Catheterization Hemodynamics and Formulae
Grant W. Reed
Amar Krishnaswamy
I. INTRODUCTION. Knowledge of the hemodynamic pressure waveforms and formulae used to diagnose valvular pathology can be a valuable tool in the management of valvular heart disease. As advances in cardiovascular imaging have decreased the need for routine invasive hemodynamic assessment, catheterization is typically reserved for patients with symptoms that are out of proportion to the severity of valvular pathology based on noninvasive testing. Additional patients that may benefit include those requiring precise assessment of valve gradients, valve areas, cardiac output (CO), or intracardiac pressures. Knowledge of normal hemodynamics is essential to interpret the hemodynamic tracings for common valvular pathologies, as discussed later.
II. NORMAL INVASIVE HEMODYNAMICS
A. Right heart catheterization. Right-sided hemodynamic pressure waveforms are obtained from right heart catheterization (RHC). During RHC, a balloon-tipped catheter (typically a 7 or 8 French Swan-Ganz) is inserted percutaneously through a venous sheath, most often placed in the internal jugular or common femoral veins; the subclavian vein is sometimes utilized, and in patients undergoing coronary angiography via the wrist a 5 French brachial vein Swan-Ganz may be floated. The catheter is passed sequentially through the central veins to the right atrium (RA), right ventricle (RV), and into one of the two main pulmonary arteries (PAs).
When the catheter tip is in a mid/distal PA branch, the inflated balloon serves to block anterograde flow and allows for an indirect measurement of pressure in the left atrium (LA), otherwise known as the pulmonary capillary wedge pressure (PCWP). In the absence of pulmonary vein stenosis, PCWP is a reasonable surrogate for LA pressure, which approximates left ventricular (LV) diastolic pressure in the absence of mitral stenosis (MS). Conversely, LA pressure can be directly measured via a transseptal puncture across the RA to the LA if the PCWP appears inaccurate in a given patient or more precise measurement of the LA pressure is necessary.
Aortic and LV hemodynamics are measured via left heart catheterization (LHC). This is usually accomplished with a pigtail catheter advanced to the aortic root and LV via a sheath in the radial artery or common femoral artery.
With these procedures, hemodynamic waveforms and oxygen saturations can be obtained in each of the cardiac chambers. This allows for the diagnosis of valvular disease, assessment of CO, intracardiac shunting, constrictive and restrictive cardiomopathy, and many other conditions. Full descriptions of the RHC and LHC procedures are beyond the scope of this chapter, though details on calculation of CO are discussed further later.
1. Right atrial pressure.
a. An understanding of the normal pressure waveforms in each cardiac chamber is necessary for the proper interpretation of how these hemodynamic tracings change with valve disease. Normal pressure waveforms for each cardiac chamber,
PCW, and pulmonary artery are displayed in Figure 20.1, and normal values are provided in Table 20.1.
PCW, and pulmonary artery are displayed in Figure 20.1, and normal values are provided in Table 20.1.
b. Normal RA mean pressure is 2 to 6 mm Hg and is characterized by “a-,” “c-,” and “v-” waves, with “x-” and “y”-descents (Fig. 20.1). The a-wave represents pressure rise due to atrial contraction and follows the P-wave on electrocardiogram (ECG). The x-descent follows and represents atrial relaxation and sudden downward motion of the atrioventricular junction due to ventricular systole. The c-wave is next, which represents a subtle pressure rise due to closure of the tricuspid valve (TV) and motion toward the RA during ventricular systole. After the c-wave, the x-descent continues (and if the c-wave is present, it is termed the x′-descent). The final event is the v-wave, which represents passive venous filling during ventricular systole and corresponds to the T-wave on ECG.
c. During inspiration, intrathoracic pressure decreases, which increases venous return and right-sided filling; thus, the v-waves and y-descents become more
prominent. Additionally, while normally a-wave amplitude exceeds v-wave amplitude, certain pathologic conditions such as tricuspid regurgitation (TR) are associated with large v-waves. Finally, during atrial fibrillation, a-waves will not be seen.
prominent. Additionally, while normally a-wave amplitude exceeds v-wave amplitude, certain pathologic conditions such as tricuspid regurgitation (TR) are associated with large v-waves. Finally, during atrial fibrillation, a-waves will not be seen.
TABLE 20.1 Normal Hemodynamic Parameters | ||||||||||||||||||||||
---|---|---|---|---|---|---|---|---|---|---|---|---|---|---|---|---|---|---|---|---|---|---|
|
2. Right ventricular pressure. The normal RV pressure is 20 to 30 systolic/2 to 8 diastolic mm Hg, with a rapid rise due to ventricular contraction during systole, and rapid decline with ventricular relaxation during diastole (Fig. 20.1). The diastolic pressure gradually rises due to RV filling during diastole. RA mean pressure should be within a few mm Hg of RV end-diastolic pressure (RVEDP), except in the presence of tricuspid stenosis (TS), in which case RA mean > RVEDP. Unlike the RA, there is not usually an a-wave seen on the RV tracing. If there is an a-wave, it will be seen just before RVEDP and is only seen in states of decreased RV compliance, such as volume overload, RV hypertrophy, or pulmonary hypertension.
3. Pulmonary artery pressure
a. Under normal circumstances, PA pressure is 20 to 30 systolic/4 to 14 diastolic mm Hg. Similar to other arterial waveforms, the PA tracing has a rapid rise, well-defined peak, a dicrotic notch (from pulmonic valve [PV] closure), and a well-defined nadir in diastole (Fig. 20.1). PA systolic pressure (PASP) should approximate RV systolic pressure (RVSP), unless there is pulmonic stenosis (PS), in which case RVSP > PASP. Furthermore, while PASP and RVSP are usually similar in magnitude, there is individual variability and these values may differ under normal conditions in certain patients.
b. Comparing the RV and PA tracings, important identifying features are the approximately 5 mm Hg increase in diastolic pressure from the RV to the PA, and the appearance of the dicrotic notch in the PA. Also, the events of the PA waveform are slightly delayed with regard to the ECG, with the PASP peaking within the T-wave on ECG.
4. Pulmonary capillary wedge pressure
a. The PCWP has a normal range of 4 to 14 mm Hg, and a-, c-, and v-waves similar to an atrial waveform (as it approximates LA hemodynamics) (Fig. 20.1). Unlike an atrial waveform, there is a delay in the pressure transmission from the LA across the pulmonary veins and pulmonary capillary bed. This delay usually places the a-wave after the QRS and v-wave after the T-wave on ECG. Additionally, unlike the RA tracing, the c-wave is not visible on the PCW tracing due to pressure dampening, and the v-wave typically exceeds the a-wave in amplitude.
b. The PCWP is typically reported as a mean with a normal value approximately 0 to 5 mm Hg lower than PA diastolic pressure, unless there is elevated pulmonary vascular resistance. Changes in thoracic pressure during the respiratory cycle alter the PCWP tracing baseline, and mean PCWP is typically measured at end expiration (corresponding to the “peaks” in normal patients, and “valleys” in patients intubated undergoing mechanical ventilation). It may be helpful in awake patients with substantial respiratory variation in pressure to simply hold their breath, but they should be advised not to take a deep breath (or exhale) before this hold.
c. While under ideal circumstances, PCWP approximates LA pressure (which approximates LVEDP), this rests on several assumptions, including no impedance of flow distally. PCWP will not correlate with LA pressure in the presence of pulmonary vein stenosis, and LA pressure will be a poor surrogate for LVEDP in patients with MS, severe mitral regurgitation (MR), severe AI, or poor LV compliance. Additionally, the presence of positive end-expiratory ventilation and improper RHC placement will decrease the reliability of the PCW tracing.
5. Left ventricular pressure. Normal LV pressure is 90 to 140 systolic/10 to 16 diastolic mm Hg, and similar to the RV waveform is characterized by a rapid
upstroke and rapid decline. Diastolic pressure slowly rises during diastole to LVEDP, which is measured at end expiration just before the rapid upstroke during systole. Similar to the RV tracing, an a-wave is not usually seen except under circumstances of LV noncompliance. Simultaneous LV, arterial pressure, and PCWP are shown in Figure 20.2.
upstroke and rapid decline. Diastolic pressure slowly rises during diastole to LVEDP, which is measured at end expiration just before the rapid upstroke during systole. Similar to the RV tracing, an a-wave is not usually seen except under circumstances of LV noncompliance. Simultaneous LV, arterial pressure, and PCWP are shown in Figure 20.2.
![]() FIGURE 20.2 Simultaneous radial artery, left ventricular, and pulmonary capillary wedge pressure tracings. |
6. Aortic pressure
a. Central aortic pressure is typically measured in the aortic root or ascending aorta, and normally measures 90 to 140 systolic/60 to 90 diastolic mm Hg. Similar to other arterial tracings, there is a rapid upstroke to a well-defined peak and gradual decline that is interrupted by a dicrotic notch, which is caused by closure of the aortic valve (AV).
b. Aortic systolic pressure should equal LV systolic pressure in the absence of obstruction within the LV, at the level of the AV (i.e., aortic stenosis [AS]), or proximal aorta (i.e., supra-aortic membrane). While not normally seen, an “anacrotic” notch may be present during systolic pressure rise in patients with turbulent flow during ejection (i.e., severe AS).
c. Simultaneous LV and aortic pressures are often measured when assessing for AS to determine transvalvular gradient (further discussed later). While femoral (or radial) arterial sheath pressures are sometimes substituted for central aortic pressures, differences between peripheral arterial sheath pressures and central aortic pressures are common. For instance, central aortic pressure may be higher than femoral (or radial) arterial sheath pressure in patients with peripheral arterial disease, sheath kinking, arterial tortuosity, or sheath thrombosis. Conversely, peripheral amplification of reflected arterial pressure waves may cause the peripheral arterial sheath pressure to be greater than central aortic pressure in certain individuals.
B. Measurement of cardiac output. Invasive assessment is the gold standard for determining CO. Normal CO increases in accordance to meet systemic oxygen demand. Thus, any factor that influences systemic oxygen demand influences CO. In the simplest terms, CO is the product of heart rate (HR) and stroke volume (SV):
CO = HR × SV
As CO is greater with body size, CO is typically normalized to body surface area (BSA), resulting in the cardiac index (CI):
BSA (m2) = [check mark]((Ht [cm] × Wt [kg])/3,600)
CI = CO/BSA
Normal values for CO range between 5 and 6 L/min, whereas normal values for CI range between 2.6 and 4.2 L/min/m2. The two major methods for determination of CO in the catheterization laboratory are the Fick technique and thermodilution technique.
1. The Fick technique
a. The Fick equation is the most commonly used method for calculation of CO. The Fick equation is based on the principle that the total uptake (or release) of a substance by a tissue (i.e., lungs) is proportional to the blood flow to the tissue multiplied by arteriovenous (A-V) concentration difference of the substance. Assuming there is no intracardiac shunt, blood flow into the pulmonary circuit should equal blood flow into the LV and systemic circuit, thus:

b. O2 consumption can be measured by subtracting the O2 uptake from room air using a Douglas bag, metabolic hood, or a cardiopulmonary exercise testing machine. Given the limited availability, cost, and time involved in utilizing these methods, most laboratories use an assumed oxygen consumption of 125 mL/min/m2, or 3 mL/min/kg.
c. The A-V O2 saturation difference across the lungs is then obtained by taking the difference between pulmonary venous blood O2 saturation (or systemic arterial O2 saturation, Sao2) and the PA O2 saturation (or “mixed venous” O2 saturation, Svo2). This difference is then multiplied by the O2-carrying capacity of hemoglobin (Hb) to obtain the A-V O2 content difference:
(A-V) O2 content difference = (Sao2 – Svo2) × 1.36 mL/O2/g Hb × g Hb/dL blood × 10
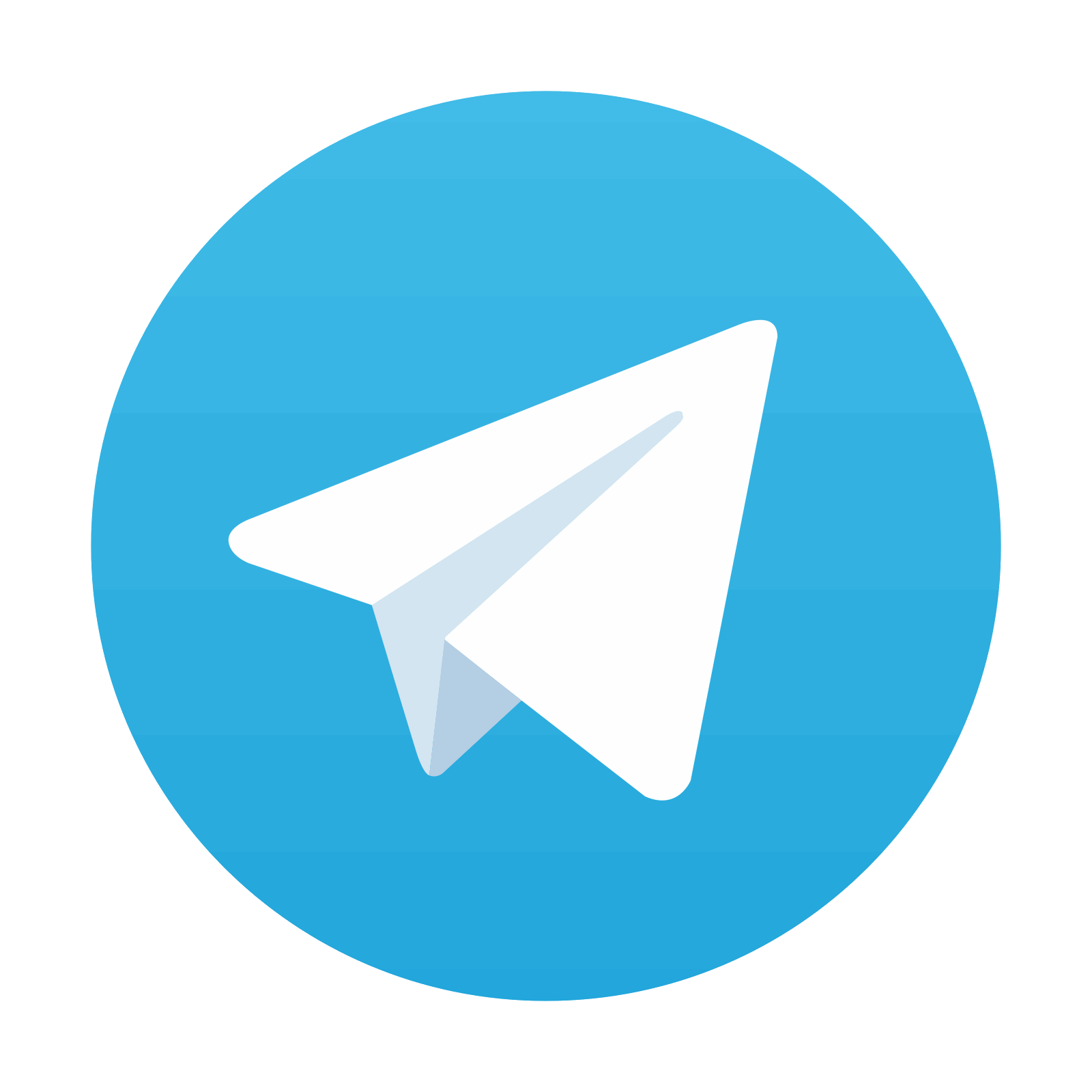
Stay updated, free articles. Join our Telegram channel

Full access? Get Clinical Tree
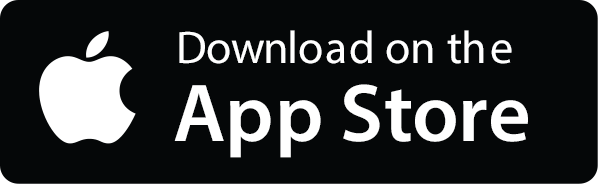
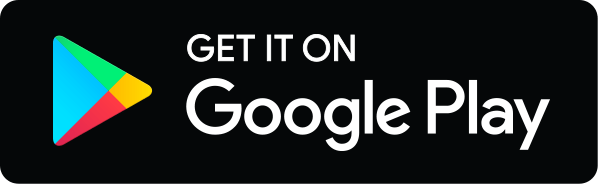