Ischemic heart disease and heart failure continue to be the predominant causes of morbidity and mortality worldwide. Acute myocardial infarction is the most common cause of heart failure and triggers a series of cellular and molecular changes leading to apoptosis, necrosis, hypertrophy of cardiomyocytes, impaired neovascularization, interstitial fibrosis, inflammation, reduced contractility, and pathologic remodeling.1 Despite advances in medical and device therapy, hospitalization rates and mortality for heart failure continue to remain high, with 1 in 5 patients dying within 12 months of diagnosis.2 Medical therapy has centered on the treatment of underlying risk factors and on the initiation of antiplatelet, lipid-lowering, β-adrenergic receptor, and angiotensin antagonist therapy. Interventions designed to facilitate cardiovascular regeneration were not initially pursued because it was assumed that the adult heart is a terminally differentiated postmitotic organ where the number of cardiomyocytes are established at birth, with no potential for regeneration after damage.3
Over the last 2 decades, irrefutable evidence has emerged that challenges these concepts due to the discovery of a population of bone marrow–derived and resident progenitor/stem cells that promote cardiomyocyte and vascular renewal and proliferation. It is now clear that human cardiomyocytes renew throughout a person’s lifespan at a rate that decreases from 1% annual turnover at age 25 to 0.45% at age 75 years.4 Regeneration of infarcted myocardium requires massive cell replenishment, possibly in the order of a billion cardiomyocytes together with functional integration with supporting cell types.5 The adult heart, however, has a relatively low number of cardiac progenitor cells with a low proliferation rate that cannot compensate for the large losses of cardiomyocytes seen after an acute myocardial infarction. Cell-based therapies offer a novel strategy to repair and regenerate injured and nonviable cardiac and vascular tissue.
In the past decade, there has been an influx of clinical trials investigating the safety and efficacy of both autologous and allogeneic stem/progenitor cells for cardiovascular repair and regeneration. To date, phase I and phase II cell therapy trials have been conducted in patients with refractory angina pectoris, acute myocardial infarction, ischemic and nonischemic heart failure, and peripheral vascular disease. While a variety of cell types and delivery techniques have been used, the majority of clinical trials have used a catheter-based approach to target delivery of cells to areas of ischemia or dysfunction. Herein we will review potential mechanisms of action of stem cells, mechanisms of cellular delivery, and cell types currently being studied for cardiovascular regeneration, and review ongoing clinical trials. Characteristics of stem cells being investigated in cardiovascular cell therapy are summarized in Table 68-1.
ESC | iPSC | SM | BMMNC | EPC | MSC | CPC | |
---|---|---|---|---|---|---|---|
Origin of cells | Blastocysts | Fibroblasts | Skeletal muscle | BM | BM, blood | BM, adipose other | Heart |
Differentiate into CMs | Yes | Yes | Unknown | Potential | Yes | Yes | Yes |
Differentiate into endothelial cells | Yes | Yes | No | Yes | Yes | Yes | Yes |
Differentiate into smooth muscle cells | Yes | Yes | No | Potential | Yes | Yes | Yes |
Differentiate into other tissue | Yes | Yes | No | Blood cells | No | Yes | Yes |
Fusion with CMs | Yes | Yes | Yes | Yes | Yes | Yes | Yes |
Paracrine effects | Yes | Yes | Yes | Yes | Yes | Yes | Yes |
Immune rejection | Yes | Unknown | NAa | NAa | NAa | No | No |
Teratoma formation | Yes | Unknown | No | No | No | No | No |
Ventricular arrhythmias | Unknown | Unknown | Yes | No | No | No | No |
Clinical trials | No | No | Yes | Yes | Yes | Yes | Yes |
Mechanisms by which cell therapy leads to cardiovascular repair and regeneration remain a subject of controversy (Fig. 68-1). Although differentiation or transdifferentiation into new cardiac or vascular cells was initially believed to be the primary mechanism, recent studies suggest that stimulation of endogenous precursor cells, secretion of growth factors and cytokines (paracrine effect) that promote vasculogenesis and prevents apoptosis, or rarely, fusion of donor cells with host cells may be potential mechanisms.6 Together, these cellular changes result in enhanced perfusion, reduced infarct size, improved left ventricular remodeling, and ultimately improved cardiac function and patient mortality.
FIGURE 68-1
Potential mechanisms underlying the cardioprotective actions of stem cells: differentiation into cells of cardiac lineages; activation of antiapoptotic signaling; promotion of angiogenesis and reendothelialization; paracrine effects on resident progenitors and myocytes leading to cellular proliferation; and favorable paracrine effects on the extracellular matrix.176 (Reproduced from Sanganalmath SK, Abdel-Latif A, Bolli R, Xuan YT, Dawn B. Hematopoietic cytokines for cardiac repair: mobilization of bone marrow cells and beyond. Basic Res Cardiol. 2011;106:709-33, with permission from Springer.)

The main objective of cell therapy is to deliver the ideal concentration of cells needed for cardiac regeneration with the lowest risk to the patient. The clinical setting and underlying cardiac pathology influence local signaling and affect transplanted cell survival and retention.7 Currently, stem cells can be delivered for cardiac diseases into the coronary arteries or veins, systemically by intravenous injection, or by direct intramyocardial injection using open surgical or transendocardial approaches (Table 68-2).
Mechanism | Description |
---|---|
Cardiovascular Disease | |
1. Intravenous infusion | Cells are delivered intravenously. Relies on physiologic homing signals for cells to home to injured tissues. Advantages: Ease of delivery. Low risk in patients with comorbidities. Can perform multiple infusions over time. Disadvantages: Lower level of cell delivery to target areas. |
2. Intracoronary artery administration | Cells delivered directly to myocardial regions via standard angioplasty balloon catheter into coronary artery lumen with either (1) balloon deflated or nonocclusive or (2) stop-flow method using intermittent balloon arterial occlusion. In the stop-flow method, the balloon is placed at the site of the former infarct-vessel occlusion. With the balloon inflated at low pressures for 2 to 4 minutes, 2 to 3 mL of cell suspension is infused. Balloon inflation prevents backflow of cells while facilitating infusion of cells into the infarcted zone. Advantages: Direct infusion into areas of interest and homogenous cell engraftment. Disadvantages: Cannot deliver cells into occluded arteries. Large cells may cause embolization of coronary microvasculature. Modest cell retention rates compared to intramyocardial injection. |
3. Catheter-based intramyocardial administration | Cells are delivered via a percutaneous catheter either transendocardially or by transcoronary venous injection. Advantages: Less invasive than surgical injection. Procedure can be repeated over time. Disadvantages: Increased risk of perforation. |
3a. Transendocardial injection | Injection needle catheter is advanced retrograde across the aortic valve and positioned against the endocardial surface. Often combined with catheter mapping systems to localize delivery to viable, ischemic, or scarred myocardium. |
3b. Retrograde transcoronary venous injection | Injection needle catheter is advanced into the coronary sinus through the right atrium. Cells are injected directly into the myocardium. Advantages: Utilizes the venous system. Offers alternative delivery mechanism for patients with occluded coronary arteries. |
4. Direct surgical intramyocardial injection | Cells are injected into infarct border zones or ischemic or infarcted myocardium under direct visualization during open revascularization procedures. Advantages: Allows targeting of localized myocardium without disturbing surrounding tissue and vasculature. Disadvantages: Invasive procedure with increased risk to patient. |
5. Engineered monolayer tissue transplantation | Cells are mounted on physical scaffolds to enhance cell adherence and survival. Multiple materials are under investigation. Can be implanted epicardially or by myocardial injection. Advantages: Novel solution to poor cell engraftment and survival. Disadvantages: Regulatory and rejection hurdles. |
Peripheral Vascular Disease | |
Intramuscular | Cells are directly injected into affected muscles of the lower extremity. Goal is to create a collection of cells with paracrine activity in the ischemic area. The fate of injected cells is unknown, and cell retention rates vary between 0.44% and 10% after 4 days. Cells are either injected in a symmetric grid with a fixed number of injections in the ischemic muscle or along the occluded native arteries. The latter approach benefits from preformed collaterals, which have the highest density parallel to the axial arteries. Advantages: Direct delivery of large numbers of cells to target areas. |
Intra-arterial | Cells are directly injected into occluded arteries of the lower extremity. Target limb arteries were selectively cannulated through a transfemoral approach with an over-the-wire catheter balloon that is advanced as distally as possible and positioned proximal to the occlusive vascular lesions, typically at the distal femoral or popliteal artery. At this point, the balloon is inflated to block antegrade blood flow, and the cells are slowly infused over 3 minutes. After infusion, the balloon is deflated, and antegrade blood flow is restored. Advantages: Direct infusion into areas of interest and homogenous cell engraftment. Disadvantages: Difficult to deliver cells to areas subtended by occluded arteries. |
Intravenous infusion of stem cells is associated with the lowest rates of cell retention. Even in the setting of acute myocardial infarction where local release of cytokines enhances homing of stem cells, the majority of intravenously infused cells become trapped in the pulmonary, hepatic, and splenic microvasculature, and few reach areas of infarcted myocardium.8 Moreover, the size of stem cells affects delivery to target areas, with a greater percentage of larger mesenchymal stem cells (20 μm) being trapped in the pulmonary vasculature than smaller bone marrow mononuclear cells (7 μm).9
Intracoronary infusion circumvents the pulmonary and hepatic first-pass effect by delivering cells directly into target areas of myocardium and is the most common strategy used in acute myocardial infarction trials.10 After over-the-wire positioning of a balloon catheter in the stented area of the infarct-related artery, coronary blood flow is stopped for approximately 2 to 4 minutes by inflation of the balloon at low pressures while the cell product is infused into the coronary artery (stop-flow technique). Mechanistically, cessation of flow maximizes contact between stem cells and the microcirculation of the infarct-related artery and optimizes cellular “homing time”; however, studies have demonstrated conflicting results.7 Advantages include direct infusion into the infarct and peri-infarct territory, but intracoronary infusion of larger mesenchymal stem cells has been associated with embolization of the microvasculature.11 Finally, uptake of stem cells into the ischemic myocardium is considerably lower than that achieved by intramyocardial delivery but is better than intravenous administration.
Percutaneous retrograde delivery via the coronary sinus is an alternative method for delivery of cellular products. Although only a few studies have used it for the delivery of stem cells, this technique has been used for many years to deliver cardioplegic solutions during cardiovascular surgery and for protection against myocardial ischemia in patients undergoing high-risk percutaneous coronary intervention.12 The technique involves placement of a catheter into the coronary sinus via cannulation of either the internal jugular or femoral vein. A single or double balloon is inflated, followed by infusion of the cell product for 5 to 30 minutes at a pressure approximately 20 mL higher than sinus pressure.13 In comparison to other methods of cell delivery, retrograde coronary sinus delivery appears to be safe, allow for more homogenous delivery across the myocardium, and have similar retention rates to intramyocardial delivery.13 This technique also allows for delivery of stem cells to ischemic areas of myocardium in patients with occlusive coronary artery disease and severe subtotal aortic stenosis.14
Transendocardial injection is performed percutaneously with retrograde advancement of the injection-needle catheter across the aortic valve and positioned against the endocardial surface. Cells are injected directly into the desired endocardial surface by advancing a small-caliber injection needle into the myocardium.7 Injection sites may be localized to the desired segment of the myocardium by mapping catheter systems. The NOGA injection catheter (Myostar; Cordis Corporation, Miami Lakes, FL) enables 3-dimensional electromechanical mapping of the left ventricle and provides real-time voltage and linear shortening data for delivery of transendocardial injections. In humans, normal regional myocardial function is indicated by high electrical activity and high local linear shortening. The presence of an abnormal or low unipolar potential (≤6 mV) and impaired mechanical activity (low linear shortening ≤4%) is interpreted as abnormal electromechanical activity and characterizes infarcted areas. When mechanical activity is impaired but electrical activity is normal or near normal, the area of interest can represent ischemic, stunned, or hibernating myocardium (Fig. 68-2).15 Direct intramyocardial cell delivery can also be performed under direct visualization during coronary bypass surgery or using a percutaneous laparoscopic approach (mini-thoracotomy).16 Although these techniques are more invasive and associated with adverse effects, including perforation and pericardial effusion, cell retention is greatest with intramyocardial injection, and higher numbers of cells can be injected without risk of downstream embolization.17 While routinely used in clinical trials in patients with chronic heart failure (Table 68-3), its safety has not been studied in the setting of acute myocardial infarction, where injured myocardium is more vulnerable to rupture.7
FIGURE 68-2
Unipolar voltage and local linear shortening maps with the corresponding 2-dimensional quantitative polar maps. Red color indicates the normally low unipolar voltage at the base of the heart (mitral valve area) with concomitant loss of electrical activity at the basal septal and anterior regions and akinesis in the entire septal wall and partial akinesis in the anterior wall. Blue color indicates a normal voltage signal (normal myocardium), whereas green and yellow colors indicate decreased viability and wall motion at the infarct border zone, respectively. The obvious discrepancy between viable myocardium and severe wall motion disturbance in the anteroseptal areas suggests the presence of hibernating myocardium. Brown points represent the locations of the NOGA-guided intramyocardial injections at the border zone of infarction, in diaphragmatic septal areas. LLS, local linear shortening; RAO, right anterior oblique.15 (Reprinted by permission from Macmillan Publishers Ltd: Gyongyosi M, Dib N. Diagnostic and prognostic value of 3D NOGA mapping in ischemic heart disease. Nat Rev Cardiol. 2011;8:393-404.)

Study | No. of Patients | Follow-Up (Months) | No. of Cells Injected | Method of Delivery | Significant Clinical End Points | Adverse Outcomes |
---|---|---|---|---|---|---|
Skeletal Myoblasts | ||||||
Chronic Heart Failure | ||||||
MARVEL158 | 23 | 6 | 4.0 × 108 or 8.0 × 108 | TCIM | None | ↑Ventricular tachycardia |
SEISMIC33 | 40 | 6 | 1.5-8.0 × 108 | TCIM | None | None |
CAUSMIC159 | 23 | 12 | 0.3-6.0 × 108 | TCIM | Improved NYHA (P <.05) Improved MLHFQ score (P = .004) | |
MAGIC160 | 97 | 6 | 4.0 × 108 or 8.0 × 108 | TEIM | None | Arrhythmia |
Bone Marrow Mononuclear Cells | ||||||
Acute Myocardial Infarction | ||||||
REGENT66 | 200 | 6 | 1.8 × 108 | Intracoronary | EF + 5% (P = .007)a | None |
BONAMI161 | 101 | 3 | 9.8 ± 0.9 × 107 | Intracoronary | Improved myocardial viability (P = .06) | |
HEBE49 | 200 | 4 | 3.0 ± 1.6 × 108 | Intracoronary | None | None |
REPAIR-AMI45 | 204 | 4 | 2.4 ± 1.7 × 108 | Intracoronary | EF + 5.5% (P = .001); ↓death, recurrent MI, revascularization | |
ASTAMI51,52 | 100 | 6, 36 | ~6.8 × 107 | Intracoronary | None | None |
FINCELL46 | 80 | 6 | 4.0 ± 2.0 × 108 | Intracoronary | EF + 4.0% (P = .03) | None |
TIME47 | 120 | 6 | 1.5 × 108 | Intracoronary | None | None |
Late TIME48 | 87 | 6 | 1.5 × 108 | Intracoronary | None | None |
SCAMI162,163 | 42 | 6, 36 | 3.2 × 108 | Intracoronary | EF + 5.6% (P = .03) | None |
BOOST43 | 60 | 18 | 2.5 ± 9.4 × 109 | Intracoronary | None | None |
TOPCARE-AMI41,42,164 | 20 | 4, 12, 60 | 2.1 ± 7.5 × 108 | Intracoronary | EF + 8.0% (P <.001) | None |
SWISS-AMI165 | 200 | 4 | 1.5 ± 1.2 × 108 | Intracoronary | None | None |
Chronic Heart Failure | ||||||
CELLWAVE58 | 103 | 4 | Shock wave + 2.4 ± 1.7 × 108 | Intracoronary | EF + 2.2% (P = .02), improved regional wall thickening, ↓MACE (HR, 0.58; P = .02) | |
TAC-HFT92 | 65 | 12 | Not reported | TCIM | Improved MLHFQ score (P = .005) | |
FOCUS-CCTRN60 | 92 | 6 | 9.9 ± 0.6 × 107 | TCIM | EF + 2.7% (P = .03) | None |
FOCUS-HF166 | 30 | 6 | 3.0 × 107 | TCIM | Improved MLHFQ (P = .009) | |
Refractory Angina | ||||||
Beeres et al167 | 25 | 12 | 8.4 ± 2.9 × 107 | TCIM | ↓CCS (P <.01), ↑QALY (P <.01) | |
CD34+/CD133+ Bone Marrow Mononuclear Cells | ||||||
Acute Myocardial Infarction | ||||||
Bartunek et al67 | 35 | 4 | 1.3 ± 0.2 × 107 | Intracoronary | EF + 7.1%, defect size –5.5% | ↑In-stent restenosis |
Quyyumi et al168 | 31 | 6 | 5/10/15 × 106 | Intracoronary | None | None |
Stamm et al169 | 55 | 6 | 5.8 × 106 | TEIM | EF + 9% (P = .007) | None |
REGENT66 | 200 | 6 | 1.9 × 106 | Intracoronary | EF + 5% (P = .007)a | None |
Chronic Heart Failure | ||||||
Vrtovec et al69 | 110 | 60 | 1.1 ± 0.3 × 106 | Intracoronary | EF + 5.7% (P = .02), 6-min walk +133 m (P <.001), decreased mortality (P = .01) | |
DANCELL-CHF70 | 32 | 12,84 | 1) 6.5 ± 3.8 × 108 2) 8.9 ± 3.6 × 108 | Intracoronary | None | None |
Refractory Angina | ||||||
Losordo et al72 | 167 | 12 | 1 × 105 cells/kg or 5 × 105 cells/kg | TCIM | ↓Angina (P = .04) | None |
Wang et al170 | 112 | 3, 6, 12 | 5.6 ± 2.3 × 107 | Intracoronary | ↓Angina (P <.01), ↑myocardial perfusion (P <.001) | None |
Mesenchymal Stem Cells | ||||||
Acute Myocardial Infarction | ||||||
Gao et al11 | 43 | 12, 24 | 3.1 ± 0.5 × 106 | Intracoronary | None | Arterial occlusion during infusion |
Hare et al88 | 53 | 6 | 6, 16, 50 × 105 cell/kg | Intravenous | EF + 5.2% (P = .003), ↓ventricular tachycardia episodes (P = .003) | |
Refractory Angina | ||||||
Haack-Sorensen et al89 | 31 | 12 | 2.2 × 107 | TCIM | ↑MET (P <.001), ↓CCS (P <.001), ↓SAQ and QoL (P <.001) | |
Chronic Heart Failure | ||||||
TAC-HFT92 | 65 | 12 | Not reported | TCIM | Improved MLHFQ score (P = .02), improved 6-min walk (P = .03), reduced infarct size (P = .004) | |
C-CURE91 | 48 | 6, 24 | 6/12 × 108 | TCIM | EF + 6.5% (P <.01), LVESV, ↑6-min walk test | |
POSEIDON90 | 30 | 13 | 2/10/20 × 107 | TCIM | None | None |
Ixmyelocel-T Cells | ||||||
Ixmyelocel-T93 | 61 | 12 | ≤1.5 × 106 | TCIM | ↓ MACE (P <.05), ↑6-min walk distance, improved MLHFQ score | |
Ixmyelocel-T95 | 114 | 12 | Not reported | TCIM | ↓All-cause deaths, CV and ADCHF admissions (P = .034) | |
Adipose-Derived Progenitor Cells | ||||||
Chronic Heart Failure | ||||||
PRECISE103 | 36 | 6, 18 | 4.2 × 107 | TCIM | Improved LV mass (P <.001) and WMSI (P = .04) | |
C-kit+ Cardiac Progenitor Cells | ||||||
Chronic Heart Failure | ||||||
SCIPIO116 | 33 | 12 | ≤1 × 106 | Intracoronary | EF + 15% (P = .013),↓infarct size (P = .04),↑LV viable mass (P = .04) | |
Cardiosphere and Cardiosphere-Derived Cells | ||||||
Chronic Heart Failure With Recent Myocardial Infarction (<4 months) | ||||||
CADUCEUS171 | 31 | 12 | 12.5/17.3/25 × 106 | Intracoronary | ↑LV viable mass (P <.001) | None |
Granulocyte Colony-Stimulating Factor (G-CSF) | ||||||
Acute Myocardial Infarction | ||||||
MAGIC132 | 27 | 6 | 10 μg/kg G-CSF × 4 days | Subcutaneous | EF + 6.4% (P = .005) | ↑In-stent restenosis |
FIRSTLINE-AMI133 | 30 | 12 | 10 μg/kg G-CSF × 6 days | Subcutaneous | EF + 8% (P <.003) | None |
STEMMI134 | 78 | 6 | 10 μg/kg G-CSF × 6 days | Subcutaneous | None | None |
REVIVAL-2135 | 114 | 6 | 10 μg/kg G-CSF × 5 days | Subcutaneous | None | None |
Chronic Heart Failure | ||||||
TOPCARE-G-CSF137 | 32 | 60 | 5 or 10 μg/kg G-CSF × 5 days or G-CSF–mobilized mononuclear cells | Subcutaneous or intracoronary | None | None |
Less frequently used methods of delivery include direct surgical intramyocardial injection and engineered monolayer tissue transplantation. Both methods are invasive and require direct visualization of the myocardium. They are summarized in Table 68-2.
Human embryonic stem cells are pluripotent cells derived from blastocysts that can differentiate into a multitude of adult cells including cardiomyocytes, endothelial, and smooth muscle cells.18,19 After aggregation to form embryoid bodies, embryonic stem cells form spontaneously contracting cardiomyocytes within 1 to 4 days.20 When injected into infarcted rodent myocardium, embryonic stem cell–derived cardiomyocytes regenerated the infarcted myocardium, leading to reduced ventricular dilation and preserved regional and global contractile function.21,22 However, their therapeutic use is complicated by immunologic rejection, propensity for teratoma formation, and ethical considerations.21 The risk of teratoma formation may be mitigated by grafting ex vivo predifferentiated embryonic stem cell–derived cardiomyocytes.
Induced pluripotent stem cells (iPSCs) mitigate the legal and ethical concerns related to embryonic stem cells. iPSCs are somatic cells that have been reprogrammed to express an embryonic phenotype. Seminal experiments by Takahashi and Yamanaka used oct4, sox2, nanog, lin28, klf4, and c-myc transgenes to reprogram adult murine somatic cells into iPSCs with subsequent differentiation into functional cardiomyocytes.23,24 Treatment of human iPSCs with activin A and bmp4 can direct differentiation into human cardiac fibroblasts,21 and injection of these transgenes into murine models of myocardial infarction results in improved cardiac function and adverse ventricular remodeling.25 iPSCs can also be generated using microRNAs. Mouse fibroblasts converted to the cardiomyocyte phenotype by transfection with microRNAs 1, 133, 208, 499, and JAK inhibitor I expressed cardiomyocyte-specific genes and proteins and exhibited sarcomeric organization, spontaneous calcium oscillations, and mechanical contractions characteristic of cardiomyocytes.26 Despite these promising results, use of iPSCs in humans has been limited due to concerns over their oncogenicity. Potent oncogenes (c-myc) used for reprogramming, involvement of the p53 pathway, and the epigenetic memory of iPSCs increase their risk for somatic tumor development.27,28
Skeletal myoblasts are skeletal muscle precursors that possess a contractile phenotype and are relatively resistant to ischemia and oxidative stress.29 Although skeletal myoblasts can differentiate into functionally competent myofibers after engraftment, they suffer a high attrition rate, and only 7.4% of transplanted cells survive at 72 hours.30 Surviving myofibers fail to develop intercalated discs and remain electromechanically isolated from host myocardium, which may promote the generation of ventricular arrhythmias.31 In human studies, autologous skeletal myoblasts harvested from the quadriceps muscle and cultured in vitro were transplanted using the NOGA-guided catheter into areas of scarred myocardium (unipolar voltage <7.0 mV). Although initial studies claimed benefits, there was an associated increase in arrhythmias (see Table 68-3).29,32 The Myoblast Autologous Grafting in Ischemic Cardiomyopathy (MAGIC) trial randomized 97 patients undergoing coronary artery bypass surgery with reduced left ventricular ejection fraction (LVEF; <35%) to receive either autologous myoblast injections (4.0 × 108 or 8.0 × 108 cells) or placebo. At 6 months, myoblast transfer did not improve regional or global LVEF compared to controls. While cell therapy was associated with a higher number of arrhythmic events, it did not result in significant differences in major adverse cardiac events.16 However, administration of periprocedural amiodarone may be successful in reducing the number of arrhythmic events.33
Ischemic tissue damage stimulates recruitment and homing of endogenous bone marrow–derived progenitor and stem cells via the release of numerous cytokines including granulocyte colony-stimulating factor (G-CSF), stem cell factor, vascular endothelial growth factor, stromal cell–derived factor-1 (SDF-1), and erythropoietin.34 Locally derived SDF-1 augments vasculogenesis and subsequently contributes to ischemic neovascularization in vivo by augmenting endothelial progenitor cell recruitment to ischemic tissues.35 In addition, the SDF-1/CXCR4 axis regulates progenitor cell proliferation and survival as well as several intracellular signaling pathways.36
Bone marrow–derived stem and progenitor cells are attractive candidates for cell therapy because of their accessibility and renewability. The human bone marrow houses several progenitor cell populations including hematopoietic stem cells, endothelial progenitor cells, mesenchymal stem cells, and others. The largely paracrine mechanisms underlying the regenerative potential of bone marrow–derived stem/progenitor cells include (1) release of antiapoptotic cytokines that improve survival of cardiomyocytes in the infarct border zone,37 (2) secretion of angiogenic factors that promote angiogenesis and vasculogenesis,38 (3) stimulation of resident cardiac and vascular stem cells,39 and (4) induction of mitosis.40 To date, unselected bone marrow mononuclear cells, selected CD34+ progenitor cells, selected CD34+/CD133+ progenitor cells, and mesenchymal stem cells have been employed in human clinical trials in a variety of clinical settings.
Several trials have been conducted in patients with acute ST-segment elevation myocardial infarction (MI) using intracoronary infusions of bone marrow mononuclear cells (see Table 68-3). The Transplantation of Progenitor Cells and Regeneration Enhancement in Acute Myocardial Infarction (TOPCARE-AMI) trial was an early randomized controlled trial to demonstrate functional improvement.41 Cells were harvested by bone marrow aspiration and delivered into the infarct-related artery using the stop-flow technique at 4.9 ± 1.5 days after ST-segment elevation MI in 29 subjects, and circulating mononuclear cells were given to 30 controls. After 4 months, LVEF significantly increased (50% ± 10% to 58% ± 10%, P <.001), and end-systolic volumes significantly decreased (54 ± 19 mL to 44 ± 20 mL, P <.001) without differences between the 2 cell groups.42 In the Bone Marrow Transfer to Enhance ST-Elevation Infarct Regeneration Trial (BOOST), 60 patients were randomized to receive either autologous bone marrow mononuclear cells (mean 2.5 × 109) or placebo, delivered 4.8 ± 1.3 days after ST-segment elevation MI and percutaneous coronary intervention (PCI).43 At 6 months, LVEF was significantly improved in the cell therapy versus control group (6.7% vs 0.7%, respectively; P = .0026). However, early improvements in LVEF did not persist at 18 months and did not translate into decreased major adverse cardiovascular events at 5 years.44 The Reinfusion of Enriched Progenitor Cells and Infarct Remodeling in Acute Myocardial Infarction (REPAIR-AMI) trial is the largest trial to date using bone marrow mononuclear cells in acute MI.45 Two hundred four patients were randomized to receive intracoronary infusion of either bone marrow mononuclear cells (2.4 ± 1.7 × 108 cells) or placebo after 4.4 ± 1.4 days after ST-segment elevation MI and PCI. At 4 months, the improvement in LVEF was significantly greater in the cell therapy group compared to controls (5.5% ± 7.3% vs 3.0% ± 6.5%, P = .01).45 At 12 months, the occurrence of major adverse cardiovascular events was significantly lower in the cell therapy group compared to controls (23 vs 40 events, respectively, P = .01).45 Finally, the Finnish Stem Cell (FINCELL) study randomized 80 subjects to receive intracoronary infusion of autologous bone marrow mononuclear cells (4.0 ± 2.0 × 108 cells) or placebo 2 to 6 days after ST-segment elevation MI and PCI.46 At 6 months of follow-up, the bone marrow mononuclear cells treatment group had significant improvement in LVEF compared to the placebo group (4.0% ± 11.2% vs –1.4% ± 10.2%, P = .03). There were no differences in major adverse cardiovascular events or arrhythmias between the 2 groups.46
In contrast, several trials have failed to demonstrate benefit from intracoronary administration of bone marrow mononuclear cells. The randomized, double-blind, placebo-controlled Timing in Myocardial Infarction Evaluation trials (TIME and Late TIME) found that intracoronary infusion of bone marrow mononuclear cells either 3 to 7 days (TIME) after MI or 14 to 21 days (Late TIME) after MI had no effect on recovery of global or regional left ventricular function compared to placebo.47,48 The Intracoronary Infusion of Mononuclear Cells From Bone Marrow or Peripheral Blood Compared With Standard Therapy in Patients After Acute Myocardial Infarction Treated by Primary Percutaneous Coronary Intervention (HEBE) trial randomized 200 patients with acute MI to receive either intracoronary infusion of bone marrow mononuclear cells, circulating mononuclear cells, or standard therapy without placebo infusion 3 to 8 days after acute MI.49 At 4 months, there were no significant differences in LVEF, infarct size, or major adverse cardiac events.50 Similarly, the Intracoronary Injection of Mononuclear Bone Marrow Cells in Acute Myocardial Infarction (ASTAMI) trial reported no significant change in LVEF or major adverse cardiovascular event rates at 6 or 36 months.51,52
Several meta-analyses of trials evaluating efficacy of intracoronary autologous bone marrow mononuclear cell therapy in ST-segment elevation MI have been conducted.53–56 In a recent meta-analysis of 22 trials enrolling 2037 patients, of whom 1218 received bone marrow mononuclear cell therapy, treatment was found to be safe and was associated with modest improvements in LVEF (+2.1%), reduction in infarct scar size (–2.7%), and preservation of left ventricular end-systolic volume (–4 mL) without increase in short-term adverse event rate.56 Subgroup analyses revealed that improvements in LVEF were more likely among patients (1) with lower baseline LVEF, (2) who received more than 1 × 106 cells, and (3) who received treatment 5 to 30 days after infarction.54 In another meta-analysis of 50 studies enrolling 2625 patients with ischemic heart disease (either acute or chronic), treatment with bone marrow mononuclear cells was associated with a modest improvement in LVEF (+3.95%), smaller infarct size (–4.03%), and reduced LV end-systolic volume (–5.23 mL).57 Similarly, subgroup analysis revealed that improvements in LVEF were greater among patients with (1) lower baseline LVEF and (2) patients who received more than 4 × 107 cells.57 Both analyses highlight the importance of timing, dose, and MI size as determinants of response to stem cell therapy in patients with acute MI. Importantly, compared to patients who received standard therapy, patients treated with bone marrow mononuclear cells experienced significant decreases in all-cause mortality (odds ratio [OR], 0.39), cardiac mortality (OR, 0.41), recurrent MI (OR, 0.25), and in-stent thrombosis (OR, 0.34). There were no statistical differences in ventricular arrhythmias between groups.57
The Effect of Shock Wave-Facilitated Intracoronary Cell Therapy on LVEF in Patients With Chronic Heart Failure (CELLWAVE) trial randomized 103 patients with chronic postinfarction heart failure in 2 steps.58 Patients were first randomized (2:2:1; single-blind) to receive echocardiographically guided low-dose (0.014 mJ/mm2), high-dose (0.051 mJ/mm2), or placebo shock wave targeted to the left ventricular anterior wall 24 hours prior to cell administration. Preconditioning of chronic ischemic tissue with shock wave therapy has been shown to improve recruitment of circulating endothelial progenitor cells via enhanced expression of chemoattractant factors (ie, SDF-1, vascular endothelial growth factor [VEGF]) in animal models.59 Patients receiving shock wave pretreatment were then randomized in a second step (1:1; double-blind) to receive intracoronary infusion of either bone marrow mononuclear cells or placebo, whereas patients receiving placebo shock wave received intracoronary infusion of bone marrow mononuclear cells.58 At 4 months, compared to patients receiving shock wave therapy alone, patients receiving shock wave therapy and bone marrow mononuclear cells had significantly improved LVEF (1.0% vs 3.2%, respectively, P = .02), regional wall thickening (0.5% vs 3.6%, respectively, P = .01), and decreased incidence of major adverse cardiac events (hazard ratio, 0.58; P = .02).58 The Transendocardial Delivery of Autologous Bone Marrow Mononuclear Cells on Functional Capacity, Left Ventricular Function, and Perfusion in Chronic Heart Failure (FOCUS-CCTRN) trial randomized 92 patients with ischemic cardiomyopathy with LVEF ≤45% to receive transendocardial injection of 9.9 ± 0.6 × 107 bone marrow mononuclear cells or placebo in a 2-to-1 ratio. At 6 months, patients who received bone marrow mononuclear cells demonstrated significant improvements in LVEF compared to control (1.4% vs –1.3%, P = .03).60 However, no change in end-systolic volume, maximal oxygen consumption, or reversibility of ischemia on single-photon emission computed tomography was observed. In exploratory analysis, higher CD34+ or CD133+ cell counts were associated with greater absolute unit increase in LVEF. Every 3% higher level of CD34+ cells was associated with an average of 3.0% greater absolute unit increase in LVEF in multivariable regression modeling. Similarly, every 3% higher level of CD133+ cells was associated with an average of 5.9% greater absolute unit increase in LVEF.60 These findings support the concept that both cell type and number of cells infused may be important factors in determining the benefit derived from cell therapy.
Whether selected populations of bone marrow mononuclear cells enriched for progenitor cells are likely to be more effective than unselected mononuclear cells has been explored in several early-phase trials. CD34+ cells are enriched for progenitor cells and localize more avidly to peri-infarct regions than unselected cells.61 CD34+ bone marrow mononuclear cells are relatively uncommon (1%-2%) and are capable of transdifferentiating into hematopoietic, endothelial, and smooth muscle cells, and rarely to cardiomyocytes in vivo.62,63 Coexpression of CD34 with CD133 identifies cells enriched for early precursor cells.64 In addition, coexpression of VEGF receptor-2 on CD34+ cells may constitute a phenotypically and functionally distinct population of cells enriched for endothelial progenitors.65
The Myocardial Regeneration by Intracoronary Infusion of Selected Population of Stem Cells in Acute Myocardial Infarction (REGENT) trial randomized 200 patients with acute MI and reduced LVEF (<40%) to receive intracoronary infusion of 1.8 × 108 unselected bone marrow mononuclear cells, 1.9 × 106 selected CD34+/CXCR4+ cells, or placebo 7 days following ST-segment elevation MI.66 At 6 months, treatment with either CD34+ or mononuclear cells did not improve LVEF or volumes compared to controls. However, in patients with baseline postinfarction LVEF <37% (median), infusion of unselected cells had a median LVEF improvement of 6.0% (P = .007), and selected CD34+/CXCR4+ cells had a median LVEF improvement of 7.0% (P = .01), indicating that cell therapy may provide the most benefit in patients with the most severely impaired LVEF after MI.66 In addition, there was a trend toward a greater proportion of patients receiving CD34+/CXCR4+ cells to improve LVEF compared to those receiving unselected cells, suggesting that selected CD34+ cells may be more therapeutic than unselected mononuclear cells.66 Finally, Bartunek et al67 randomized 35 patients with acute MI treated with PCI to receive intracoronary administration of CD133+/CD34+ bone marrow cells (1.3 ± 2.2 × 107 cells) 11.6 ± 1.4 days after infarction or standard medical care. At 4 months, patients receiving cell therapy experienced improvements in LVEF (from 45.0% ± 2.6% to 52.1% ± 3.5%, P <.05) and perfusion defect size (from 28.0% ± 4.1% to 22.5% ± 4.1%, P <.05) compared to controls. However, patients receiving intracoronary cell therapy had significantly higher rates of in-stent restenosis.67
Vrtovec et al68 randomized 110 patients with dilated cardiomyopathy to receive intracoronary administration of CD34+ cells (1.1 ± 0.3 × 106 cells) or placebo. CD34+ cells were mobilized with G-CSF and collected via apheresis. Patients underwent myocardial scintigraphy, and cells were injected in the artery supplying segments with the greatest perfusion defect. At 1 and 5 years, CD34+ cell therapy was associated with increased LVEF, increased 6-minute walk distance, and decreased N-terminal B-type natriuretic peptide.68,69 LVEF improvement was more significant in patients with higher myocardial homing of injected cells as determined by cell labeling.69 During the 5-year follow-up, mortality was lower in the cell therapy group compared to controls (14% vs 35%, P = .01).69 The Danish Stem Cell Study–Congestive Heart Failure (DANCELL-CHF) trial studied the benefit of repeated bone marrow mononuclear cell infusions in patients with chronic ischemic heart failure.70 Thirty-two patients with LVEF <45% received 2 treatments of intracoronary CD34+ bone marrow stem cells 4 months apart. Patients received a mean of 6.5 ± 3.8 × 108 bone marrow cells on the first infusion and 8.9 ± 3.6 × 108 cells on the second infusion. At 12 months, there was no change in LVEF.70 At 7 years, post hoc analysis revealed increased CD34+ cell count was significantly associated with survival (hazard ratio, 0.9; P = .04), indicating that repeated injections of high numbers of CD34+ cells may improve long-term survival in patients with chronic ischemic heart failure.71
In a phase II randomized controlled trial, 167 patients with refractory angina were randomized to receive 1 of 2 doses (1 × 105 or 5 × 105 cells/kg) of mobilized autologous CD34+ cells or an equal volume of placebo.72 Treatment was distributed into 10 sites of ischemic but viable myocardium as determined by the NOGA mapping system, as described previously. At 6 months, patients in the low-dose cell therapy group had significantly decreased angina frequency and improved exercise tolerance as compared to patients receiving placebo. Interestingly, there was a lack of dose response, and patients receiving high-dose cell therapy did not experience the same improvements in angina frequency and exercise tolerance seen in the low-dose cell therapy group.72 Clinical trials evaluating the use of CD34+ bone marrow mononuclear cells in the treatment of cardiovascular diseases are ongoing and are summarized in Table 68-4.
Clinical Trial Identifier and Phase | Stem Cell Type | Cardiac Condition | No. of Patients | Method of Delivery | Primary Outcomes |
---|---|---|---|---|---|
NCT02059512 Phase III | BMMNC | Ischemic heart failure | 100 | Intracoronary | All-cause mortality |
NCT01569178 Phase III | BMMNC | Ischemic heart failure | 3000 | Intracoronary | All-cause mortality |
NCT01693042 Phase III | BMMNC | Ischemic heart failure | 676 | Intracoronary (single vs repeat injections) | Mortality |
NCT01969890 Phase III | G-CSF BMMNC mobilization | Ischemic heart failure | 1530 | Intravenous | Death, MI, hospitalization |
NCT00936819 Phase II | EPC | Ischemic heart failure | 100 | Intracoronary | LVEF |
NCT01508910 | CD34+ | Refractory angina | 291 | TCIM | Exercise time, angina frequency |
NCT00950274 Phase II/III | CD133+ | Ischemic heart failure, CABG | 142 | TEIM | LVEF |
NCT01753440 Phase II/III | MSC | Ischemic heart failure, CABG | 30 | TEIM | LVEF, myocardial segmental perfusion |
NCT01394432 Phase III | MSC | Ischemic heart failure | 50 | TCIM | LVEF |
NCT01652209 Phase III | MSC | Ischemic heart failure | 135 | Intracoronary | LVEF |
NCT01759212 Phase III | MSC (allogeneic) | Ischemic heart failure, LVAD | 5 | TEIM | Myocardial perfusion |
NCT01770613 Phase II | MSC (allogeneic) | Ischemic heart failure | 50 | Intravenous | MACE |
NCT01458405 Phase II | MSC (allogeneic) | Ischemic heart failure | 274 | Intracoronary | Infarct size |
NCT02032004 Phase III | MSC (allogeneic) | Heart failure (all etiologies) | 1730 | TCIM | Time to first heart failure–related major adverse event |
NCT02123706 Phase IIa | MSC (allogeneic) | Nonischemic heart failure | 20 | Intravenous | SAE |
NCT02052427 Phase II | ADRC | Ischemic heart failure | 45 | TCIM | Change in MLHFQ |
NCT01758406 Phase II | CSC | Ischemic heart failure | 50 | Intracoronary | Death, arrhythmia, hospitalizations |
NCT02099500 Phase II | ADRC | Peripheral vascular disease | 200 | Intramuscular | ABI, adverse events |
NCT01483898 Phase III | Multicellular Ixmyelocel-T | Peripheral vascular disease | 41 | Intramuscular | Amputation-free survival |
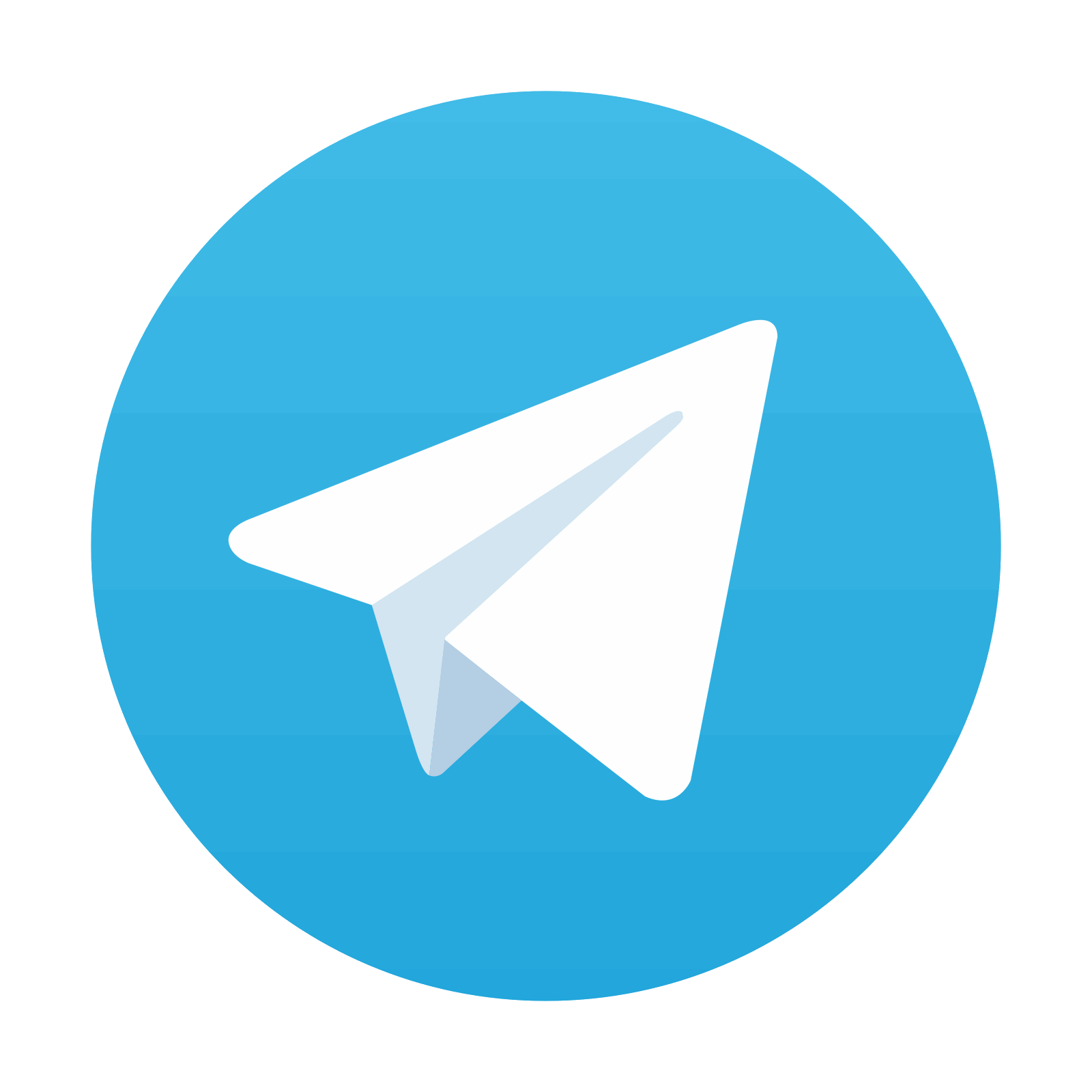
Stay updated, free articles. Join our Telegram channel

Full access? Get Clinical Tree
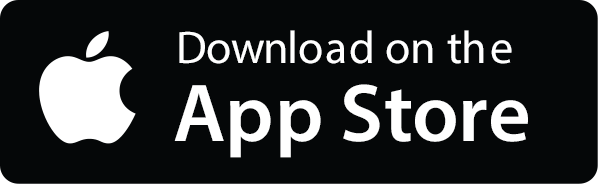
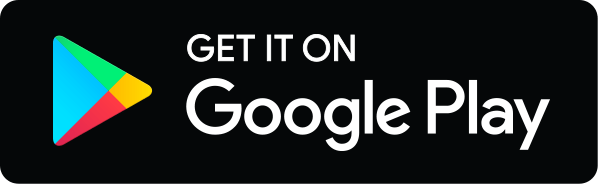
