (12.9 ± 3.4) |
Height (cm) | 42–210 (113 ± 39) | 42–148 (90 ± 29) | 110–181 (152 ± 16) | 119–210 (159 ± 19) |
Weight (kg) | 1.9–120.0 (36.2 ± 22.0) | 1.9–37.0 (13.5 ± 7.9) | 20.0–80.0 (44.9 ± 14.1) | 21.0–120.0 (51.2 ± 18.9) |
BSA (m 2 ) | 0.14–2.65 (1.14 ± 0.52) | 0.14–1.25 (0.57 ± 0.27) | 0.78–1.89 (1.38 ± 0.28) | 0.84–2.65 (1.50 ± 0.36) |
HR (beats/min) | 42–168 (89 ± 23) | 65–168 (110 ± 23) | 52–121 (79 ± 12) | 35–112 (76 ± 13) |
RV length (mm) | 24–108 (63 ± 17) | 24–73 (45 ± 12) | 53–93 (70 ± 9) | 56–108 (74 ± 10) |
Pulmonary VTI (cm) | 7.8–27.7 (19.1 ± 3.4) | 7.8–26.4 (16.5 ± 3.1) | 13.0–27.7 (20.4 ± 2.7) | 14.1–27.3 (20.5 ± 2.7) |


Statistics
Differences between female and male subjects were statistically significant only for children ≥7 years of age. Consequently, further analysis was performed in three groups (0–6 years, unisex; 7–20 years, female; 7–20 years, male). Intraobserver variability for echocardiographic measurements was low, with mean differences between 1.2% and 5.1%; interobserver variability was slightly higher, with mean differences between 2.6% and 10.1%. The complete results of the Bland-Altman analysis for intra- and interobserver variability and the correlation coefficients are presented in Table 3 .
Variable | Intraobserver variability | Interobserver variability | ||||
---|---|---|---|---|---|---|
Mean ± SD (%) | LOA (%) | ICC | Mean ± SD (%) | LOA (%) | ICC | |
LV length (mm) | 5.1 ± 4.9 | −4.7 to 14.9 | 0.952 | 4.8 ± 6.0 | −7.3 to 16.8 | 0.967 |
Aortic VTI (cm) | −1.7 ± 3.2 | −8.1 to 4.6 | 0.988 | 2.6 ± 3.9 | −5.2 to 10.4 | 0.985 |
RV length (mm) | 1.2 ± 5.2 | −9.2 to 11.6 | 0.969 | 10.1 ± 6.2 | −2.3 to 22.6 | 0.952 |
Pulmonary VTI (cm) | 2.9 ± 4.5 | −11.9 to 6.0 | 0.918 | 5.1 ± 5.3 | −5.5 to 15.6 | 0.936 |
Test of Clinical Utility
Table 4 shows the demographic data and echocardiographic values measured in patients with PDA and ASDs. Figures 6 and 7 show the values of children with congenital heart disease plotted into centile curves. The severity of cardiac disease, classified by the comparison of values measured in patients and normal values in the literature, is represented by color codes. Values of patients with PDA with LA/Ao < 1.5:1 are all located in the reference range under the 97th percentile. Values of children with suspected moderate hemodynamic significant ductus arteriosus (LA/Ao 1.5:1–2:1) are mostly in the upper reference range or above the 97th percentile. In contrast, values of children with an allusion to large hemodynamic significant ductus arteriosus and large shunt volume are nearly all outside the reference range ( Figure 6 ). Values from patients with ASDs with normal RV midcavity diameters ( Z score < 2) are predominantly located in the reference range, whereas values from children with enlarged right ventricles (RV midcavity end-diastolic diameter Z score > 2) are mainly above the 97th percentile ( Figure 7 ).
Variable | PDA | ASD |
---|---|---|
( n = 40) | ( n = 40) | |
Age (y) | 0–6 (1.88 ± 1.89) | 0–6 (4.3 ± 1.50) |
Height (cm) | 45–121 (77 ± 21.95) | 75–126 (106 ± 12.02) |
Weight (kg) | 2.2–21.3 (9.6 ± 5.62) | 8.4–31.0 (17.5 ± 4.41) |
BSA (m 2 ) | 0.16–0.85 (0.44 ± 0. 20) | 0.41–1.00 (0.71 ± 0.13) |
HR (beats/min) | 82–177 (118 ± 18.80) | 83–131 (103 ± 12.33) |
Ventricular length (mm) | 30–64 (44 ± 8.37) | 40–65 (51 ± 5.94) |
Aortic VTI (cm) | 12.8–36.1 (22.9 ± 5.20) | |
LVEDD (mm) | 18–43 (30 ± 0.66) | |
LA (mm) | 11–30 (20 ± 0.49) | |
LA/Ao | 1.3–3.0 (1.9 ± 0.34) | |
Pulmonary VTI (cm) | 16.8–35.5 (26.6 ± 4.60) | |
RV basal (mm) | 26–45 (34 ± 3.99) | |
RV mid (mm) | 24–40 (30 ± 3.80) | |
Maximal ASD dimension (mm) | 8–24 (14 ± 3.68) |
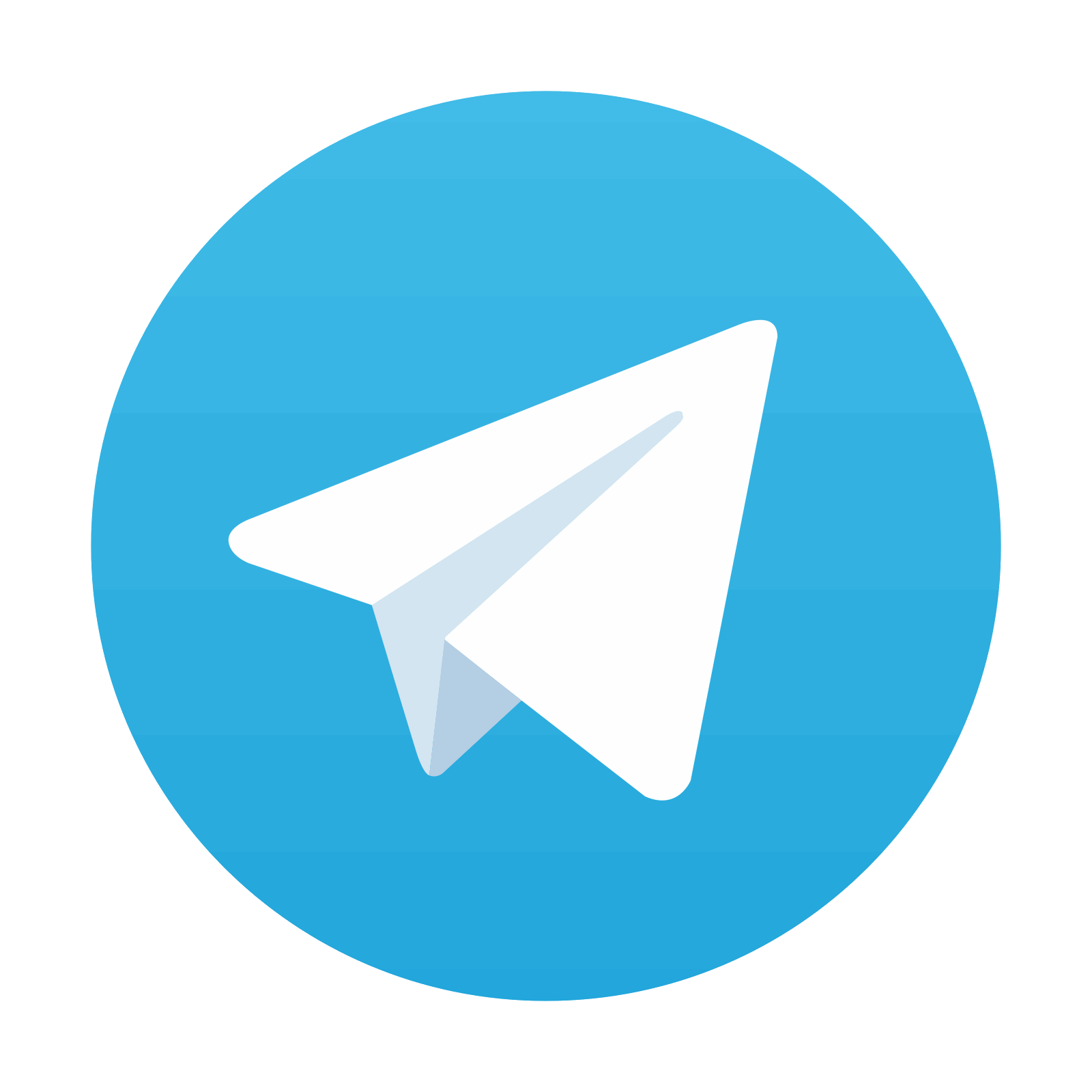
Stay updated, free articles. Join our Telegram channel

Full access? Get Clinical Tree
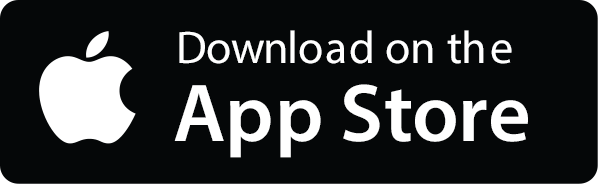
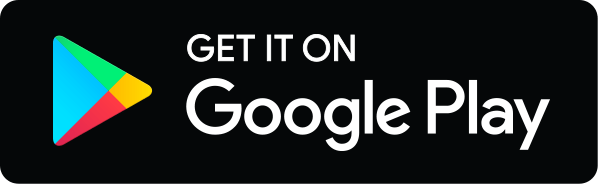
