Catheter-Based Radiation
Ron Waksman
Vascular brachytherapy (VBT), using beta and gamma emitters, revolutionized the treatment of percutaneous coronary interventions (PCI), particularly in-stent restenosis (ISR). Preclinical trials were encouraging and facilitated the initiation of the feasibility clinical trials, first in the peripheral arteries, later in coronary arteries through pivotal trials, and then onto commercialization of the technology for clinical use in Europe in 1999. In November 2000, the U.S. Food and Drug Administration (FDA) approved VBT for ISR. Since then, brachytherapy has become an established modality of treatment for ISR, while expanding indications include ISR in saphenous vein grafts (SVG), lower extremities, renal arteries, and de novo lesions in coronary and femoropopliteal vessels.
RADIATION BIOLOGY AND MECHANISM
Radiation inhibits smooth muscle cell (SMC) proliferation and intimal hyperplasia in the irradiated atherosclerotic arteries by intervening in the cell cycle to cause cell death to radiosensitive cells, especially those undergoing mitosis following vascular injury. Cells in the M and G2 phases are the most sensitive to radiation; G1 phase cells are sensitive only in the early G1 phase, and cells in G0 are radio resistant. Radiation effects tissue damage either directly by absorbing into the target molecules, such as DNA, RNA, or enzymes, or indirectly by interacting with these molecules via the formation of highly reactive hydroxyl free radicals. An example of intraarterial radiation following balloon and stent angioplasty is shown in Figure 14.1. Another mechanism of radiation tissue damage is through inducing programmed cell death or apoptosis.
Radiation potentially may reduce restenosis by inhibiting the first wave of cell proliferation in the adventitia and the media and by inducing favorable remodeling (1). Additional mechanisms include the suppression of macrophages and adventitial myofibroblasts (2,3). Cells can repair radiation damage; therefore subtherapeutic doses may only delay the restenosis, because the surviving cells will continue to divide and eventually occupy the lumen wall. Dose optimization is, therefore, important to achieve adequate results. Late effects of VBT may be seen 5 to 10 years after treatment and include late thrombosis, fibrosis, thinning of the media, aneurysm formation, and late restenosis.
RADIATION PHYSICS, DOSIMETRY, AND RADIATION SYSTEMS
Among several isotopes developed for the use in VBT, only three have seen widespread use in clinical application: namely the gamma emitter 192Ir and beta emitters 90Sr/90Y and 32P. The requirements for an ideal radioisotope in VBT include dose distribution of a few mm from the source with minimal dose gradient, low dose levels to the surrounding tissues, treatment time of <10 minutes, and a sufficient half-life for multiple applications when used in a catheter-based system. Gamma- and beta-emitting radionuclides currently investigated and used in the clinical trials for VBT are presented in Table 14.1. The main platforms that deliver radiation are catheter-based systems, such as line source wires, radioactive seeds, radioactive gas, and liquid-filled balloons, or stents utilizing beta or gamma emitters. The latter are no longer used because they created the problem of narrowing at the ends of the stent—the “candy-wrapper effect.”
Source selection depends on the source energy half-life, available activity, penetration and dose distribution, radiation exposure to the patient and the operator, shielding requirements, availability, and cost. Isotope selection and dosimetry for intracoronary brachytherapy are derived from the anatomy of the vessel and the treated lesion, the target tissue (intima, media, or adventitia), the diameter and curvature of the vessel, the eccentricity and composition of the plaque, lesion length, amount of calcium, and the presence or absence of a stent in the treated segment. Dosimetry issues considered include the choice of isotope, beta- versus gamma-emitting radioisotopes, administration of high- versus low-dose rates of radiation, centering versus noncentering of the source in the vessel wall, and the need for stepping. Intravascular ultrasound (IVUS) can assist in treatment planning and dosing, because it determines the actual lumen size and the distances from the source to the lumen and the adventitia. Nevertheless, fixed radial prescription points seem to be easier and more practical, without compromising the outcome.
TABLE 14.1. RADIONUCLIDES FOR VASCULAR BRACHYTHERAPY | ||||||||||||||||||||||||||||||||||||||||||||||||||||||||||||||||||||||||||||||||
---|---|---|---|---|---|---|---|---|---|---|---|---|---|---|---|---|---|---|---|---|---|---|---|---|---|---|---|---|---|---|---|---|---|---|---|---|---|---|---|---|---|---|---|---|---|---|---|---|---|---|---|---|---|---|---|---|---|---|---|---|---|---|---|---|---|---|---|---|---|---|---|---|---|---|---|---|---|---|---|---|
|
192Ir is administered by the CheckMate system (Cordis Corporation, Miami, Florida), which requires manual loading of the radioactive seeds (Fig. 14.2). The AngioRad system (Vascular Therapies, Norwalk, Connecticut) uses a flexible, 30 mm 192Ir wire source. The Galileo system (Guidant Corporation, Irvine, California) administers 32P with automated stepping technology and a centering balloon (Fig. 14.3). The RDX system (Radiance Medical, San Diego, California) also administers 32P; however, the isotope is incorporated directly into the balloon material of the percutaneous transluminal coronary angioplasty (PTCA)-type catheter. 90Sr/Y is administered by BetaCath system (Novoste Corporation, Norcross, Georgia), which employs a hydraulic technique to deliver the radioactive seeds (Fig. 14.4). 188Re is obtained from the 188W/188Re radionuclide generator as a solution, which is injected into the coronary dilatation balloon and can be applied at the target by inflating the balloon.
BRACHYTHERAPY IN ANIMAL MODELS
In a porcine stent model of restenosis, Schwartz et al. applied external radiation therapy (400 to 800 cGy) with an orthovoltage x-ray unit to the coronary vessels following stent placement and showed accentuated development of neointimal hyperplasia (4). In later studies, higher doses of 21 Gy to the entire heart showed inhibition of the neointima formation in the same model. The degree of injury and lack of precise delivery to moving epicardial arteries are potential explanations for these findings. Higher volumes of radiation, although helpful, may result in late fibrosis to the whole heart, and are therefore less attractive compared with the endovascular approaches.
Several groups utilizing catheter-based systems have obtained consistent evidence for the efficacy and safety of radiation using both beta and gamma emitters. Some showed marked reduction with 192Ir in neointimal hyperplasia following balloon injury in both the short term (2 to 4 weeks) and at 6 months (as assessed by histomorphometric studies in the porcine restenosis model), and complete reendothelialization by electron microscopy at 2 weeks in balloon injured and irradiated arteries (dose of 14 Gy) (5). However, at 4 weeks, incomplete reendothelialization was seen after stent implantation with similar doses and normal endothelial vasodilatory response to acetylcholine. The specifics of radiation dose and system have been studied (5, 6, 7, 8, 9, 10).
Verin et al. (11) delivered intra-arterial radiation through 90Y and a centering-balloon catheter in atherosclerotic rabbit iliac and carotid arteries using 6, 12, and 18 Gy and demonstrated a reduction in neointimal cells in all treated groups. At 6 weeks, however, the reduction in neointima was maintained only in the rabbits that received 18 Gy. Waksman et al. (12) demonstrated a reduction in neointima in a dose-dependent manner (7 to 56 Gy) with 90Sr/Y in porcine coronary arteries. A consistent effect of neointimal reduction was seen with doses above 21 Gy, without an increase in adverse effects. Raizner showed a similar effect of reduction of neointima formation, post-balloon and stent injury with another pure beta emitter, 32P, using doses of 32 Gy. In summary, both beta and gamma emitters appear to be equally effective in stented arteries, particularly when radiation is delivered before stent implantation.
UNDERSTANDING GAMMA RADIATION
Gamma rays are photons originating from the center of the nucleus, as opposed to x-rays, which originate from the orbital outside of the nucleus. Gamma rays have energies between 20 keV and 2 MeV and are deeply penetrating; because of this deep penetration, excessive shielding (as compared to beta and x-ray emitters) is needed. The only gamma emitter used for the prevention of restenosis in clinical trials is 192Ir. The efficacy of 192Ir in reducing clinical and angiographic restenosis in patients with in-stent restenosis has been confirmed by a number of studies.
UNDERSTANDING BETA RADIATION
Beta rays are high-energy electrons emitted by nuclei that contain too many or too few neutrons. These negatively charged particles have a wide variety of energies. The half-life of beta emitters ranges from several minutes (62Cu) to up to almost 30 years (90Sr/Y). Beta emitters rapidly lose their energy to the surrounding tissue, and their range is within 1 cm of the tissue. Large-scale clinical studies testing
the effectiveness of beta radiation for de novo, restenotic, and ISR lesions have paralleled the encouraging results of gamma radiation.
the effectiveness of beta radiation for de novo, restenotic, and ISR lesions have paralleled the encouraging results of gamma radiation.
CLINICAL TRIALS WITH VASCULAR BRACHYTHERAPY
The Peripheral System
Liermann and Schopohl (Frankfurt, Germany) were the first to perform VBT for the prevention of restenosis in the peripheral arteries in a pilot study of 30 patients with ISR in their saphenous femoropopliteal arteries (SFAs) (23). Patients underwent atherectomy and PTCA followed by endovascular radiation using the MicroSelectron-HDR afterloader (Nucletron-Odelft, The Netherlands) (Fig. 14.5) and a noncentering catheter with 192Ir. No adverse effects from the radiation treatment were reported up to 7 years follow-up. The 5-year patency rate of the target vessel was 82%, with only 11% stenosis within the treated segment reported. Late total occlusion developed in 7% after 37 months.
In the Vienna trial, 113 patients with de novo or recurrent femoropopliteal lesions were randomized to either PTCA + brachytherapy (n = 57) or to PTCA alone (n = 56). The primary endpoint of cumulative patency rates at 12 months follow-up was higher in the PTCA + brachytherapy group (63.6%) compared to the PTCA group (35.3%) (24). The group from Vienna continued to investigate a series of patients using a higher dose of 18 Gy to improve the outcome of the radiation group. In another series of studies, the effectiveness of radiation as adjunct therapy with primary stenting of the SFA was evaluated in 33 patients. In this series, the recurrence rate at 6 months was 30%; however, this was attributed to a high rate of late thrombosis (nearly 10%) following thrombolysis. Only 12% had ISR.
Late thrombosis was reduced significantly once prolonged antiplatelet therapy was administered, as shown in the Vienna 5 study, in which 90 patients were randomized to stent + radiation versus stent alone for the treatment of SFA lesions.
Late thrombosis was reduced significantly once prolonged antiplatelet therapy was administered, as shown in the Vienna 5 study, in which 90 patients were randomized to stent + radiation versus stent alone for the treatment of SFA lesions.
![]() Figure 14.6. (A) Novoste 40 mm radiation source train: Stepping ×3. (B) Final angiographic result post-radiation. (C) Six-month follow-up angiography. |
Preliminary analysis of the PARIS trial, which includes 203 patients, reported that restenosis was not different at follow-up between the radiated and control groups (28.6% versus 27.5%, respectively). In this trial, a 14 Gy dose was administered using the MicroSelectron HDR afterloader following PTCA to SFA stenosis (25). These data have shown the safety of high-dose radiation to treat femoropopliteal disease, but failed to show improvement in restenosis or functional symptoms when compared with balloon angioplasty (BA) treatment.
THE CORONARY SYSTEM
Gamma and beta radiation were studied for the treatment of in-stent restenosis, whereas only beta sources examined the potential of this therapy in the treatment of de novo lesions.
Gamma Trials
The first study of intracoronary radiation in human coronary arteries was conducted in 1994, by Condado et al., in which 21 patients (22 lesions, with two-thirds being de novo lesions) were treated with 192Ir after routine BA. Serial quantitative coronary angioplasty (QCA) detected a binary restenosis rate of 28.6% at 6 months (26), which remained the same at 5 years. Angiographic complications included four aneurysms (two procedure-related and two occurring within 3 months). At 3 and 5 years, all aneurysms except one remained unchanged, and no other angiographic complications were observed (27).
GAMMA RADIATION FOR IN-STENT RESTENOSIS
The efficacy of 192Ir in reducing clinical and angiographic restenosis in patients with in-stent restenosis (Fig. 14.6A-C) was confirmed by a number of studies, including two single-center trials, Scripps Coronary Radiation to Inhibit Proliferation Post-Stenting (SCRIPPS)
and the Washington Radiation for In-Stent Restenosis Trial (WRIST) series; and multicenter trials GAMMA-1 and -2.
and the Washington Radiation for In-Stent Restenosis Trial (WRIST) series; and multicenter trials GAMMA-1 and -2.
SCRIPPS Trials
SCRIPPS was the first randomized trial to evaluate the safety and efficacy of intracoronary gamma radiation as adjunctive therapy to stents. Fifty-five patients were randomized to either 192Ir therapy (n = 26) or to placebo (n = 29). The angiographic follow-up at 6.8 months and 3 years showed significantly lower restenosis rates in the 192Ir group: 17% and 33%, respectively, compared to placebo (54% and 63%). A subgroup analysis of the 35 patients enrolled due to ISR showed a 70% reduction in the recurrence rate in the irradiated group compared to the placebo (28,29). No evident clinical complications resulted from the radiation treatment, and clinical benefits were maintained at 5 years, with a significant reduction in the need for target lesion revascularization (TLR) (30).
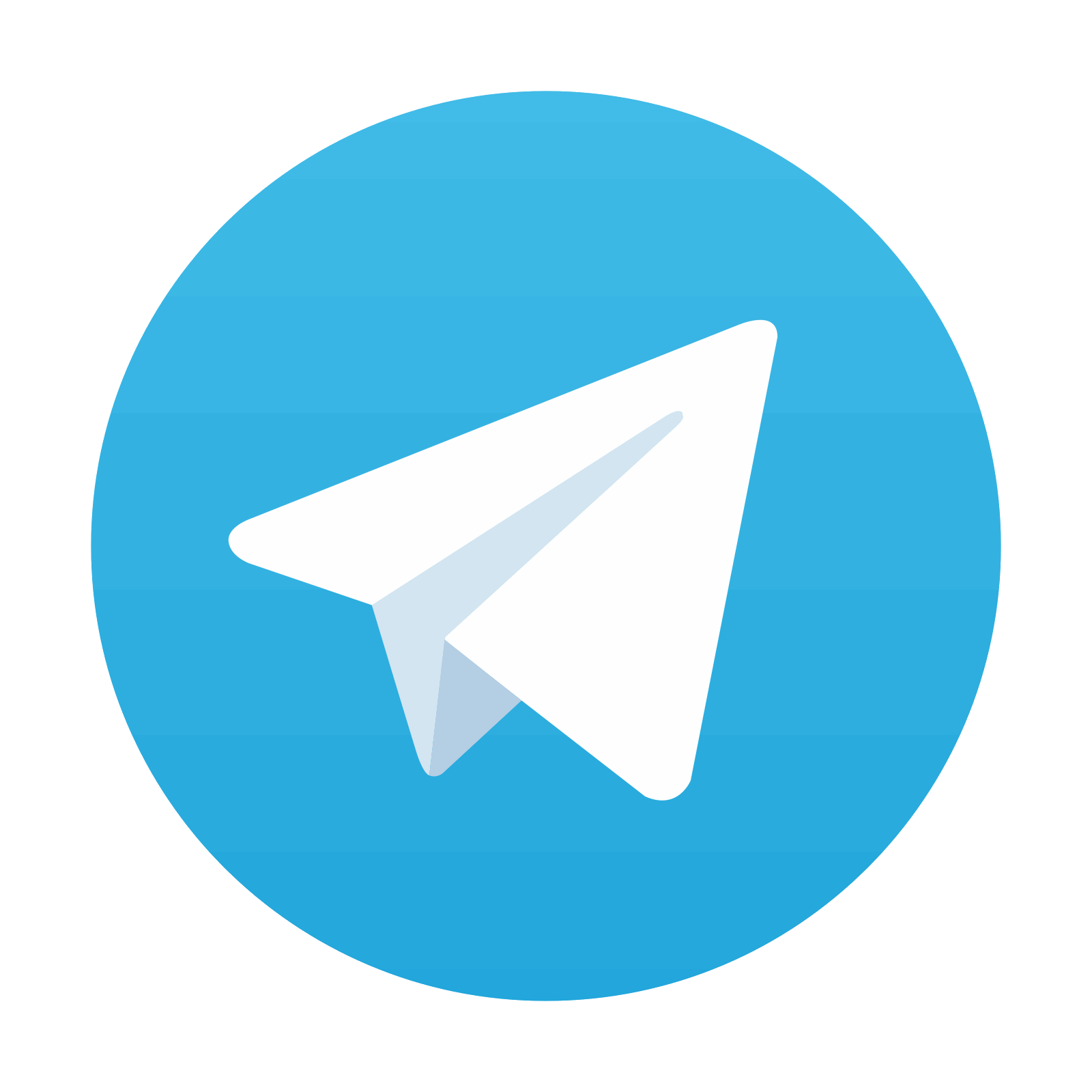
Stay updated, free articles. Join our Telegram channel

Full access? Get Clinical Tree
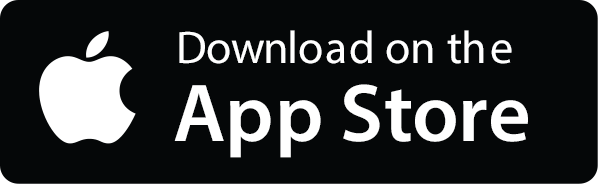
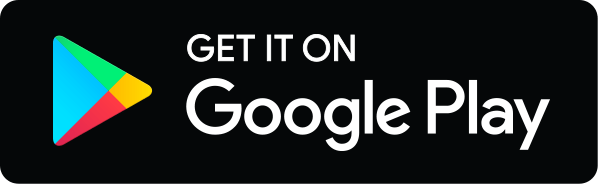