Fig. 30.1
The pathway to clinical translation. Vital steps along the path involve small-animal studies (proof-of-concept studies and studies to investigate the mechanism of action) and large-animal studies (to optimize dosage, formulation, and delivery as well as to test safety and efficacy in clinically relevant models), before moving on to human subjects. Skeletal myoblasts (SKMs) followed a fairly convincing preclinical developmental program (without systematic testing in large animals) before moving on to humans; they are currently being tested in phase 2 trials. On the other hand, bone marrow mononuclear cells (BM-MNCs) entered the clinical arena immediately after the first reports of rodent studies; currently large-scale phase 3 outcome trials are about to commence. In the case of bone marrow mesenchymal stromal cells (BM-MSCs) and heart-derived cells, a more logical and systematic approach for clinical translation has been employed; BM-MSCs are now entering phase 3 trials while heart-derived cells are currently being tested in phase 2 trials. Embryonic stem cells (ESCs) and induced pluripotent stem cells (iPSCs) are currently being tested in large animals. The arrows represent the progression of the discussed cell types along the path to clinical translation
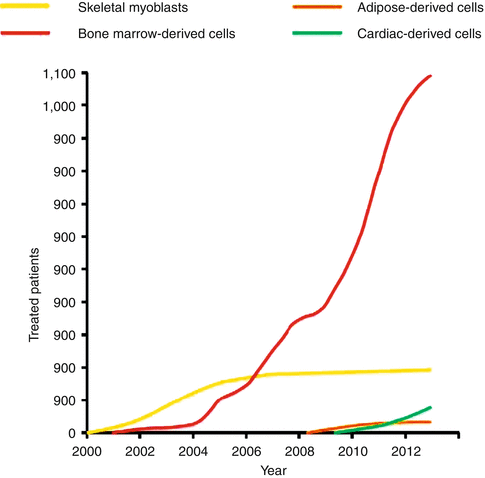
Fig. 30.2
Cell therapy in patients with cardiac disease. The approximate number of patients with cardiac disease enrolled in clinical studies and treated with various cell types from 2000 (the first clinical application of cell therapy for heart disease) to 2013 is presented
Within this framework, we now consider and critically assess each of the major cell types that has been tested (or is planned to be tested in the case of embryonic stem cells/induced pluripotent stem cells) in patients.
30.3 Skeletal Myoblasts
Skeletal myoblasts (SKMs), mononucleated cells located between the basal lamina and the sarcolemma of skeletal myofibers, are activated, proliferate, differentiate and fuse to form new skeletal muscle fibers (myotubes) in response to injury. They are resistant to ischemia, and can be easily harvested in abundant numbers and expanded for autologous transplantation. These features, along with their myogenic phenotype, have made SKMs an attractive population for cardiac repair and regeneration.
Preclinical experimental studies suggested that administration of autologous SKMs improves LV function and attenuates remodeling in animal models of ischemic heart disease [6, 7]. In recipient myocardium, SKMs differentiate into multinucleated myotubes (not cardiomyocytes) that lack expression of connexin-43 and gap junctions, forming islands of conduction block that slow conduction velocity and create the substrate for reentrant arrhythmias [8].
After a decade of experimental studies [6, 7] SKMs were the first cell type to enter the clinical arena for heart regeneration. In June 2000 autologous SKMs, isolated and expanded from a thigh muscle biopsy, were intramyocardially injected in a patient with severe ischemic heart failure as an adjunct to coronary bypass grafting (CABG) surgery [9]. Several small non-randomized phase I studies ensued demonstrating functional benefit in treated patients, albeit with a high incidence of ventricular arrhythmias, confirming the concerns raised by preclinical research [10, 11]. The first phase II SKM trial, MAGIC, enrolled patients with chronic ischemic cardiomyopathy randomized to receive direct intramyocardial injections (as an adjunct to CABG surgery) of autologous myoblasts or placebo solution. The MAGIC trial failed to demonstrate efficacy and was discontinued prematurely, while a trend towards more ventricular arrhythmias in the treated groups was observed, despite the prophylactic use of amiodarone [12]. Increased incidence of ventricular arrhythmias in treated patients was also observed in the pilot MARVEL-1 study, a randomized, placebo-controlled trial of catheter-guided SKM transplantation in patients with ischemic heart failure that was also discontinued prematurely (due to financial reasons) [13]. On the other hand, the SEISMIC trial demonstrated a more favorable safety profile of catheter-guided SKM administration in patients with ischemic heart failure, along with a trend towards increased exercise tolerance in treated patients; nevertheless, no significant effect on LV ejection fraction (EF) was observed [14]. Taken together, the trajectory of SKMs is instructive and argues against premature enthusiasm solely on the basis of preclinical studies.
30.4 Bone Marrow-Derived Cells
The bone marrow is a highly heterogeneous tissue, containing several different cell populations with progenitor-like properties. These progenitor cells can be roughly divided into the following three categories: (a) hematopoietic stem cells (HSCs) that can give rise to all types of mature blood cells; (b) endothelial progenitor cells (EPCs), a subset of hematopoietic cells that promote neovascularization either directly (differentiation into endothelial cells) or indirectly (secretion of pro-angiogenic cytokines); and (c) mesenchymal stromal cells (MSCs) that can differentiate into osteoblasts, chondroblasts and adipocytes.
In the clinical field, bone-marrow derived cells have been administered in the following forms: (a) autologous bone marrow mononuclear cells (BMMNCs) either unfractionated or after purification for a progenitor cell marker (CD34 or CD133); and (b) autologous or allogeneic MSCs. These cellular products are considered individually below.
30.4.1 Bone Marrow Mononuclear Cells
Unlike SKMs, BMMNCs moved into patients without the benefit of a convincing preclinical development program; the first report of clinical application of BMMNCs for heart regeneration [15] surfaced within 4 months of the publication of a rodent study reporting extensive engraftment and cardiogenic differentiation of bone marrow-derived cells in mice [16]. Clinical application was catalyzed by the relative accessibility of bone marrow, the large numbers of unfractionated autologous cells that can be obtained without ex vivo expansion, and the extensive clinical experience with bone marrow transplantation. Ironically, the initial report of extensive transdifferentiation of bone marrow-derived cells into cardiomyocytes has proven to be controversial; several laboratories have been unable to reproduce the findings [17, 18]. Nevertheless, clinical studies have continued apace. It should be noted that BMMNCs, isolated by density centrifugation of bone marrow aspirates, actually contain very few progenitor cells (~2–4 % HSCs/EPCs and ~0.01 % MSCs); the vast majority of BMMNCs comprise committed hematopoietic cells at various stages of maturation [19].
In the clinical setting, autologous BMMNCs are by far the most frequently administered cell type for treatment of heart disease and have been used primarily in the setting of acute MI. To date >1,000 patients have been treated with BMMNCs in numerous clinical trials worldwide. As with SKMs, early small and uncontrolled pilot studies of BMMNC delivery after acute MI demonstrated consistently positive results [20, 21]. However, the results of later large randomized studies of intracoronary infusion of BMMNCs in acute MI have been conflicting. The first large-scale randomized but not placebo-controlled study (BOOST) demonstrated a transient functional benefit (increase in EF) at 6 months after BMMNC infusion [22] that was not sustained at 18 months or 5 years after therapy [23]. Two subsequent placebo-controlled studies yielded varied results: REPAIR-AMI showed a significant improvement in EF at 4 months [24], whereas a study by Jannsens et al. [25] (in which BMMNCs were infused within 24 h after reperfusion, earlier than in the other studies) showed no improvement in EF at 4 months but a more profound decrease in scar size in cell-treated patients. In contrast to the previous studies, results of the randomized but not placebo-controlled ASTAMI trial were resoundingly negative [26]. The results of subsequent large randomized studies have also been conflicting, ranging from: (a) positive: the FINCELL trial [27], the first study of BMMNC infusion in patients treated with thrombolysis, showed improvement of EF at 6 months; (b) marginally positive: in the REGENT study [28], EF improved in the cell-treated patients but not in controls at 6 months; however, no difference was observed when the treatment effect was compared among groups; (c) mixed: in the BONAMI trial no difference in EF but an increase in myocardial viability was observed after BMMNC therapy [29]; or (d) resoundingly negative: in the HEBE trial [30], SCAMI trial [31], TIME trial [32], LateTIME trial [33], and SWISS-AMI trial [34] no benefits of BMMNC infusion in patients with acute MI were observed. The reasons for the inconsistent outcomes of the aforementioned clinical trials are unclear but may be attributed to differences in cell isolation and preparation protocols [35]. Nevertheless, a recent meta-analysis (including 811 patients participating in 13 randomized trials) showed modest but significant benefit following BMMNC therapy: EF increased by ~3 %, end-systolic volume decreased by ~5 ml and scar size decreased by ~3.5 % in the cell-treated groups compared with controls [36].
Critically reviewing the accumulated data of BMMNC therapy in the acute MI setting, a number of conclusions can be drawn:
(i)
(ii)
Sustained functional benefits after BMMNC therapy in acute MI remain in doubt;
(iii)
The patient population enrolled in BMMNC trials was not very ill at baseline (first MI population receiving prompt reperfusion, with a median EF of ~50 % pre-therapy) leaving little room for improvement.
The legacy of the studies of BMMNC therapy in acute MI has left the field puzzled. The next (and possibly definitive) chapter for BMMNCs will be the BAMI study (NCT01569178), a large phase 3 trial to be conducted in Europe with all-cause mortality as the primary endpoint. 3,000 patients with acute MI and EF <45 % will be randomized to either conventional therapy or intracoronary infusion of autologous BMMNCs. The BAMI study is powered to detect a 25 % relative decrease in 2-year all-cause mortality after cell therapy (11.5 % vs. 8.6 %). This highly anticipated study will hopefully answer, once and for all, whether BMMNCs are a useful adjunctive therapy in acute MI.
BMMNCs have also been used for the treatment of refractory angina. To date, four randomized controlled studies have been published. The study by van Ramhorst et al. showed that catheter-guided injection of BMMNCs resulted in improvements in myocardial perfusion as assessed by single photon emission computed tomography and EF at 3 months after therapy [37]; such improvements were not observed, however, in the PROTECT-CAD trial and the study by Losordo et al. (catheter-guided injection of BMMNCs and granulocyte colony stimulating factor [GCSF]–mobilized CD34+ cells, respectively) [38, 39]. A subsequent placebo-controlled phase 2 trial by the Losordo group, in which patients with refractory angina were randomized to receive catheter-guided transendocardial injections of GCSF-mobilized autologous CD34+ cells (collected from the peripheral blood via leukapheresis) or placebo, revealed significant improvements in symptoms (reduced angina frequency) and functional capacity (increased exercise tolerance) in cell-treated patients. However, cell-mobilization and collection procedures were associated with cardiac enzyme elevations in 4.6 % of patients [40]. CD34+ cells for the treatment of refractory angina are currently entering phase 3 trials (RENEW study, NCT01508910). In RENEW (which is currently recruiting participants) 444 patients with refractory angina will be randomized in a 2:1:1 fashion to cell therapy (transendocardial injection of GCSF-mobilized autologous CD34+ cells collected from the peripheral blood via leukapheresis), active control (GCSF-mediated stem cell mobilization, apheresis, and intramyocardial placebo injection), or open-label standard of care. The primary efficacy endpoint of RENEW is change in exercise treadmill time (the study has 90 % power to detect a 60-s difference in exercise time between cell-treated and active control patients) [41].
Finally, BMMNCs have also been investigated in the setting of chronic ischemic cardiomyopathy and heart failure, albeit on a much smaller scale compared with acute MI (or to a lesser extent refractory angina). Early, small clinical studies have shown some hints of efficacy [42–44]. However, the FOCUS trial (a multicenter phase II, randomized, placebo-controlled trial investigating the efficacy of transendocardial injection of BMMNCs in patients with chronic ischemic cardiomyopathy and heart failure) was resoundingly negative and failed to meet any of its pre-specified efficacy endpoints (no change in end-systolic left ventricular volumes, maximal oxygen consumption or myocardial perfusion) [45].
With regard to mechanism of action, well designed studies employing genetic labeling techniques have convincingly demonstrated that bone marrow-derived cells cannot readily transdifferentiate into cardiomyocytes. Thus, the prevailing concept of bone marrow-derived cell bioactivity has shifted towards the ‘paracrine hypothesis’, according to which the transplanted cells are proposed to produce soluble factors that are beneficial to the infarcted heart. With regard to the latter, studies in rodents have shown that exogenously administered bone marrow-derived cells can indirectly (in a paracrine manner) protect resident cardiomyocytes from apoptosis, stimulate angiogenesis and upregulate recruitment of endogenous cardiac progenitors in the infarcted heart [46].
30.4.2 Bone Marrow Mesenchymal Stromal Cells
MSCs reside in the bone marrow and their physiologic role is to create/function as a stromal network supporting the differentiation of HSCs into blood lineages. MSCs are self-renewing cells characterized by: (1) adherence to plastic in culture; (2) surface expression of CD105, CD90, and CD73; (3) lack of expression of hematopoietic markers; and (4) the capacity to differentiate into osteoblasts, adipocytes, and chondroblasts under specific in vitro conditions. While the ability of MSCs to undergo true cardiomyogenic transdifferentiation remains highly controversial [47, 48], MSCs are known to secrete a broad variety of cytokines, chemokines and growth factors involved in cardiac repair. To that end, preclinical animal studies have shown that MSCs can indirectly (in a paracrine manner) protect resident cardiomyocytes from apoptosis [49], promote angiogenesis [50], stimulate proliferation of endogenous cardiac progenitors and amplify resident cardiomyocyte cell-cycle re-entry in the infarcted heart [51]. These indirect mechanisms seem to underlie the reduction in infarct size, attenuation of LV remodeling and improvement in cardiac function observed in large animal models of MI and chronic ischemic cardiomyopathy [52, 53].
In the clinical field, MSCs have been primarily used in the setting of chronic ischemic cardiomyopathy. The Hare group performed a head-to-head comparison of autologous bone marrow-derived MSCs versus autologous BMMNCs (delivered by catheter-guided transendocardial injections) in patients with chronic ischemic cardiomyopathy (randomized-controlled TAC-HFT study) [54]. While both MSCs and BMMNCs did not raise significant safety concerns and improved quality of life, only MSCs (but not BMMNCs) decreased scar size, improved regional contractility and increased 6-min walk distance. However, chamber volumes and EF did not improve with either cell population.
The benefit of autologous MSC therapy may be enhanced by exposing cells in vitro to a “cardiogenic cocktail”, a combination of cytokines that promotes adoption of a cardiopoietic phenotype (as indicated by expression of cardiac transcription factors in bone marrow-derived MSCs) [55]. In a rodent study by the Terzic group, infarcted animals treated with “cardiopoietic” bone marrow-derived MSCs derived greater functional benefit compared to animals treated with naïve MSCs [55]. The first clinical trial of “cardiopoietic” bone marrow-derived MSCs (delivered by catheter-guided transendocardial injections) for the treatment of ischemic heart failure, the randomized controlled C-CURE trial, demonstrated the safety and feasibility of this approach. C-CURE also revealed intriguing hints of efficacy: treatment with “cardiopoietic” MSCs improved systolic function, reduced LV end-systolic volume and improved 6-min walk distance compared to standard of care [56]. Following this initial study, a larger phase 3 trial powered to evaluate efficacy in ischemic heart failure patients has been approved and is currently recruiting participants (the CHART-1 study, with a composite primary endpoint comprising mortality, worsening of heart failure, quality of life, exercise capacity, cardiac volumes and EF [NCT01768702]).
Bone marrow-derived MSCs lack major histocompatibility complex class II receptors and costimulatory molecules, and possess immunomodulatory/immunosuppressive properties; these characteristics have made them attractive for allogeneic transplantation (without immunosuppression). A randomized, placebo-controlled trial of intravenous infusion of proprietary allogeneic bone marrow-derived MSCs (Prochymal, Osiris) in patients with acute MI did not raise any significant safety concerns and revealed hints of efficacy (improved EF) [57]. The latter is somewhat paradoxical, taking into account that preclinical studies have demonstrated that intravenous infusion is hampered by trapping of cells in the lungs, resulting in acute cardiac retention rates of <1 % [58]. With regard to chronic ischemic cardiomyopathy, the Hare group recently performed a head-to-head comparison between autologous and allogeneic bone marrow-derived MSCs, delivered by catheter-guided transendocardial injections. The major finding of POSEIDON is that therapy with allogeneic MSCs (without immunosuppression) appears to be safe and at least as effective as therapy with autologous MSCs (both allogeneic and autologous MSCs reduced infarct size and LV sphericity index but did not increase EF) [59]. The results of POSEIDON are encouraging, particularly with regard to the safety of allogeneic MSCs, but the small sample size and the lack of a proper control group (receiving standard of care without cell therapy) should prompt some caution regarding claims of efficacy [35]. With regard to heart failure, catheter-guided transendocardial injection of allogeneic proprietary stro-3+ bone marrow-derived MSCs (Revascor, Mesoblast) in 60 patients with ischemic and non-ischemic heart failure was not associated with a clinically significant immune response and resulted in a significant reduction of major adverse cardiac event rate. A phase III study of catheter-guided transendocardial injection of allogeneic stro-3+ bone marrow-derived MSCs is currently in preparation and plans to enroll 1,700 heart failure patients (Mesoblast). The primary efficacy endpoint of the trial is a time-to-first event analysis of heart failure-related major adverse cardiac events (defined as a composite of cardiac related death and decompensated heart failure events).
Bone marrow aside, subcutaneous adipose tissue is an alternative source of MSCs. Like bone marrow-derived MSCs, adipose-derived MSCs produce cytokines that promote angiogenesis, improve cardiac function and attenuate adverse remodeling in animal models of acute MI [60]. Importantly, autologous adipose-derived MSCs can be easily harvested in abundant numbers from lipoaspirates (with no need for ex vivo expansion, in contrast to bone marrow-derived MSCs); this enables therapeutic administration of an autologous cell product early in the course of an acute MI. In the clinical field, the randomized, placebo-controlled APOLLO trial demonstrated the feasibility and safety of intracoronary infusion of autologous adipose-derived MSCs in the setting of acute MI [61]. In addition, cell infusion in the APOLLO trial resulted in significant reductions in myocardial perfusion defect and scar size; however, the very small size of the control group (n = 4) precludes any efficacy-related conclusions. The efficacy of intracoronary infusion of autologous adipose-derived MSCs in the setting of acute MI is currently being investigated in the phase 2 ADVANCE trial (NCT01216995). In addition, ongoing clinical studies are assessing a potential role of adipose-derived MSCs in ischemic heart failure and refractory angina.
30.5 Heart-Derived Progenitor Cells
During the past decade several studies supported the notion that the adult mammalian heart contains its own reservoir of progenitor cells. Numerous populations of putative adult endogenous cardiac progenitor cells (CPCs) have been proposed, largely based on expression of surface markers or functional properties that have been used to mark progenitors in other organs. Putative adult CPCs include: c-kit+ (CD117+, the receptor for stem cell antigen) cells [62], cardiosphere-forming cells (CSps, three-dimensional structures of CPCs and supportive cells) [63], cardiosphere-derived cells (CDCs) [64], sca-1+ cells (stem cell antigen-1+ cells) [65], cardiac side population cells [66], and SSEA-1+ (stage-specific embryonic antigen-1+) cells [67]. The high number of distinct populations of putative endogenous CPCs is difficult to reconcile with the limited regenerative capacity of the adult mammalian heart. Importantly, while all of the aforementioned cell types have been shown to express cardiac proteins in vitro or after delivery into recipient hearts following ex vivo expansion, their physiologic importance and contribution to cardiomyocyte replenishment in the normal or injured adult heart remains a topic of intense debate. Nevertheless, most studies suggest that endogenous CPCs (the identity of which still remains elusive) may generate new myocytes in the adult heart. While the role of endogenous CPCs in postnatal cardiomyogenesis during normal ageing appears to be limited (as most studies concur that myocyte turnover in the healthy aging heart most likely occurs through proliferation of pre-existing myocytes [68, 69]), multiple studies indicate CPC-mediated cardiomyocyte renewal occurs following myocardial injury [68–70]. From a therapeutic perspective, since CPCs may presumably be endogenously programmed to replenish heart muscle, their isolation and ex vivo expansion for the treatment of heart diseases seems a logical (yet challenging) strategy. So far, only c-kit+ cells and CDCs have proceeded into human studies; these cellular products are considered individually below.
30.5.1 C-kit+ Cells
C-kit+ cells are self-renewing, clonogenic and can differentiate in vitro and in vivo into endothelial cells, smooth muscle cells and (possibly) cardiomyocytes [62]. Delivery of c-kit+ cells in small and large animal models of MI and ischemic cardiomyopathy results in improved systolic performance and attenuation of LV remodeling purportedly through generation of newly-formed cardiomyocytes and vessels by transplanted cells [62, 71, 72]. However, the ability of c-kit+ cells to readily differentiate into cardiomyocytes has been disputed by several studies reporting that adult c-kit+ cells have only angiogenic but not cardiomyogenic potential [73]. With regard to clinical translation, intracoronary infusion of autologous c-kit+ cells (isolated from right atrial appendages discarded during CABG surgery) in surgically revascularized patients with chronic ischemic heart failure is currently being investigated in the phase 1 SCIPIO trial. An interim analysis of the still-ongoing SCIPIO trial revealed that administration of c-kit+ cells resulted in striking improvement in EF (measured by echo), that was not observed in control patients [74]. MRI (which was only performed in a subset of treated patients but not in controls) revealed a significant reduction in scar mass and a significant increase in viable myocardium after cell therapy [75]. While SCIPIO demonstrated that isolation and intracoronary infusion of c-kit cells is feasible and does not raise significant safety concerns, claims of efficacy are undermined by methodological concerns (poor randomization scheme, lack of MRI in control patients, and confounding effect of CABG surgery which can by itself result in gradual natural improvement of heart function) [76].
30.5.2 Cardiosphere Derived Cells
CDCs are a naturally heterogeneous cell population of intrinsic cardiac origin. CDCs are uniformly positive for CD105, while a subset of CDCs (~25 %) is positive for CD90 (a marker of MSCs) and a small fraction (~3–10 %) is positive for c-kit (a putative marker of endogenous CPCs [as explained above]) [64]. CDCs can be readily grown in clinically relevant numbers from percutaneous endomyocardial biopsy specimens [64], and exhibit clonogenicity, self-renewal capacity and multipotentiality [77]. Several preclinical animal studies have demonstrated that CDCs can improve cardiac function, decrease scar size and increase viable myocardium post-MI in mice [64, 78], rats [79–81] and pigs [82, 83]. Importantly, the salutary effects of CDC transplantation cannot be explained by direct cardiomyogenic differentiation of transplanted cells (such events represent “needle in haystack” incidents [78, 81]) and should be attributed instead to indirect (paracrine) mechanisms of action. To that end, CDCs are potent secretors of cytokines that indirectly stimulate endogenous repair and regeneration [78, 81, 84], by promoting angiogenesis, recruitment of resident cardiac progenitor cells, and proliferation of preexisting cardiomyocytes in the infarct border zone [69, 78, 81]. This indirect mechanism of action (without the need for long-term engraftment of transplanted cells) rationalizes the efficacy of allogeneic CDCs; it has been shown that allogeneic CDCs are just as effective as syngeneic CDCs in a rat model of MI and in a pig model of ischemic cardiomyopathy [81, 83, 85]. With regard to clinical translation, a phase 1/2 clinical study of autologous CDCs—the CADUCEUS study, has been recently completed. In CADUCEUS, human subjects with convalescent MI (1.5–3 months post-MI) LV dysfunction (EF: 25–45 %) were randomized to receive intracoronary infusion of autologous CDCs (harvested from endomyocardial biopsies), or conventional treatment, by prospective randomized assignment. Intracoronary infusion of autologous CDCs did not raise significant safety concerns in CADUCEUS. Analysis of exploratory efficacy endpoints revealed a decrease in scar size, an increase in viable myocardium and improved regional function of infarcted myocardium (but not global function) in CDC-treated patients compared to controls [86, 87]. These intriguing hints of efficacy (which are consistent with therapeutic regeneration) need to be confirmed in future larger clinical trials. The safety and efficacy of allogeneic CDCs in human subjects with LV dysfunction post-MI is currently being tested in the phase 1/2 multicenter, randomized, placebo-controlled ALLSTAR trial (NCT01458405).
30.6 Embryonic Stem Cells and Induced Pluripotent Stem Cells
Embryonic stem cells (ESCs), derived from the inner mass of the developing embryo in the blastocyst stage, are the prototypical stem cells. They have the capacity of self-renewal, can be clonally expanded and are capable of differentiating into any cell type in the body, including atrial, ventricular and nodal cardiomyocytes. However, significant obstacles need to be overcome before ESCs can be used clinically for heart regeneration. First, the unlimited differentiation potential of ESCs is a double-edged sword: when ESCs are transplanted in their primitive undifferentiated state, they form teratomas in recipient hearts [88]. The risk of oncogenicity greatly diminishes after cardiomyogenic differentiation of ESCs in vitro prior to administration; the feasibility and safety of this approach has so far been demonstrated in small animal MI models [89]. Second, ESC-derived cardiac grafts could plausibly contribute to arrhythmogenesis through automaticity and triggered activity, while their irregular graft geometry/architecture (abnormal alignment of ESC-derived cardiomyocytes with host myocardium, smaller size of ESC-derived cardiomyocytes, abnormal distribution of connexins) could promote reentry [90]. Third, due to their allogeneic origin, ESCs carry the risk of immune rejection; there is now clear evidence that the differentiated progeny of ESCs are rejected by the host immune system [88]. Since ESCs regenerate the heart directly (through cardiomyogenic differentiation of exogenously administered cells), sustained benefits would presumably require permanent engraftment of transplanted ESCs, rendering the use of long-term immunosuppression in the clinical setting mandatory. Finally, ESCs are ethically problematic since they are created from early human embryos (discarded after in vitro fertilization).
In the past 6 years, induced pluripotent stem cells (iPSCs) from somatic (adult) cells have been generated through ectopic expression of selected transcription factors via viruses, plasmids, or bioengineered proteins [91]. The resultant iPSCs closely resemble ESCs and can be subsequently guided to differentiate into desired specific cell types (including cardiomyocytes) using specific protocols developed for ESC differentiation. These techniques make patient-specific (autologous) pluripotent stem cells an imaginable reality and provide an alternative source for cardiogenic cell lines; functional cardiomyocytes have been successfully derived from both mouse [92] and human iPSCs [93]. Nevertheless, significant roadblocks preclude near-term clinical applicability for heart regeneration, including the risk of oncogenicity associated with the pluripotent state, risk of arrhythmiogenicity, insertional mutagenesis due to integrating vectors, low efficiency of successful nuclear reprogramming and of subsequent cardiogenic differentiation, genetic abnormalities, potential immunogenicity despite the autologous origin, and high cost. Methods to expedite the generation of cardiomyocytes from noncontractile somatic cells, without transit through a pluripotent state, are intriguing [94]. Nevertheless, the use of genetically modified cells that have undergone nuclear reprogramming will most likely face significant regulatory hurdles before clinical applications commence.
Currently, both ESCs and iPSCs are being tested for cardiac applications in large animals. Rigorous safety studies (focusing primarily on arrhythmiogenicity and oncogenicity) are essential before these pluripotent cells can enter the arena of clinical investigation.
30.7 Future Directions
Going forward (and regardless of investigated cell type), the pursuit of improved methods for cell delivery, means to boost cardiac cell retention, more potent and better-standardized “off the shelf” cellular products, and more apt patient populations is certainly merited [4, 5]. These points are considered individually below.
30.7.1 The Challenge of Low Cell Retention After Cardiac Delivery
Low cell retention after delivery to the heart is a persistent obstacle to successful myocardial regeneration [79, 95]. Numerous studies have investigated short-term cell retention both in experimental animals [96] and in humans [97] and the results have been overwhelmingly disappointing; acute cell retention (i.e., within 24 h of delivery) in the heart is generally <10 %, regardless of the cell type or delivery route. Cells acutely lost from the heart are washed out via the coronary venous system or mechanically ejected via the injection site, while retention rates in beating hearts are markedly lower than in non-beating hearts [79]. Taking into account that studies of bone marrow-derived and heart-derived cells [65, 79, 98] reveal a strong correlation between acute cell retention and long-term functional benefit, there is good reason to believe that: (a) the development of more effective delivery methods, combined with (b) successful means of boosting transplanted cell retention (ensuring that once cells are delivered, they will biologically bond with the host tissue and survive), would significantly enhance the utility of cell therapy.
< div class='tao-gold-member'>
Only gold members can continue reading. Log In or Register a > to continue
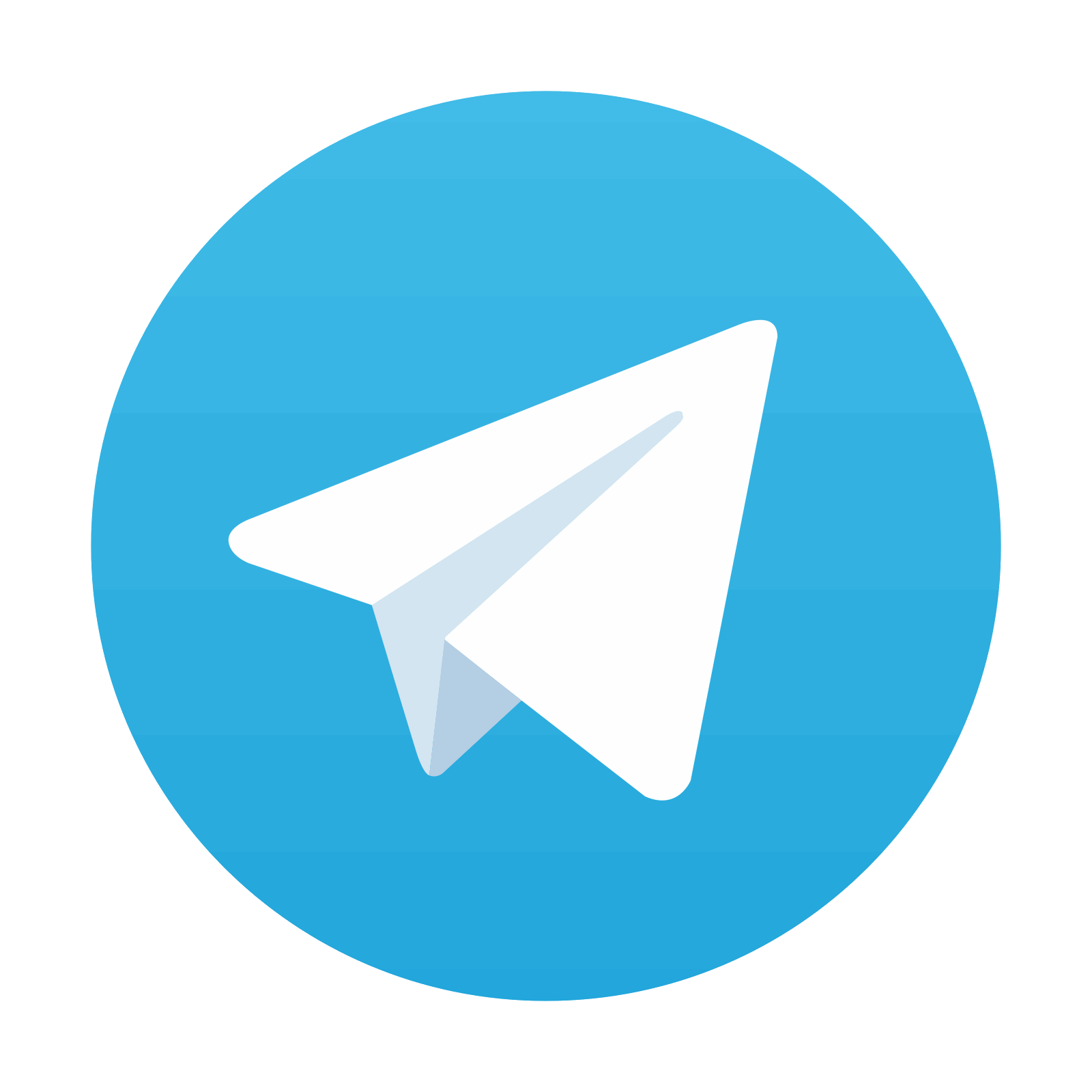
Stay updated, free articles. Join our Telegram channel

Full access? Get Clinical Tree
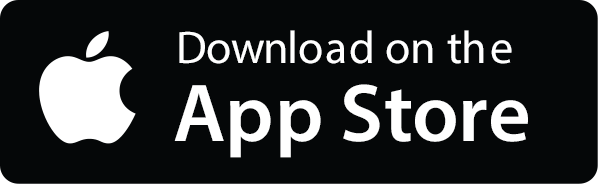
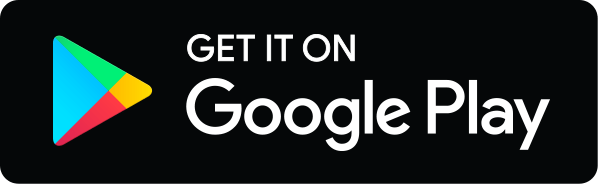