Carotid Artery Stenting
Jacqueline Saw
Jay S. Yadav
Carotid artery stenting (CAS) has recently been accepted as an alternative revascularization strategy to carotid endarterectomy (CEA) for the treatment of high-risk patients with significant extracranial carotid artery stenosis. Although CEA is traditionally considered the gold standard therapy to prevent strokes among patients with >50% symptomatic, or >60% asymptomatic carotid stenosis (1, 2, 3), this technique is invasive and may cause potentially serious complications. Thus, since carotid angioplasty was first introduced in 1980, this percutaneous technique has evolved rapidly, and now routinely is performed with the use of an emboli protection device (EPD) and a self-expanding nitinol stent. The remarkable findings from the pivotal Systolic and Pulse Pressure Hemodynamic Improvement by Restoring Elasticity (SAPPHIRE) trial have led to the recent recommendation by the Food and Drug Administration (FDA) Circulatory Systems Devices Panel (in April 2004) to approve the Precise stent in conjunction with the AngioGuard XP device (Cordis Corporation, Miami Lakes, Florida) for the treatment of carotid stenosis. An enormous upsurge in CAS procedures is anticipated in the near future.
EPIDEMIOLOGY OF CAROTID ARTERY STENOSIS AND STROKES
The primary goal of carotid revascularization is to prevent strokes, which have substantial healthcare and economic burdens to individuals and society. Stroke is the leading cause of disability and the third leading cause of mortality in the United States (4). About 750,000 patients present with strokes annually, with an economic burden surpassing $50 billion per year for the diagnosis and treatment of strokes (4). Approximately one-quarter of stroke victims will die within 1 year following the event, and about one-fifth will require institutional care within 3 months. Over 80% of strokes are ischemic in origin, with three-quarters involving the anterior circulation, and one-quarter involving the posterior vertebrobasilar circulation (5). Overall, up to one-third of all strokes in the United States are accounted by extracranial carotid stenosis.
Carotid artery stenosis is prevalent, and it is associated with an increased risk of stroke and other ischemic manifestations of systemic atherosclerosis (e.g., myocardial infarction [MI] and vascular deaths). In a community screening study, among asymptomatic individuals older than 60 with ≥1 risk factor (hypertension, heart disease, smoking, family history of stroke), the prevalence of extracranial carotid stenosis >50% on duplex ultrasound was 10.8% (6). This prevalence was higher among patients with heart disease (18.2%) or concomitant hypertension and heart disease (22.1%) (6). Furthermore, patients presenting with acute strokes had a much higher prevalence of extracranial carotid stenosis, up to 60% on duplex ultrasound (7).
The risk of stroke depends on carotid stenosis severity, symptom status of the patient, and specific lesion characteristics. It is well recognized that symptomatic patients (cerebrovascular event within 6 months) have a much higher stroke risk than asymptomatic individuals. In the North American Symptomatic Carotid Endarterectomy Trial (NASCET) study, medically treated symptomatic patients with severe stenosis (70% to 99%) had a 26% recurrent ipsilateral stroke-rate at 2 years (1), whereas patients with moderate stenosis (50% to 69%) had a 26% recurrent ipsilateral stroke-rate at 5 years (8). In contrast, medically treated asymptomatic patients in the ACAS (Asymptomatic Carotid Atherosclerosis Study) trial had a lower 5-year ipsilateral stroke-rate of 11% (3). Furthermore, the stroke incidence is higher with greater stenosis severity among patients having asymptomatic extracranial carotid stenosis: ˜1% annually with stenosis <75%, and over 3% to 5% annually with stenosis ≥75% on ultrasound (9,10). Recent studies using high-definition ultrasonography also have identified particular atherosclerotic
lesion characteristics (i.e., hypoechogenic and ulcerated lesions) that may be associated with higher stroke risk (11,12).
lesion characteristics (i.e., hypoechogenic and ulcerated lesions) that may be associated with higher stroke risk (11,12).
ANATOMY OF THE CEREBROVASCULAR CIRCULATION
A comprehensive knowledge of the aortic and cerebrovascular anatomy is necessary for interventionalists performing CAS. Specific attention to the aortic arch anatomy and tortuosity of the carotid arteries also helps to anticipate technical difficulties that may be encountered during interventions.
The normal great vessel branching in the following order—innominate artery, left common carotid artery (CCA), and left subclavian artery—is seen in only 65% of the population. The most common arch variant is the left CCA having a common origin with the innominate artery, or arising from the innominate artery (bovine arch); this occurs in up to 27% of arches. The left vertebral artery may have a separate origin between the left CCA and the left subclavian artery in ˜6% of cases. Much less commonly, the right subclavian artery may rise from the arch distal to the left subclavian artery (˜1%). Other very rare variants may also be encountered (e.g., two brachiocephalic trunks, single-arch vessel, a common carotid trunk, and a common subclavian trunk).
The aortic arch is classified according to the distance of the origin of the great vessels from the top level of the arch, with the widest diameter of the left CCA used as the reference distance (Fig. 18.1). In a type I arch, the origins of all great vessels arise within one diameter of the left CCA from the top of the arch. In a type II arch, all great vessels arise within two left CCA diameter lengths from the top of the arch. In a type III arch, all great vessels originate more than two left CCA diameters from the top of the arch. Type II and III arches occur more frequently among elderly patients with severe atherosclerotic disease, and these pose more challenges to both cerebral angiography and CAS.
Both CCAs divide into the external and internal carotid arteries (ECA and ICA) usually at the upper level of the thyroid cartilage (C4-C5 vertebral space; although, in some individuals, the bifurcation level may vary from C1 to C6). The ICA lies posterior and lateral to the ECA, and it begins with a fusiform dilated carotid sinus that contains sensory baroreceptor nerve endings from the glossopharyngeal nerve. Beyond the carotid sinus, the ICA continues superiorly in the cervical portion without any branches. The distal cervical ICA may be tortuous, kinked, or looped, which creates difficulty during percutaneous interventions. The ICA continues superiorly and enters the carotid canal at the base of the skull, where it becomes the petrous segment. Above the petrous bone, the ICA enters into the intracranial portion, which is divided into the precavernous, cavernous, and supraclinoid segments (Fig. 18.2). The cavernous segment travels within the S-shaped cavernous sinus and courses upward into the supraclinoid segment when it crosses the dura.
The ICA gives off its first major branch, the ophthalmic artery, anteriorly just below the dura (80% of the time; Fig. 18.3). The ICA then gives off the posterior communicating artery (PCOM) posteriorly, which joins the ipsilateral posterior cerebral artery (PCA) (Fig. 18.3). The ICA gives off the anterior choroidal artery usually 2 to 4 mm above the PCOM posteriorly, before bifurcating into the anterior cerebral (ACA) and middle cerebral (MCA) arteries (Fig.18.3). The ACA is divided into the A1 segment [proximal horizontal segment before the anterior communicating artery (ACOM)], and A2 segment (beyond the ACOM), which then gives off the pericallosal artery with its branches (orbitofrontal, frontopolar, and callosomarginal arteries; Fig. 18.4). The MCA is divided into the M1 segment (the proximal horizontal segment that terminates at the Sylvian fissure), M2 segment (insular segment, from the insular turn to the circular sulcus of insula hairpin), M3 segment (opercular segment, from the circular sulcus of insula to the lateral convexity), and M4 segment (cortical segment; Fig. 18.5).
Several collateral pathways may be present intrinsically, via intracranial or extracranial sources. The most common
intracranial collateral pathway is the circle of Willis, which connects the anterior circulation (ACA and MCA) to the posterior vertebrobasilar circulation, and also connects both hemispheres. It consists of the ACOM connecting both ACAs, and the two PCOMs that connect the distal ICA to the posterior cerebral arteries (PCA). However, some variation or anomaly of this complete circle is present in ˜60% of cases, with either absent, hypoplastic, or extra communicating arteries. Less common intracranial connections include embryonic communication between the ICA and the basilar artery via the persistent trigeminal artery, the persistent hypoglossal artery, and the proatlantal intersegmental artery. Connections also can occur between the terminal branches of cortical cerebral arteries via leptomeningeal collaterals. With ICA occlusion, several pathways of ECA to ICA collateral may also exist, particularly with anastomoses to the ophthalmic artery (via the supraorbital, medial frontal, and the nasal branches).
intracranial collateral pathway is the circle of Willis, which connects the anterior circulation (ACA and MCA) to the posterior vertebrobasilar circulation, and also connects both hemispheres. It consists of the ACOM connecting both ACAs, and the two PCOMs that connect the distal ICA to the posterior cerebral arteries (PCA). However, some variation or anomaly of this complete circle is present in ˜60% of cases, with either absent, hypoplastic, or extra communicating arteries. Less common intracranial connections include embryonic communication between the ICA and the basilar artery via the persistent trigeminal artery, the persistent hypoglossal artery, and the proatlantal intersegmental artery. Connections also can occur between the terminal branches of cortical cerebral arteries via leptomeningeal collaterals. With ICA occlusion, several pathways of ECA to ICA collateral may also exist, particularly with anastomoses to the ophthalmic artery (via the supraorbital, medial frontal, and the nasal branches).
DIAGNOSTIC IMAGING OF CEREBROVASCULAR CIRCULATION
Duplex Ultrasound
Duplex ultrasound is the noninvasive imaging modality most widely used to evaluate extracranial carotid stenosis. A standard duplex system that has high-resolution B-mode imaging, pulsed Doppler, and a frequency spectrum analyzer is necessary for a complete examination. These allow an assessment of the severity of the stenosis, as well as the characteristics of the plaque. The peak systolic velocity (PSV), end-diastolic velocity (EDV), and the ratio of ICA/CCA velocities are used as an indirect estimation of percentage diameter reduction. These measurements had been correlated retrospectively with angiographic stenosis measurements and then prospectively tested. The specific velocity thresholds or ratios differ for individual laboratories; Table 18.1 shows the current criteria used at the Cleveland Clinic Vascular Laboratory. A growing number of surgeons base the decision for CEA on ultrasoundestimated diameter stenosis alone. However, the accuracy of duplex ultrasound is very dependent on good sonographers and a laboratory that has a routine quality assurance program. Furthermore, duplex ultrasound may give falsely elevated velocity readings in tortuous vessels or in the presence of severe contralateral carotid stenosis (13). In a
recent meta-analysis of 64 studies comparing duplex ultrasound to angiography, the pooled sensitivity was 86% and specificity was 87% for diagnosing a stenosis of 70% to 99% severity (14). Therefore, although duplex ultrasound is a useful noninvasive screening test, it should not be the sole imaging test prior to revascularization; confirmation with another noninvasive test or conventional angiography is recommended.
recent meta-analysis of 64 studies comparing duplex ultrasound to angiography, the pooled sensitivity was 86% and specificity was 87% for diagnosing a stenosis of 70% to 99% severity (14). Therefore, although duplex ultrasound is a useful noninvasive screening test, it should not be the sole imaging test prior to revascularization; confirmation with another noninvasive test or conventional angiography is recommended.
![]() Figure 18.4. The anterior cerebral artery (ACA) and its branches (A) PA projection, (B) lateral projection. |
Magnetic Resonance Angiography
Magnetic resonance angiography (MRA) and contrast-enhanced MRA are increasingly used to complement duplex ultrasound as a noninvasive test. Newer MRA methods have allowed better visualization of the cerebrovasculature, from the thoracic aorta, to the carotid arteries, and to the intracranial circulation. However, MRA has several limitations, such as its dependence on flow, susceptibility to motion artifacts, and interference by metal instruments. Turbulent flow can lead to false interpretation of stenosis (pseudostenosis), including false interpretations of long stenosis distal to a focal tight lesion. The use of gadolinium-enhanced MRA allows the assessment of plaque ulcerations, decreases flow-related artifacts, and shortens image times (ability to image from the aortic arch to circle of Willis in ˜30 seconds). In the recent meta-analysis by Nederkoorn et al. (14), 21 studies on MRA were pooled and compared to conventional angiography. The pooled sensitivity was 95% and pooled specificity was 90% for diagnosing a stenosis severity of 70% to 99%. MRA also had a better discriminatory power than duplex ultrasound in this meta-analysis (14). Other studies have indicated that the combined use of duplex ultrasound and MRA
improved sensitivity (96%) and specificity (80% to 85%) for diagnosing extracranial carotid stenosis (15,16). Therefore, the combination of both these imaging studies is useful and may reduce the need for conventional angiography prior to CEA.
improved sensitivity (96%) and specificity (80% to 85%) for diagnosing extracranial carotid stenosis (15,16). Therefore, the combination of both these imaging studies is useful and may reduce the need for conventional angiography prior to CEA.
TABLE 18.1. CLASSIFICATION CRITERIA FOR INTERNAL CAROTID ARTERY STENOSIS AT THE CLEVELAND CLINIC VASCULAR LABORATORY | ||||||||||||||||||||||||||||
---|---|---|---|---|---|---|---|---|---|---|---|---|---|---|---|---|---|---|---|---|---|---|---|---|---|---|---|---|
|
Conventional Angiography
Diagnostic angiography is considered the gold standard for evaluating carotid artery stenosis. Although it gives only a two-dimensional depiction of the lumen, it has several advantages over noninvasive imaging. It allows the visualization of plaque characteristics (i.e., irregularity, ulceration, length, calcification, and the presence of thrombus), high-resolution assessment of the entire cerebrovascular circulation (from the aortic arch to the intracranial vessels), differentiation between critical stenosis and complete occlusion, and characterization of collateral flow. However, angiography does pose a risk of potential major complications (e.g., embolization, dissection, strokes). In the ACAS trial, the periprocedural stroke and death risk was 1.2% (3). A much lower complication rate is achievable in high-volume centers by experienced operators, when particular attention is given to avoid air and thrombotic embolisms. At the Cleveland Clinic, our periprocedural incidence of stroke was 0.3% among ˜700 cerebral angiographies, with no incidence of death.
A comprehensive diagnostic angiography is necessary before CAS, because it provides anatomic details to enable the physician to strategize the interventional approach and anticipate potential challenges. At our center, we routinely perform cerebral angiography with low-level anticoagulation, administering 2,000 to 3,000 units of intravenous heparin. A 5 Fr diagnostic catheter is used, starting with either a JR4 or angled glide catheter (Terumo Medical Corporation) for patients with Type I or II arches. When a Type III arch is encountered, we frequently start with a Vitek catheter (Cook, Inc.), progressing to Simmons catheters (Cook, Inc.) for particularly challenging cases (Fig. 18.6). The typical angiographic views taken with digital substraction are detailed in Table 18.2.
THE LIMITATIONS OF CAROTID ENDARTERECTOMY
Although large randomized trials have shown that CEA indisputably reduces strokes in patients with significant carotid stenosis, it can cause significant morbidities. The perioperative death or stroke rate was ˜6% in NASCET
and 2.3% in ACAS. The incidence of other complications were also high in NASCET: cranial nerve injury (7.6%), wound hematoma (5.5%), wound infection (3.4%), MI (0.9%), congestive heart failure (0.6%), arrhythmia (1.2%), and other cardiovascular problems (1.2%) (17). Furthermore, the large randomized trials included relatively healthy patients with carotid disease, thus their findings may not be applicable to “real-world” patients (1, 2, 3). Patient subsets who have higher complication rates following CEA include elderly patients (>75 to 80 years), those with coronary artery disease (CAD), renal dysfunction, severe pulmonary disease, congestive heart failure (CHF), restenosis following prior CEA, and contralateral carotid occlusion (18, 19, 20, 21).
and 2.3% in ACAS. The incidence of other complications were also high in NASCET: cranial nerve injury (7.6%), wound hematoma (5.5%), wound infection (3.4%), MI (0.9%), congestive heart failure (0.6%), arrhythmia (1.2%), and other cardiovascular problems (1.2%) (17). Furthermore, the large randomized trials included relatively healthy patients with carotid disease, thus their findings may not be applicable to “real-world” patients (1, 2, 3). Patient subsets who have higher complication rates following CEA include elderly patients (>75 to 80 years), those with coronary artery disease (CAD), renal dysfunction, severe pulmonary disease, congestive heart failure (CHF), restenosis following prior CEA, and contralateral carotid occlusion (18, 19, 20, 21).
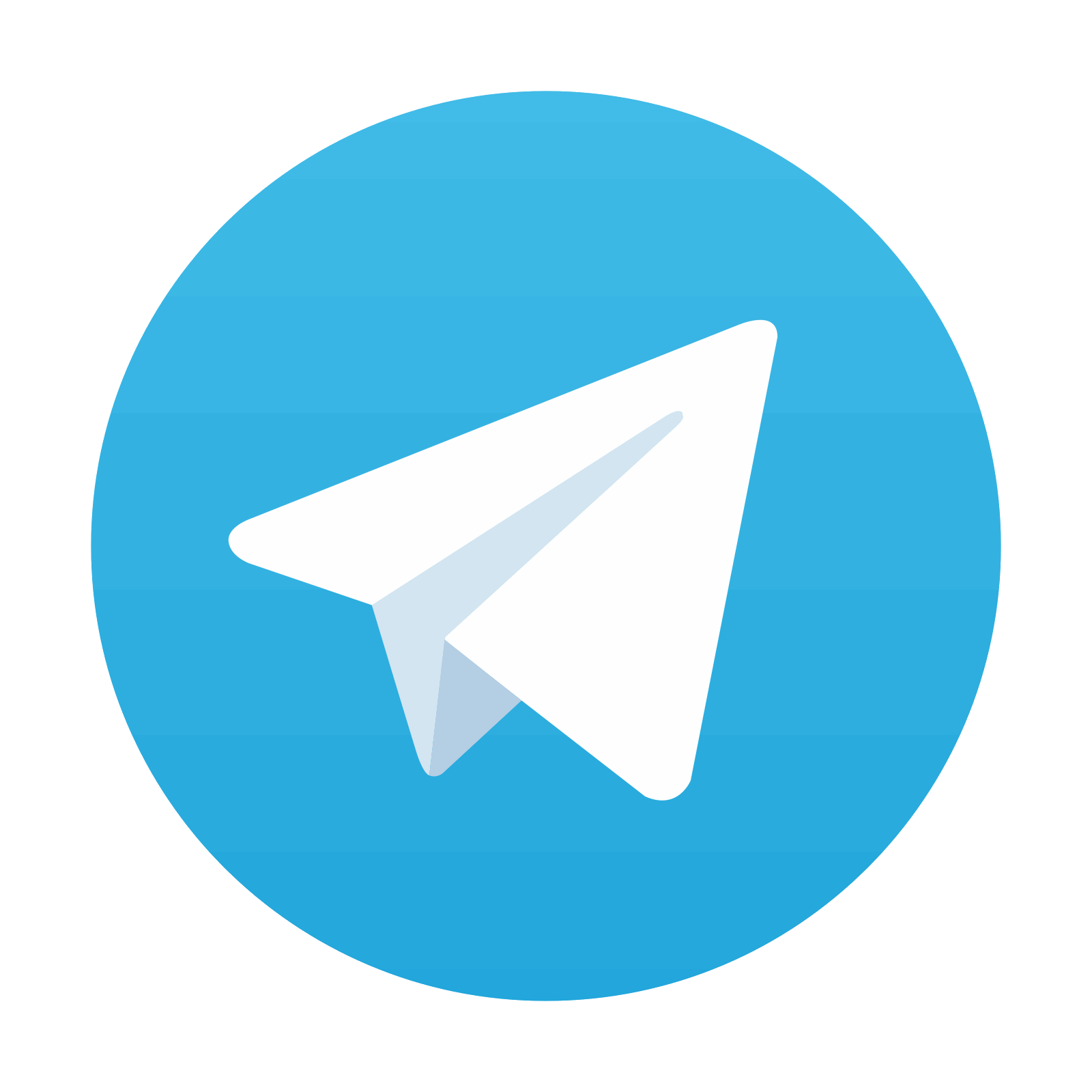
Stay updated, free articles. Join our Telegram channel

Full access? Get Clinical Tree
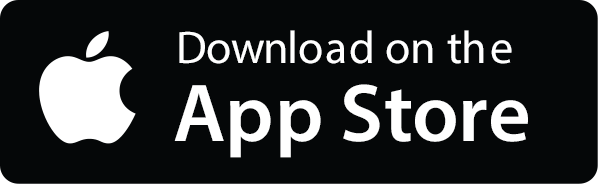
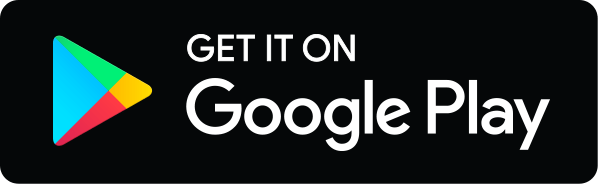