24 Multiple different approaches have been used for catheter ablation of AF.1–8 These involve PV ostial isolation, extending the PV-encircling lesions in the posterior LA to include much of the PV antrum (antrum isolation), adding linear LA lesions (roof line and/or mitral isthmus line), isolation of the SVC, and ablating sites exhibiting fractionated APs in the RA, LA, and CS9–11 during AF (CFAE7). Recent experimental and clinical studies on AF suggest that activation of the intrinsic cardiac autonomic nervous system may plays a significant role in the generation of fractionated AP and the initiation and maintenance of AF.9–21 Autonomic influences on the heart are generated by both the extrinsic (central) and intrinsic cardiac autonomic nervous systems. The extrinsic cardiac autonomic nervous system is comprised of the vagosympathetic system from the brain and spinal cord to the heart. The intrinsic cardiac autonomic nervous system includes autonomic ganglionated plexi (GP) on the epicardial surface of the left and right atria.22,23 The intrinsic system receives the input from the extrinsic system but acts independently to modulate cardiac functions, including automaticity, contractility, and conduction.12,15 The intrinsic cardiac autonomic nervous system contains clusters of autonomic GP located in epicardial fat pads on the left and right atria and in the ligament of Marshall.22,23 The GP contain afferent neurons from the atrial myocardium and from the central autonomic nervous system (extrinsic system), efferent cholinergic and adrenergic neurons (with heavy innervation of the PV myocardium and the atrial myocardium surrounding the GP), and an extensive array of interconnecting neurons.22,23 It has been postulated that AF induced by increased GP activity is produced by triggered firing resulting from early after-depolarizations (EADs).16,17 GP activation includes both parasympathetic and sympathetic stimulation of the atrium surrounding the GP and the PV. Parasympathetic stimulation markedly shortens action potential duration, especially in the PV myocardium (Figures 24.1 and 24.2). Sympathetic stimulation increases calcium loading and calcium release from the sarcoplasmic reticulum (enhancing calcium transient). The combination of short action potential duration (early repolarization) and enhanced calcium release results in high intracellular calcium concentrations during and immediately after repolarization. The high calcium concentration during and immediately after repolarization drives Na+/Ca++ exchange, with 3 Na+ atoms entering the cell for each Ca++ atom exiting the cell, creating a net inward current and producing EADs and triggered firing (“Calcium Transient-Triggered Firing Hypothesis,” Figure 24.1).17 Myocardial contractility, EADs, and triggered firing are enhanced by a pause, especially when the pause follows rapid rate. The rapid rate increases Ca++ loading in the cells (shorter diastolic periods prevent extrusion of all of the Ca++ that enter the cell during systole). The increased Ca++ accumulates in the sarcoplasmic reticulum during the pause. Activation of the cell at the end of the pause results in an exaggerated release of Ca++ from the sarcoplasmic reticulum (increased calcium transient), producing triggered firing (Figure 24.1).17 Figure 24.1 “Calcium Transient Triggered Firing” hypothesis for the mechanism of short episodes of very rapid irregular firing in the pulmonary vein (PV) initiating atrial fibrillation (AF). Action potential is drawn in black and the calcium transient is drawn in red. Ganglionated plexi (GP) activation results in both sympathetic and parasympathetic stimulation. Acetylcholine shortens the action potential duration and norepinephrine enhances the calcium transient. The disparity between the short action potential duration and the enhanced calcium transient can produce early after-depolarization (EAD) with inward sodium–calcium exchange current. Further enhancement of the calcium transient is observed after rapid rhythm followed by pause, initiating triggered firing. (Modified with permission from reference 17.) Figure 24.2 Pulmonary vein triggered firing during high-frequency stimulation (HFS) of the ganglionated plexi (GP) in a canine model. Microelectrode recordings from the left atrium (LA) and left superior pulmonary vein (LSPV) in the isolated canine PV preparation. Left panel: During LA pacing at 50/min without HFS, the action potential duration at 90% of repolarization (APD90) was markedly shorter in the PV myocyte (105 ms) than the LA myocyte (144 ms). Right panel: Short trains of high-frequency stimulation (HFS, CL 10 ms, 0.1 ms pulse width, train duration 300 ms, 100 volts) were delivered to the LA pacing site, immediately after each pacing stimulus (without capture of the LA). HFS produced significant shortening of action potential duration in both the LA and LSPV myocytes, EADs, and triggered firing. At the first triggered beat of each episode, activation of the LSPV precedes the LA activation, indicating the occurrence of triggered firing from the LSPV. The greater APD90 shortening and occurrence of triggered firing in the PV myocyte suggests the PV has greater sensitivity to autonomic stimulation than the LA. (Modified with permission from reference 16.) In a canine isolated LSPV preparation with a rim of adjacent LA, selective electrical stimulation (high- frequency stimulation, HFS: CL 10 ms, 0.1 ms pulse width, train duration 300 ms) of axons originating from the GP produced a striking shortening of action potential duration with EAD and triggered firing in the PV myocardium (Figure 24.2).16,17 The response to HFS was completely blocked by superfusion with tetrodotoxin in low concentration, which blocks neurons without affecting the action potential of the LA or PV myocardium, confirming that HFS produces its effects by stimulating autonomic axons (i.e., GP activity), and not by direct electrical stimulation of the myocardial cells. In this model, atropine prevents the shortening of action potential duration by HFS and prevents triggered firing.17 Atenolol allows the shortening of action potential duration by HFS but prevents the triggered firing. Ryanodine, which prevents the release of Ca++ from the sarcoplasmic reticulum, allows some shortening of the action potential duration, but totally prevents EAD formation and triggered firing.17 The response to ryanodine supports the role of the primary Ca++ release from the sarcoplasmic reticulum in the generation of EADs and triggered firing. The EADs and triggered firing in this preparation are increased by a pause following rapid pacing, similar to the pause-dependant pattern of PV firing seen clinically (Figure 24.3).17 Figure 24.3 Spontaneous pausedependent PV firing from the LIPV in a patient with paroxysmal AF. Tracings from the top are surface ECG leads II, III, V1, and intracardiac EGMs from RAA, HB region, the LIPV, the LA, and the CS. A spontaneous termination of brief AF episode resulted in a sinus pause (1100 ms), followed by very rapid (mean CL < 100 ms), irregular firing in the LIPV, initiating AF. The AF episode terminated again, resulting in another sinus pause (890 ms) and PV firing. PVP, pulmonary vein potential (red arrows). The magnitude of action potential shortening, EAD formation, and triggered firing produced by HFS is greater in the PV myocardium than in the adjacent LA myocardium (Figure 24.2).16 The increased sensitivity of the PV myocardium may explain the clinical observation, in patients with paroxysmal AF, that the focal firing which triggers AF is often located in the PV myocardial sleeves. We have shown that, in an in vivo canine model, epicardial HFS of a fat pad containing GP produces: (1) a vagal response (sinus bradycardia or AV block); (2) marked shortening of the atrial refractory period close to the activated GP; and (3) initiation of sustained AF either spontaneously or by a single atrial extrastimulus delivered close to the GP.10,11,14,15 Testing in the LA distant from the stimulated GP shows little or no decrease in atrial refractory period and sustained AF can not be induced by a single atrial extrastimulus.10,11,15 During AF produced by HFS of GP, rapid and fractionated atrial potentials are consistently located in the adjacent PV and LA surrounding the stimulated GP (Figure 24.4). Intracardiac EGMs recorded at sites distant from the stimulated GP exhibit more organized atrial potentials and longer CLs. These experimental data suggest a relationship between fractionated AP and autonomic activity from GP (Figure 24.4). Figure 24.4 Effects of anterior right GP stimulation on intracardiac EGMs during AF in a canine model. Panel A: Schematic representation of the RA showing position of epicardial recording electrodes (blue electrode catheters) and epicardial pacing electrodes (red cross-hatched areas) over the fat pads containing the anterior right GP. The epicardial pacing electrode was used to deliver HFS to the anterior right GP. RSPV, right superior pulmonary vein; RMPV, right middle pulmonary vein; RA, right atrium close to the anterior right GP; RAA, right atrial appendage; RV, right ventricle. Panel B: Rapid RA stimulation initiated only nonsustained episodes of relatively organized AF (beginning of the tracing). HFS at the anterior right GP region (without capture of the atrial myocardium) was initiated during organized AF (top yellow arrow). The HFS resulted in rapid firing, recorded initially in the RA EGMs (RA3-4, bottom yellow arrow) located close to the stimulated anterior right GP, accelerating AF. Panel C: With the continuation of HFS for 30 seconds, AF became sustained and continued to accelerate in EGMs close to the anterior right GP (RA EGMs), exhibiting fractionated APs with very short CLs (< 30–40 ms) even after the termination of HFS. (Modified with permission from reference 16.)
Catheter Ablation of Autonomic Ganglionated Plexi in Patients with Atrial Fibrillation
Hiroshi Nakagawa, MD, PhD; Benjamin J. Scherlag, PhD; Warren M. Jackman, MD1
Introduction
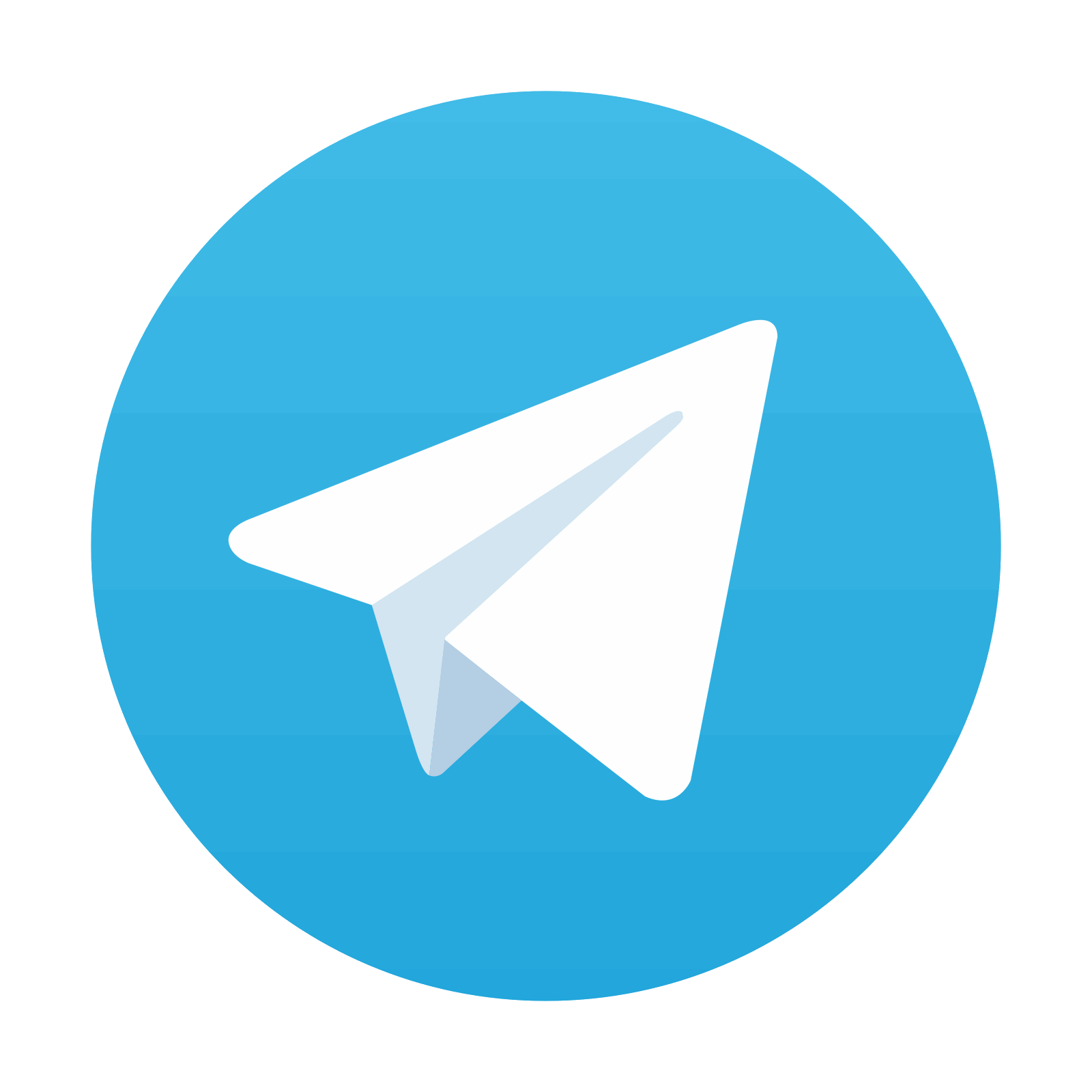
Stay updated, free articles. Join our Telegram channel

Full access? Get Clinical Tree
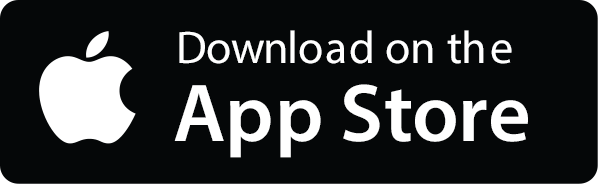
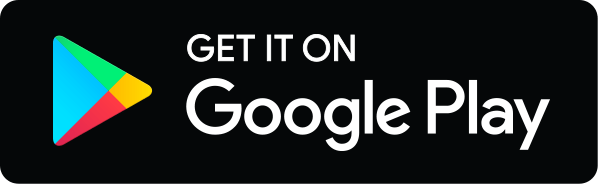