Fig. 24.1
Distinct differences are observed between pluripotent (a) and multipotent (b) stem cell populations. Schematic highlighting the major differences between pluripotent (i.e., ESC and hiPSC) versus multipotent (i.e., myogenic stem cells, c-kit stem cells, MSCs, SP cells, etc.) stem cell populations
Allotransplantation of Pancreatic Islets
Diabetes mellitus is a heterogeneous disease with a subpopulation of patients having such brittle disease that control with insulin supplementation is inadequate with patients having life-threatening episodes (i.e., seizures, hypoglycemic events, coma, etc.). Due to the limited number of pancreatic organs available for transplantation, isolated islets have been utilized with success. Initial studies were undertaken at the University of Alberta in Edmonton, Canada, with limited success in the 1970s [7]. More recently, increased success has been achieved using the Edmonton Protocol, which uses an enzyme cocktail for pancreatic digestion that is more protective of the fragile islets obtained from cadaveric organs [8]. Typically, one million islets are delivered into the portal vein of the diabetic recipient using ultrasound guidance. Following portal vein delivery, these allogeneic islets are embolized in the liver. This Edmonton protocol has increased insulin independence from approximately 15 to 80 % following alloislet transplantation [8, 9]. Future studies will further examine the impact of the Edmonton protocol and other sources of islet tissues including human-induced pluripotent stem cell (hiPSC) -derived islets or porcine islets for the treatment of diabetes [10]. Collectively, these SCT strategies focused on BMT and alloislet transplantation serve as a platform to experimentally examine the safety and efficacy of SCT for cardiovascular disease (◘ Fig. 24.1).
Cell Sources for SCT for Cardiovascular Disease
Embryonic Stem Cells (ESCs )
Embryonic stem cells were first isolated from the inner cell mass of the mouse blastocyst in 1981 [11]. ESCs have an unlimited proliferative capacity, a normal karyotype, and maintain high telomerase activity. These cells are pluripotent as they are able to differentiate into all three germ layer derivatives and able to give rise to every lineage with the exception of the extraembryonic cells [11, 12]. They have been an invaluable tool for the generation of gene disruption models in the mouse model system. Human embryonic stem cells (hESCs) were first isolated by Dr. Jamie Thomson and his colleagues in 1998 [13]. Due to ethical issues surrounding the isolation of hESCs from human embryos, the NIH developed guidelines (2009) to guide NIH-funded research and established an NIH hESC Registry [14]. Studies utilizing mouse and human ESCs have demonstrated efficient and directed cardiomyocyte differentiation (◘ Fig. 24.1) [15, 16]. Previous studies have demonstrated positive results using hESC-derived cardiomyocytes as a cell therapy source for cardiac regeneration in small animal models [17]. Recently, preclinical studies utilized a nonhuman primate model and delivered 1 billion hESC-derived cardiomyocytes following myocardial injury with evidence of remuscularization [18]. While these studies produced promising results, they were limited by the large number of cells needed for delivery, arrhythmic complications of the graft, maturation of the donor cell population, and the need for immunosuppression therapy.
Human-Induced Pluripotent Stem Cells (hiPSCs )
Recent scientific advances have demonstrated the capacity of adult somatic cells (i.e., skin cells) to be reprogrammed using defined transcription factors into pluripotent stem cells, known as human-induced pluripotent stem cells (hiPSCs) [19–21]. In 2006, the Yamanaka laboratory identified 24 transcription factors that were differentially enriched in mouse embryonic stem cells [22]. Yamanaka’s research team determined that the combination of Oct4, Sox2, Klf, and c-Myc, when virally transduced into mouse fibroblasts, reprogrammed the fibroblasts into embryonic-like stem cells. Therefore, these cells were termed induced pluripotent stem cells [22]. In 2007, this technology was translated into the development of human iPSCs by both the Yamanaka and Thomson laboratories independently [20, 21]. Importantly, the hiPSCs retain the genetic blueprint of the patient and can be differentiated into a variety of cell types. This innovative technology allowed for an unprecedented opportunity to study human diseases in vitro and to develop and test novel cell therapies.
The ability to generate high purity, functional cardiomyocytes is a transformative step in the development of cardiac disease models. The current understanding of embryonic cardiac development and differentiation of embryonic stem cells has been applied to the development of several differentiation protocols that reliably direct hiPSCs to the cardiac lineage (◘ Fig. 24.1) [23–25]. These differentiation protocols include the temporal modulation of the BMP, TGF-β/activin/nodal, WNT/β-catenin, and FGF pathways to specify hiPSCs to a cardiac fate in a multistep process involving epithelial to mesenchymal transition , mesodermal and cardiac specification resulting in functional cardiomyocyte-like cells. While cardiomyocytes can be derived from hiPSCs , the gene expression profiling and physiological characterization indicate that they are immature (i.e., fetal-like cardiomyocytes). Compared to adult human cardiomyocytes, the hiPSC-derived cardiomyocytes are smaller, circular rather than rodlike shaped and have a less organized sarcomeric structure resulting in decreased force generation [25–27]. hiPSC-derived CMs develop more organized cardiac sarcomeric structure during differentiation, but have a morphology that is more comparable to fetal cardiomyocytes than rod-shaped adult cardiomyocytes. Additionally, hiPSC-derived CMs express genes such as smooth muscle actin, connexin 45, and MLC2a are expressed in immature cardiomyocytes. Similar to immature or fetal cardiomyocytes, hiPSC-derived CMs do not have well-developed T-tubules, and they rely primarily on glucose for metabolism and have less mature calcium handling [26, 27]. Strategies to generate more mature hiPSC-derived CMs are a research focus and will be essential for future advancement of the field.
While a number of small animal models have evaluated the capacity of hiPSC-derived cardiomyocytes for remuscularization of the injured heart, recent preclinical studies have been examined using the porcine model . In these studies, hiPSC-derived cardiomyocytes, endothelial cells, and smooth muscle cells were delivered using a fibrin patch following cardiac injury in the porcine model [28]. These hiPSC-derived cells survived and improved heart function without inducing any ventricular arrhythmias. Collectively, these results support the notion that hiPSC-derived cell populations have a tremendous potential as a cell source for cardiac repair and regeneration.
Bone Marrow Mononuclear Cells and CD34+ Cells
Bone marrow transplants are performed worldwide and have been shown to be a proven therapy for terminal diseases. Initially, the bone marrow donor cell population was harvested directly as a bone marrow aspirate and characterized based on the quantification of CD34+ cell populations . CD34 is a transmembrane sialomucin protein that has a functional role in cell-cell adhesion and cellular migration [29]. It is expressed on long-term hematopoietic stem cells in humans. Numerous studies have demonstrated the capacity of bone marrow mononuclear cells to participate in cardiac repair following injury [30, 31], and an equal number have demonstrated that this cell population does not participate in cardiac regeneration following injury (◘ Fig. 24.1) [32–34]. These controversies are ongoing, but the consensus at this time is that the bone marrow mononuclear cells are less effective in assuming a cardiac fate and regenerating injured myocardium.
Mesenchymal Stem Cells (MSCs )
MSCs have several unique features that make them attractive candidates for cell transplantation. First, they are easily accessible and expandable, as they could serve as an “off the shelf” allogeneic product, which would be cost-effective and available at the time of urgent interventions. Importantly, these cells appear to be immunoprivileged as they lack MHC II and B-7 costimulatory molecule expression and therefore have limited T-cell responses [35–39]. Yet, they are considered to directly inhibit inflammatory responses via paracrine mechanisms including the production of transforming growth factor beta-1 and hepatocyte growth factor [40, 41]. Previous studies using small animal (i.e., rodents) and large animal models (i.e., porcine) have shown short-term beneficial effects following the delivery of MSCs into the post-injured heart. These MSC-mediated effects include increased vasculogenesis, decreased scar burden, reverse remodeling, and improved cardiac function [42–44]. Again, controversial results have confronted this field as studies undertaken in Robert Kloner’s laboratory, using a rat model of post-infarction LV remodeling, found that the beneficial effects on left ventricular function were short term and were absent after 6 months post-MSC delivery [45].
c-Kit-Expressing Cells
Beltrami et al. isolated cells expressing the tyrosine kinase receptor for stem cell factor (also referred to as steel factor or c-kit or CD117) from the interstitium of the adult rat heart [46]. The highest density of these lineage negative (lin−), c-kit+ stem cells was in the atria and ventricular apex. These cells were characterized as self-renewing, clonogenic, and multipotent. Further, they had the ability to differentiate into multilineages, including cardiomyocytes, endothelial cells, and smooth muscle cells. Moreover, the delivery of these c-kit-expressing clonogenic stem cells following myocardial injury improved left ventricular function and supported the notion that they contributed to myocardial regeneration. Recently, the same laboratory expanded their analyses to include preclinical studies using large animal models. They have demonstrated that the canine model also harbors a c-kit-expressing stem cell population in the adult heart that is clonogenic, multipotent, and capable of activation following injury. In response to myocardial injury, these c-kit-expressing stem cells were shown to be activated by cytokines and home to areas of injury to participate in repair and regeneration [47]. These preclinical studies have been extended to the study of the human heart. A similar c-kit-expressing stem cell population (c-kit-positive but negative for the expression of the hematopoietic and endothelial antigens including CD45, CD31, and CD34) has been isolated from the adult human heart and has been shown to be multipotent (capable of forming myocyte, smooth muscle cell, and endothelial cell lineages) in vivo and in vitro [48]. Moreover, studies have established that these human c-kit-expressing cardiac stem cells undergo both symmetrical and asymmetrical cell divisions [48]. Importantly, these results have generated intense interest and are being validated by other cardiac stem cell laboratories. For example, van Berlo et al. utilized genetic labeling strategies and demonstrated that c-kit-expressing stem cells were able to daughter cardiomyocytes, yet this was a rare event [49]. These investigators demonstrated that c-kit-expressing stem/progenitors primarily daughtered the endothelial cell population (as opposed to the cardiomyocyte lineage) within the adult mouse heart. These studies underscore the ongoing controversies associated with the stem cell/progenitor cell field.
Myogenic Stem Cells
The adult skeletal muscle has a resident stem cell population that is capable of complete regeneration and restoration of the myogenic architecture following an injury that destroys up to 90 % of the skeletal muscle [50]. This resident stem cell population is also referred to as the satellite cell pool; these stem cells are located in close approximation to the multinucleated myofiber. These satellite cells are sandwiched beneath the basal lamina and the plasmalemma (which expresses dystrophin) and constitute 2–5 % of all nuclei (in the skeletal muscle) [50]. Satellite cells express the transcription factor Pax7 as well as other markers including c-met, CD34, VCAM, α7-integrin, syndecan 3/4, and other proteins. Satellite cells are capable of tremendous cellular proliferation and self-renewal and can assume both a quiescent and activated state. Activation of these satellite cells is mediated by an Fgf-CK2-mSds3-Foxk1-Foxo/Sox/Tgfb-p21 network [50, 51]. Studies have demonstrated that the delivery of a single isolated myofiber which contains as few as seven myogenic stem cells was capable of muscle regeneration and generated 100 new myofibers containing thousands of myonuclei [52]. These animal studies provided a platform for human clinical studies using human myoblasts for cardiovascular disease (◘ Fig. 24.1).
Cardiospheres
In 2004, Messina et al. isolated undifferentiated cells that proliferated as self-adherent clusters (termed cardiospheres) from subcultures of postnatal atrial or ventricular human biopsy specimens and also from murine hearts [53]. These cardiospheres varied in size (20–150 μm) and were observed to beat spontaneously in culture. The cardiosphere-forming cells had the properties of adult cardiac stem cells as they were clonogenic, they expressed stem and endothelial progenitor cell antigens/markers (c-kit, Sca-1, CD31, and Flk-1), they were capable of long-term self-renewal, and they could differentiate in vitro and in vivo into myocytes and endothelial cells [53]. Importantly, these cardiospheres represented a heterogeneous cell population that was expanded in vitro. The expansion of the cardiosphere-forming cells resulted in more than 1 million human cardiospheres within a several-month period. These studies provided a platform for further studies undertaken in the Marban laboratory [54]. Cardiospheres and cardiosphere-derived cells express antigenic characteristics of stem cells at each stage of processing, as well as proteins vital for cardiac contractile and electrical function [54]. Human and porcine cardiosphere-derived cells cocultured with neonatal rat ventricular myocytes exhibited biophysical signatures characteristic of myocytes, including calcium transients synchronous with those of neighboring myocytes [54]. Moreover, the delivery of cardiosphere-derived cells following myocardial injury resulted in improved myocardial function compared to their respective controls.
Sca-1 and Side Population (SP) Cells
Resident murine cardiac progenitors have also been isolated on the basis of stem cell antigen-1 (Sca-1) expression [55]. These Sca-1-expressing CPCs were small interstitial cells that lacked hematopoietic lineage markers such as CD45, B220, TER119, or Flk-1, and they lacked c-kit expression, supporting the notion that they were distinct from the c-kit stem cell population . Studies verified that the Sca-1-expressing CPC population expressed the vascular marker CD31 and the cardiogenic transcription factors including GATA4, MEF2C, and TEF-1 (but lacked expression of NKX2-5). A subpopulation of these Sca-1-expressing CPCs activated cardiac genes, but did not exhibit spontaneous contractile properties in response to DNA demethylation with 5-azacytidine [55]. Studies were further undertaken to examine the capacity of the Sca-1 CPCs to form cardiomyocytes independent of fusion to the differentiated host cardiomyocytes, using genetic mouse models (Cre/Lox and the R26R genetic mouse models) for cellular labeling. Genetically labeled Sca-1 CPCs isolated from the αMHC-Cre transgenic mouse model and delivered into injured hearts of R26R (resulting in the labeling of all host cells with LacZ) mice. Two weeks following injury, the animals were sacrificed, and the hearts were examined for Cre and LacZ expression. Interestingly, approximately half of the cells expressing αMHC-Cre did not express LacZ, suggesting that the Sca-1-expressing cells were capable of myocardial differentiation independent of fusion to existing (host) cardiomyocytes [55]. Additional studies from other laboratories (Matsura and coworkers) have also isolated Sca-1+ cells from adult murine hearts and demonstrated that they were capable of differentiation into beating cardiomyocytes in the presence of oxytocin but not 5-azacytidine [56]. These Sca-1 CPCs were heterogeneous, but a subpopulation was capable of effluxing Hoechst 33342 dye. Due to their ability to efflux Hoechst 33342 dye, these cells were described as a side population using flow cytometry and were termed SP cells. Subsequently, these SP cells have been isolated from a number of lineages including adult bone marrow, skeletal muscle, the lung, and mouse ESCs. These respective SP cell populations were multipotent when placed in a permissive environment. The ability of the SP cells to efflux the Hoechst dye was due to the presence of multidrug resistance (MDR) proteins . Studies have demonstrated that Abcg2 was a member of the ATP-binding cassette (ABC) transporters (also known as multidrug resistance proteins) and was the molecular determinant for the SP cell phenotype [57]. Both specific (FTC) and nonspecific (calcium channel blockers such as verapamil) blockers of Abcg2 prevented Hoechst 33342 dye exclusion. Abcg2-expressing SP cells participate in cardiac development and reside in the adult mouse heart [57]. Following injury, these Abcg2-expressing cardiac SP cells increased in number and formed fetal cardiac-like cells. In addition to serving as a marker for the SP cell population, Abcg2 has a cytoprotective function in response to oxidative stress. Moreover, previous studies have demonstrated that HIF2α is a direct upstream regulator of the Abcg2 gene [58]. These results support the notion that CPCs in the adult heart likely have a cytoprotective mechanism that promotes survival following injury. To date, whole-genome analyses using microarray platforms have examined the molecular signature of adult cardiac SP cells, adult bone marrow SP cells, adult skeletal muscle SP cells, and SP cells isolated from ESCs [59]. As expected, cardiac SP cells express Abcg2, Sca-1, and c-kit. They also have induction of signaling pathways including the Notch signaling pathway and the Wnt signaling pathway, which are characteristics of a number of other stem cell populations [59]. Yet, the cardiac SP cells largely lacked expression of hematopoietic markers (CD45 and TER119). Other laboratories have also isolated the SP cells from mouse hearts based on their ability to exclude Hoechst 33342 dye and have shown they differentiate into cardiomyocytes after coculture with rat cardiomyocytes [57, 60].
Stem Cell and Progenitor Cell Consortium for Cardiovascular Disease
To establish an interactive and collaborative network for the acceleration of discoveries, the National Heart, Lung, and Blood Institute at the National Institutes of Health established the NHLBI Progenitor Cell Biology Consortium [61]. One of the goals of this consortium was to gain an understanding of the mechanisms that direct stem and progenitor cells to a cardiac fate. Importantly, this network provided an infrastructure for the field and addressed issues including but not limited to:
- 1.
The definition of a hierarchy of somatic stem and progenitor cells that reside in the adult heart
- 2.
The definition of transcriptional networks, epigenetic networks, and microRNA networks that direct stem cells and progenitors toward a cardiac fate
- 3.
Provided protocols for stem/progenitor cellular characterization and cardiomyocyte differentiation pathways
- 4.
Established strategies to reprogram somatic cells (i.e., fibroblasts) to cardiomyocytes
- 5.
Established fate-mapping strategies to define the contribution of selected stem/progenitor cell populations to the cardiac lineage during development and following myocardial injury
- 6.
Compared specific cardiac and hematopoietic stem/progenitor cells using FACS, transcriptional, microRNA, functional, or epigenetic analyses
SCT studies have variable results. More than 2000 patients have received SCT for cardiovascular disease, and studies support the notion that the safety profile for SCT is favorable. However, while the first study using SCT for cardiovascular disease was performed more than 10 years ago, there has been a variable and, at best, only a modest benefit from such therapy. These initial studies utilized the delivery of skeletal myoblasts at the time of surgical revascularization [62]. These skeletal myoblasts survived the delivery into the injured heart, and they differentiated to form skeletal muscle in the cardiac milieu. Unfortunately, the newly formed skeletal muscle was not electrically synchronized with the host cardiomyocytes, and therefore there were a number of arrhythmogenic events requiring implantable cardioverter defibrillator (ICD) support. Therefore, due to the lack of integration and the inability of skeletal myoblasts to transdifferentiate to form cardiomyocytes, SCT using skeletal myoblasts has received less interest.
Due to the remarkable success of bone marrow transplantation for the treatment of hematopoietic diseases, studies were undertaken to examine the safety and effectiveness of unfractionated bone marrow mononuclear cells to transdifferentiate to a cardiomyocyte fate. One of the first randomized clinical studies compared mobilized stem cells with bone marrow mononuclear cells delivered 5 days following percutaneous coronary intervention [63]. One year following SCT, there was a modest decrease in infarct area in patients receiving either cell population. A comparable modest improvement in cardiac function was observed in the Reinfusion of Enriched Progenitor cells and Infarct Remodeling in Acute Myocardial Infarction (REPAIR-AMI) study which examined 204 patients with bone marrow mononuclear SCT following percutaneous coronary intervention (PCI) at a 1-year follow-up period compared to control patients [64]. The Bone Marrow Transfer to Enhance ST-Elevation Infarct Regeneration (BOOST) trial delivered bone marrow mononuclear cells (intracoronary route) 5 days following PCI and observed no significant difference in LV function between experimental vs. control patients [65]. Similarly, no significant improvement in cardiac function was observed in the Autologous Stem cell Transplantation in Acute Myocardial Infarction (ASTAMI) study (n = 100) following intracoronary delivery of bone marrow mononuclear SCT 6 days following PCI at a 3-year follow-up [66]. Moreover, little functional improvement was demonstrated in the NHLBI-supported Cardiovascular Cell Therapy Research Network (CCTRN) studies (TIME, LATE TIME, and FOCUS) which examined the use of SCT (bone marrow mononuclear cells) for ischemic cardiovascular disease [32–34]. In contrast to these SCT studies using bone marrow mononuclear cells , Haddad et al. demonstrated that intracoronary delivery of CD34+ cells in patients with DCM has an average increase of LVEF of 5 % over a 5-year follow-up period [67]. Overall, while selected studies have demonstrated a modest improvement, it is generally agreed that many of the bone marrow-derived cell populations have not resulted in remuscularization of the heart. These data further emphasize the limited plasticity of somatic cell populations.
Another bone marrow-derived stem cell population is the mesenchymal stem cell (MSCs) (CD73+, CD90+, CD105+, CD34−, CD45−, HLA-DR−, CD14−, CD11b−, CD79a−, CD19−) population. While MSCs are believed to be immunoprivileged and have increased plasticity (able to daughter endothelial cells, osteoblasts, chondrocytes, adipocytes, etc.), a limited number of clinical studies have utilized this cell population for acute ischemic cardiomyopathy [68, 69]. In a small, Phase I study (n = 53), patients were randomized to receive placebo or allogeneic MSCs following an acute injury (i.e., MI) which resulted in a modest cardiac functional improvement at 6 months following delivery [70]. Moreover, adipose-derived MSCs were delivered (intracoronary) in 14 patients following ST segment elevation MI (APOLLO study) resulting in a reduction of the scar burden and modest cardiac functional improvement [71]. In contrast to the pilot studies using MSCs for acute ischemia, more than six studies are underway or have been published using this cell population for chronic ischemic dilated cardiomyopathy . Several of these studies from the Hare laboratory (PROMETHEUS and POSEIDON studies) have demonstrated beneficial effects [72, 73]. In the POSEIDON study, which utilized autologous and allogeneic MSCs, there were decreased scar burden and ventricular volumes in both groups [72]. In the PROMETHEUS study, autologous MSCs were delivered at the time of surgical revascularization (i.e., CABG), which resulted in decreased scar tissue with increased perfusion, wall thickness, and systolic strain at 18 months following delivery [73]. Currently, Phase III clinical studies are in progress, which will enroll more than 1500 patients with chronic heart failure to receive intramyocardial delivery of MSCs.
Similarly, limited functional improvement was observed in the CADUCEUS study that examined SCT (intracoronary delivery of autologous cardiospheres) for ischemic CHF [74]. While only modest functional changes were observed, reduced scar burden and reversed structural remodeling of the injured human heart were noted. In addition, more recent studies have focused on the cardiosphere-mediated release of exosomes . These exosomes (cellular vesicles which have a diameter of approximately 50 nm) have been postulated to contain growth factors, mRNAs, miRs, double-stranded DNA, and antifibrotic factors that may promote structural changes of the injured heart (◘ Fig. 24.2) [53]. Alternatively, these exosomes may also harbor factors that stimulate the endogenous cardiac progenitors to participate in new vessel and remuscularization of the heart following cardiosphere delivery (◘ Fig. 24.2). Similarly, the SCIPIO study delivered c-kit+(lin−) cardiac stem cells in 33 patients, and the CELLWAVE study (bone marrow mononuclear cells; n = 103) demonstrated improved LVEF in patients with ischemic heart disease [31, 74–77].


Fig. 24.2
Somatic cell therapy has a number of possible roles following the delivery into an organ of interest. Schematic highlighting the possible impact of SCT other than remuscularization
Alternative cell sources include the use of hESC and hiPSC lines (◘ Fig. 24.1). Previous studies have demonstrated the use of hESC-derived retinal support cells delivered into the eyes of patients with macular degeneration, which appeared to be safe and have encouraging results [78]. While hESC- or hiPSC-derived cardiomyocytes have not been utilized in clinical studies for patients with heart failure, nonhuman primate studies have shown that the delivery of a large number of hESC-derived cardiomyocytes results in remuscularization of the injured heart but has associated arrhythmogenic events [18, 79]. These hESC and hiPSC sources continue to lag behind others but may have increased potential for remuscularization of the injured heart compared to other cell sources.
Collectively, the variable results of SCT for cardiovascular disease may be multifactorial. These varied results may represent differences in the selection of cell source (i.e., CD34+ cells, bone marrow mononuclear cells, mesenchymal stem cells, c-kit-/lin−-expressing cells, cardiospheres, umbilical cord blood stem cells, skeletal myoblasts, etc.), cell processing and storage, mode of delivery [i.e., intracoronary, intramyocardial (endocardial vs. epicardial delivery), venous (coronary sinus), arterial, etc.], endpoints (3 months to 5 years), timing of delivery and disease etiology (i.e., delivery during the acute post-MI period, chronic ischemic coronary artery disease, nonischemic DCM, etc.), patient selection (age of patient, gender of patient, presence of comorbidities such as diabetes mellitus or tobacco use, and biomarker status), and cell source (autologous vs. allogeneic sources) (◘ Fig. 24.3). These pilot studies with limited patient numbers and one or more of the above variables may be important contributors to the uneven response of SCT for cardiovascular disease. To further emphasize the challenges facing SCT, we will further highlight issues pertaining to the proposed mechanisms, the mode of cell delivery, and the ideal cell population for SCT and cardiovascular disease.


Fig. 24.3
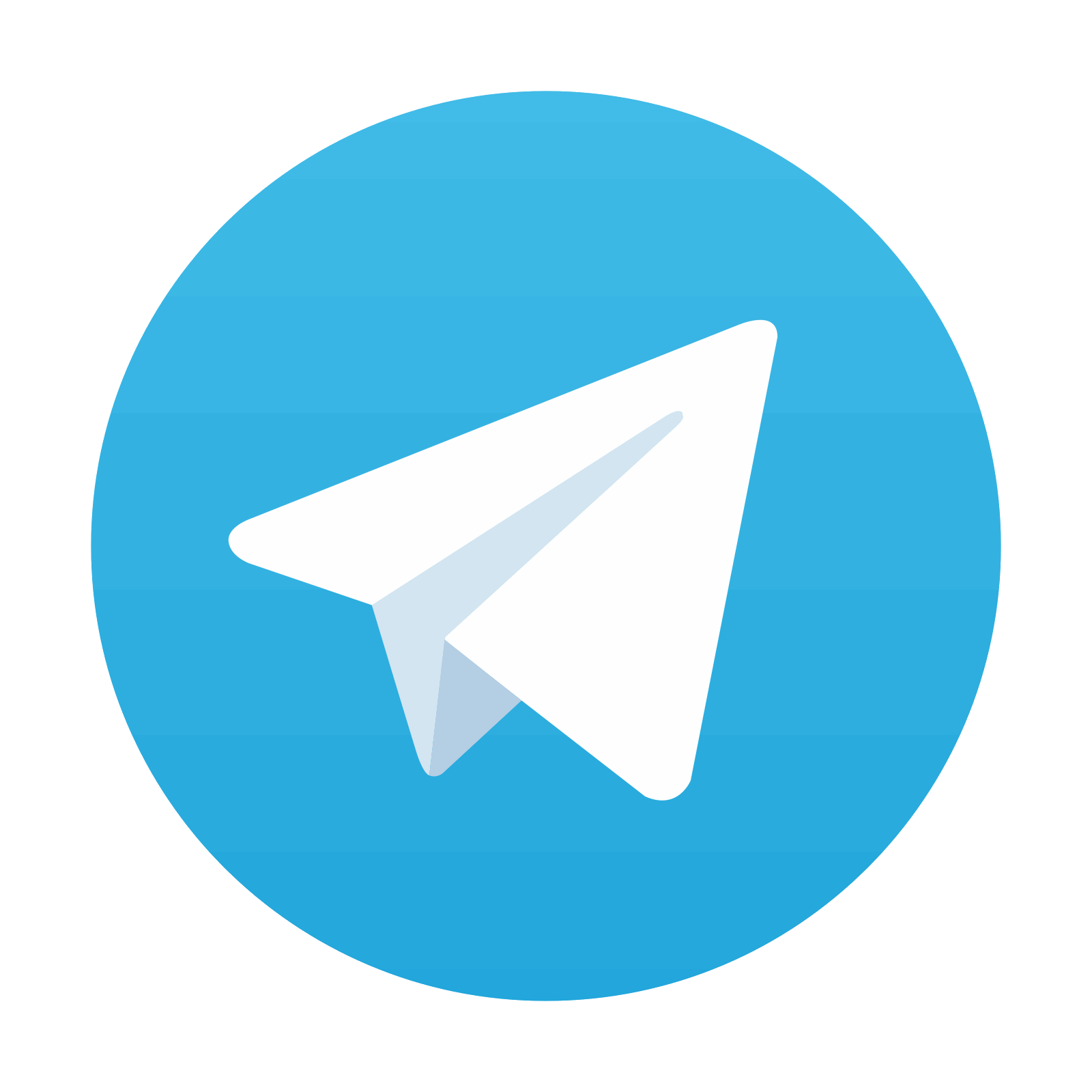
Somatic cell therapy may mobilize endogenous cardiac progenitors . A proposed role for SCT is that the exogenous cells may mobilize endogenous cardiac progenitors to the area of myocardial injury and repress fibrosis/scar or apoptosis (infarct extension) or promote neovascularization or remuscularization
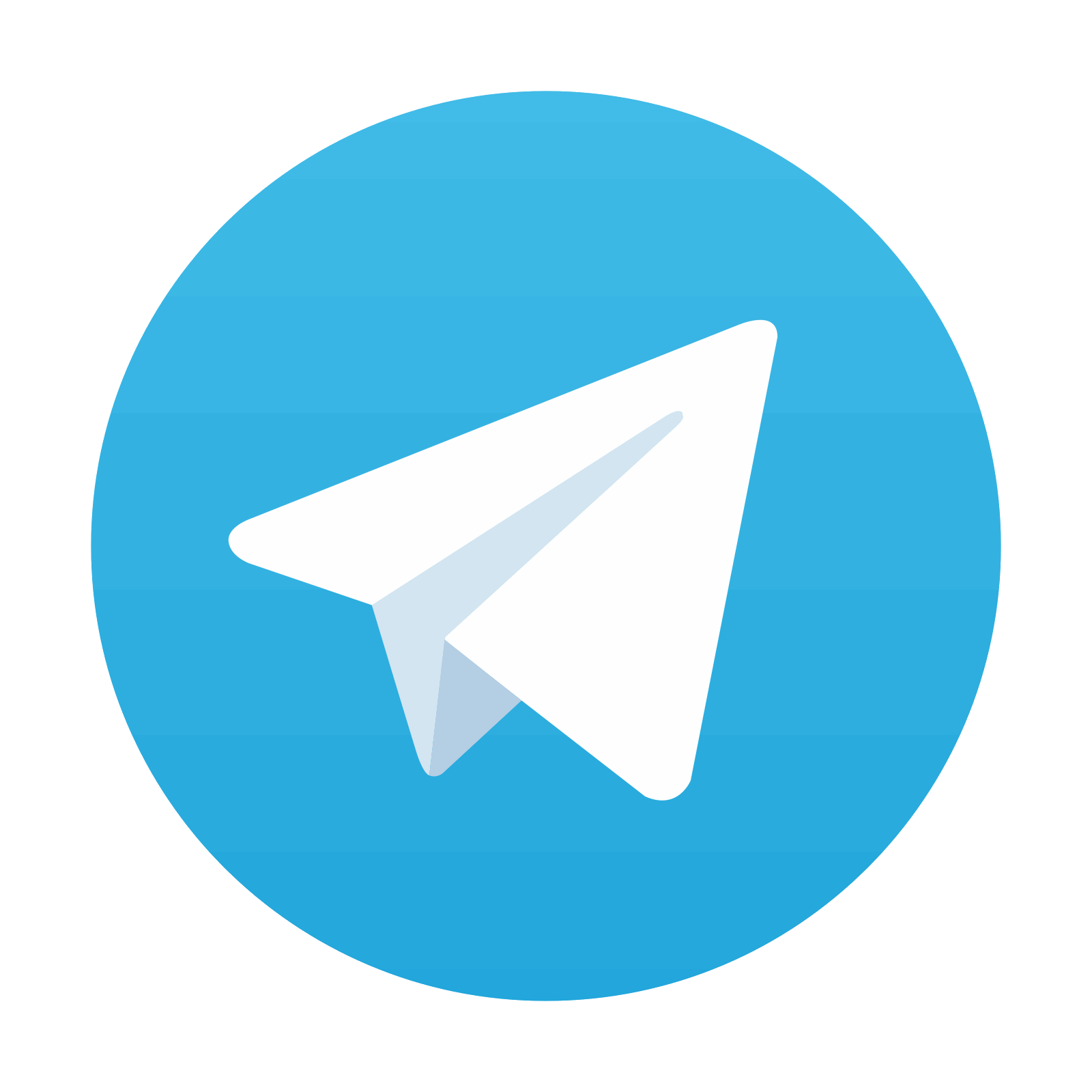
Stay updated, free articles. Join our Telegram channel

Full access? Get Clinical Tree
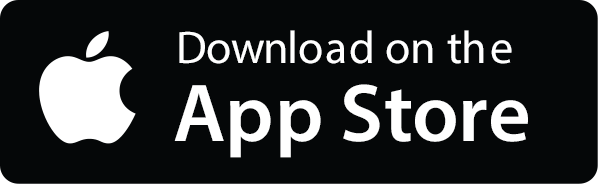
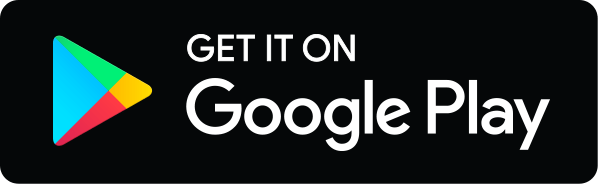
