39 Carotid and Cerebrovascular Intervention
Introduction
Stroke is the leading cause of adult disability and the third leading cause of death in North America, Europe, South America, and Asia. The vast majority of strokes (~80%–85%) are ischemic in etiology. In the United States, atherosclerotic disease affecting the extra- and intracranial arterial circulation accounts for approximately 20% of ischemic strokes (Fig. 39-1) and thus is an important target for stroke prevention.1 Cerebrovascular intervention has evolved largely for the treatment of atherosclerotic disease with the goal of stroke prevention. Based on dramatic technological advances and increased operator expertise, these procedures can now be performed with a high rate of technical success. However, because of the potential for serious neurological complications from endovascular intervention in the cerebrovascular circulation, clear documentation of the safety of these procedures and of the overall clinical efficacy is of paramount importance. These considerations have raised the bar for cerebrovascular intervention compared with other peripheral vascular procedures. In the field of cerebrovascular intervention, carotid bifurcation intervention is unique in that the natural history of carotid artery bifurcation disease has been well defined, and large randomized trials have previously documented the clinical effectiveness of surgical revascularization for this disease. There is already a large evidence base supporting carotid intervention in specific patient subgroups, and several randomized trials are ongoing in the remaining patient populations. With over 140,000 and 280,000 carotid endarterectomy (CEA) procedures performed each year in the United States and worldwide, respectively, the potential impact of percutaneous revascularization has captured the interest of endovascular specialists who are keen to offer an alternative to surgery. In contrast, endovascular intervention in the cerebrovascular circulation outside of the carotid bifurcation has been hampered by two important considerations: the natural history of noncarotid cerebrovascular disease is less well defined, and there is a notable absence of randomized data documenting the benefit of revascularization compared with medical therapy alone. Despite these obstacles, dramatic advances in the technical aspects of these interventions have been made, and there is an increased recognition of the need for well-designed clinical studies that address these deficiencies. What is often underappreciated is that noncarotid bifurcation cerebrovascular disease is responsible for at least the same number of ischemic strokes as carotid bifurcation disease and represents an equally important target for stroke prevention. This chapter summarizes the current status of carotid bifurcation intervention and the most frequently performed noncarotid bifurcation cerebrovascular interventions, notably proximal vertebral artery and intracranial intervention.
Carotid Bifurcation Intervention
Carotid Bifurcation Atherosclerosis and Stroke
The carotid bifurcation has a remarkable predilection for the development of atherosclerosis, which is typically located at the origin of the internal carotid artery (ICA) (Fig. 39-2). This plaque is similar to that found at other sites throughout the arterial system in that it contains a dense cap of connective tissue with embedded smooth muscle cells and an underlying core of lipid and necrotic debris.2 Histological studies of plaque from the carotid bifurcation of symptomatic and asymptomatic individuals have revealed features associated with the development of symptoms that are similar to those associated with plaque vulnerability in the coronary circulation: i.e., reduced amounts of collagen, increased inflammation, thinning of the fibrous cap, and increased cholesterol in the necrotic core.2,3 Based on our current understanding, these processes result in plaque fissuring or rupture at the carotid bifurcation, causing either occlusive or nonocclusive thrombus formation. The dominant mechanism of stroke is believed to result from distal thromboembolism to the anterior cerebral circulation. However, a number of considerations such as the size and composition of the embolus, the presence of contralateral disease, the anatomy of the circle of Willis, and the activity of fibrinolytic pathways may attenuate or accentuate the clinical consequence of the pathological event, such that the same pathological event may result in a reversible neurological deficit (i.e., transient ischemic attack), an irreversible neurological deficit (i.e., stroke), or no symptoms at all.
Natural History of Carotid Artery Bifurcation Disease
In clinical practice, two dominant factors are used to determine the risk of ischemic complications from a lesion of the carotid artery bifurcation: the symptomatic status of the lesion and the severity of stenosis. Although many of these data are derived from the medical arm of the large randomized CEA trials performed between the late 1980s and early 2000s, these considerations continue to be used as the major criteria for choosing patients for endovascular procedures and for enrolling subjects in carotid endovascular trials. Symptomatic lesions of the carotid bifurcation are associated with a high risk of recurrent ischemic stroke. Based on data from the NASCET (North American Symptomatic Carotid Endarterectomy Trial), the risk of any ipsilateral stroke at 2-year follow-up in medically treated patients with symptomatic stenoses of 70% to 99% was 26%.4 Among patients with symptomatic stenoses of 50% to 69%, the 5-year risk of any ipsilateral stroke was 22.2%.5 There is a close temporal relationship between these recurrent strokes and the index event, with a steep exponential decline in risk within the first months, followed by a more gradual decline and ultimate normalization of risk at 2 to 3 years (Fig. 39-3). By contrast, asymptomatic lesions of the carotid bifurcation are associated with a much lower risk of ischemic stroke. Over a 5-year period following the diagnosis of an asymptomatic carotid stenosis, >60% by ultrasound, the risk of any stroke among medically treated patients in the ACST (Asymptomatic Carotid Surgery Trial) was 11%.6 Not surprisingly, the risk of stroke was constant over the duration of the study. The implication from these findings is that carotid revascularization should be performed as expediently as possible following a neurological event caused by a culprit symptomatic stenosis, while intervention for an asymptomatic lesion may be approached in a more elective fashion.7 Among symptomatic patients, a close relationship between the severity of stenosis as assessed by careful angiographic methods and subsequent risk of ipsilateral stroke has been demonstrated.8 The relationship is nonlinear, with a steep increase in risk associated with the tightest degree of stenosis (Fig. 39-4). However, for symptomatic patients with “near occlusion” of the internal carotid artery, defined as a stenosis causing sufficient obstruction to flow as to result in a decrease in the ICA diameter beyond the lesion (Fig. 39-5), there are data to suggest that the risk of recurrent stroke is reduced compared with patients with severe stenosis without features of near occlusion.9 One potential explanation for this finding is the reduced likelihood of distal cerebral embolization caused by diminished flow distal to a critical stenosis. Among asymptomatic patients, the association between stenosis severity and risk of subsequent stroke has been inconsistent.6,10 This finding likely underscores the heterogenous nature of carotid plaque histology in asymptomatic patients and suggests that assessments of plaque vulnerability may be a more potent predictor of recurrent events than severity of stenosis in this patient group. Today there are more sophisticated models to predict the risk of stroke in patients with carotid disease, particularly for symptomatic patients.11,12 In addition to stenosis severity, these models incorporate variables such as age, sex, nature of the presenting symptomatic event, time from index event, plaque surface morphology, and transcranial Doppler findings to provide a more individualized estimate of risk. However, these models have yet to be incorporated into patient selection criteria of randomized trials to more fully establish their clinical relevance.
Benefit of Carotid Revascularization
The benefit of carotid revascularization in patients with carotid artery disease has been documented in several randomized controlled trials (RCTs) comparing medical therapy with surgical revascularization (i.e., CEA). These data are extremely important in any discussion of endovascular therapy for carotid bifurcation disease as they form the cornerstone justifying revascularization in certain subsets of patients. In a pooled analysis of data from the three major RCTs in symptomatic patients,13 CEA as compared with medical therapy reduced the endpoint of stroke or operative death at 5 years in patients with carotid stenoses ≥50%, as assessed by carotid angiography using the NASCET criteria (Fig. 39-6). This benefit was more pronounced in patients with stenoses of 70% to 99% (absolute risk reduction [ARR] 15.3%, 95% CI 9.8-20.7) compared with those with stenoses of 50% to 69% (ARR 7.8%, 95% CI 3.1-12.5). In addition, the crossover of the event-free curves occurs very early in the patient cohort with 70% to 99% stenoses (1–2 months) compared with the patient cohort with 50% to 69% stenosis (1 year). The incidence of perioperative stroke and/or death in these studies was uniformly <6%; the benefits derived from CEA are predicated on the maintenance of similar procedural outcomes. No significant benefit was observed in patients with near occlusion of the carotid artery (ARR 0.1%, 95% CI -10.3-10.2), likely related to the lower risk of recurrent stroke with medical therapy in this group. These studies were performed in the late 1980s and early to mid-1990s; therefore the only stipulated medical therapy in the nonsurgical arm was aspirin. Contemporary medical therapy would likely attenuate the observed benefit associated with CEA. However, given the magnitude of the observed benefit associated with CEA in symptomatic patients, investigators have been reluctant to repeat randomized studies using contemporary medical therapy alone as a treatment arm. Compared with medical therapy, CEA has also been shown to significantly reduce the incidence of stroke or operative death at 5-year follow-up in asymptomatic patients with carotid stenoses ≥60%, as assessed by carotid ultrasound (11.8% vs. 6.4%, ARR 5.4%, 95% CI 3-7.8).6 It is important to emphasize that in this asymptomatic population, the early hazard associated with revascularization persists up to 2 years from the time of CEA. If the life expectancy of a patient is less than 5 years, then significant benefit should not be anticipated. In addition, participation in these trials involving patient’s with asymptomatic carotid stenoses required documentation of a perioperative stroke and death rate of <3% at the investigation site, and the generalization of these findings is predicated on reproducing similar procedural outcomes.
Carotid Artery Stenting: The Procedure14
Preprocedural Assessment
Prior to any CAS procedure, clinical assessment of the patient and anatomic assessment of the aortic arch and carotid/cerebral vasculature is essential. Advanced age (>80 years) has been associated with significantly worse outcomes with CAS and should be carefully considered for the appropriateness of intervention.15,16 Decreased cerebral reserve, manifest by the presence of dementia or cognitive impairment, and a history of prior strokes or lacunar infarcts increase the likelihood that distal embolization will be clinically manifest and is a relative contraindication for the procedure.17Anatomical assessments can generally be made using noninvasive studies, notably computed tomography angiography (CTA) and magnetic resonance angiography (MRA). CTA offers higher spatial resolution and superior visualization of the aortic arch compared with MRA and allows an assessment of the degree of calcification of the aortic arch and carotid bifurcation lesion that is not possible with MRA. Table 39-1 lists the anatomical features that should be reviewed using these studies and highlights the importance of each. Overall, these anatomical features allow the operator to more accurately determine the procedural risk and facilitate the planning of appropriate technique for procedural success.
TABLE 39-1 Anatomic Assessments Recommended Prior to Carotid Artery Stenting Procedure and Their Impact on Interventional Planning
Angiographic Assessment | Impact on Interventional Procedure |
---|---|
Arch anatomy | |
Lesion characteristics | |
ICA distal to lesion | |
Patency of external carotid artery |
CCA, common carotid artery; ICA, internal carotid artery; EPD, embolic protection device.
Interventional Technique
The technique for CAS placement follows a number of well-defined steps. Prior to CAS placement, all patients should receive aspirin. In addition, it is the authors’ practice to administer clopidogrel for at least 3 days prior to the procedure. During the procedure, anticoagulation using unfractionated heparin to achieve an activated coagulation time (ACT) of 275 to 300 seconds is standard.18 For patients with a contraindication to heparin, a direct thrombin inhibitor such as bivalirudin is administered.19 Currently there are limited data documenting the safety of bivalirudin for routine use during CAS. Most operators perform the procedure without the administration of sedatives, which enhances the ability to screen for any neurological change during the procedure.
Delivery of Embolic Protection Device
The use of embolic protection devices (EPDs) is now considered the standard of care during CAS. Compelling observational data support this recommendation. Several studies demonstrate that distal embolization is ubiquitous during CAS,20,21 and observational series have shown a significant association between decreased periprocedural rates of stroke and death and the use of EPDs.16,22 Over the last decade, three different device systems that provide protection from distal embolization at the time of carotid intervention have been developed.23,24 In clinical practice, the most popular and user-friendly of these systems is the filter-type EPD (Table 39-2, Fig. 39-7). These systems allow continued antegrade flow during carotid intervention, an important consideration for patients with compromised collateral flow to the ipsilateral carotid territory (e.g., patients with contralateral carotid artery disease or occlusion). Since filter-type EPDs have been used in most contemporary CAS registries and RCTs, more data exist to support their use in carotid intervention as compared with other EPDs. Based on the submission of these data to the FDA, multiple filter-type EPDs have received FDA approval (i.e., Accunet, Emboshield, Spider, Angioguard, FilterWire, and FiberNet). Although there is some variation in the individual design of these devices, they typically contain a polyurethane membrane, with pores of fixed size ranging from 80 to 140 µm between devices, supported by a nitinol frame. The Spider and Interceptor EPDs are unique in that the filter pores are formed by a nitinol mesh. Each filter is designed such that it is integrated with a 0.014-in. guidewire with a 3- to 4-cm shapeable floppy tip. With the exception of the Emboshield and Spider devices, the filter is fixed to the wire.
The technique for delivery of the filter-type EPD varies according to the design of the system. In systems such as the Accunet, FilterWire EZ, and Angioguard, the filter is delivered in a collapsed form across the carotid lesion on the attached guidewire. With the Emboshield system, a unique 0.014-in. wire (BareWire) is used to cross the lesion first; the filter is then delivered in a collapsed form over this wire and deployed over the distal portion of the wire. The Spider system allows the lesion to be crossed using any 0.014-in. wire, followed by a 2.9-Fr delivery catheter. It allows delivery of the Spider filter, which is integrated with a dedicated 0.014-in. wire that allows a small range of independent motion of the wire and filter. Predilation of the carotid lesion prior to delivery of the filter-type EPD is required in <1% to 2% of cases. If required, a small-caliber coronary balloon (i.e., 2.0 mm diameter) that minimizes the risk of distal embolization should be used. Regardless of the filter-type EPD that is used, the filter should ideally be deployed in a straight and nondiseased portion of the cervical ICA, which is typically in the distal cervical portion of the vessel. The presence of tortuosity or disease in the cervical portion of the ICA may require an alternate placement, but there must be at least 3 to 4 cm of distance between the proximal margin of the filter and the distal margin of the ICA lesion to allow subsequent delivery of interventional equipment. Distal occlusion balloon EPDs were the first type of EPD used during a carotid intervention (circa 1998). The only remaining example of this type of system is the Percusurge GuardWire device (Medtronic Vascular, Minneapolis, MN), which consists of an 0.014-in. angioplasty wire with a hollow nitinol hypotube and a distal compliant balloon that is inflated and deflated through the hypotube. The GuardWire is advanced across the carotid lesion with the balloon deflated. Complete interruption of antegrade flow is then achieved by inflating the balloon. Following treatment of the carotid lesion, a monorail Export catheter is used to aspirate the column of blood proximal to the balloon, thus removing any debris that may have embolized from the treatment site. The balloon is then deflated and the GuardWire removed. There are no randomized comparisons of carotid intervention using filter-type and distal occlusion EPDs. A retrospective comparison of outcomes from a large CAS registry showed no significant difference in in-hospital death or stroke between these systems (2.3% for distal occlusion EPDs vs. 1.8% for filter-type EPDs, P = 0.96).25 The most recent group of EPDs developed for carotid intervention comprises the proximal occlusion devices (e.g., the Parodi Anti-embolism System from Gore Medical, Flagstaff, AZ, and the MO.MA System from Medtronic). These systems attempt to protect the brain from distal embolization by eliminating antegrade flow in the ICA during the procedure and thus, essentially generating an endovascular clamp.26 Compliant balloons are inflated in the distal CCA and ECA, interrupting antegrade carotid flow and theoretically eliminating the risk of distal embolization from debris liberated during angioplasty and stenting. The success of such systems is predicated on adequate collateral circulation from the circle of Willis to maintain cerebral perfusion. With the Parodi device, retrograde flow is generated in the internal carotid artery by connecting the lumen of the catheter, whose tip is in the CCA and distal to the occlusive balloon to a catheter in the femoral vein. Consequently blood flows down its pressure gradient from the common carotid, through the blood return system, and to the femoral vein. In contrast, the MO.MA device simply creates a static column of blood in the internal carotid artery without continuous flow reversal, and removal of this unfiltered column of blood is achieved by aspiration with a syringe. Proximal EPDs appear particularly useful for cases in which tortuosity or disease distal to the carotid bifurcation lesion would preclude the use of filter-type or distal balloon-occlusion EPDs. Data with proximal EPDs are more limited than those for distal EPDs, but they are emerging. An initial large registry using a proximal balloon-occlusion system (i.e., PRIAMUS, which enrolled 416 “real world” patients with carotid disease) reported a high rate of technical success (~99%) and acceptable clinical outcomes (4.5% incidence of in-hospital stroke, death, and myocardial infarction [MI]). Two recent prospective registries using the MO.MA system have also been reported. In the ARMOUR study, 262 high-risk patients undergoing carotid artery stenting with embolic protection were enrolled, with a reported 30-day rate of stroke, death, and MI of 2.7%.27 In a larger, single-center registry of 1,300 patients who also underwent CAS with embolic protection from the MO.MA device, the 30-day rate of stroke and death was an impressive 1.4%, and the procedural success rate was 99.7%.28 While these results are encouraging, a direct comparison of proximal versus distal embolic protection in a randomized trial is needed.
Angioplasty and Stenting
Predilation
Following placement of the EPD system, the lesion is generally predilated to facilitate stent delivery. Low-profile coronary balloons with 3.0- to 4.0-mm diameters are generally used. Attempts to deliver the stent without predilation have been associated with a greater amount of athereombolism,17 likely related to increased trauma to the lesion with forcible passage of the stent across a tight stenosis.
Stent Selection and Placement
See Table 39-4. As is the case in other vascular territories, the ability to stent carotid lesions has allowed operators to achieve a predictable angiographic result, deal with procedural complications such as dissection and abrupt vessel closure, and improve long-term patency by eliminating vessel recoil. Initial attempts at carotid stenting using relatively inflexible stainless steel balloon-expandable stents (e.g., Palmaz, Cordis) were associated with acute technical success. However, their use was abandoned owing to the subsequent development of stent crushing, likely related to compression of the superficially located carotid stent from neck movements.29 This complication led to the development and use of flexible self-expanding stents that could conform to the tortuous anatomy of the carotid bifurcation and changes in vessel shape associated with neck movements (Table 39-3). The functional properties of these stents are defined by their metal composition and design.30 Nitinol, a nickel-titanium alloy, is the most widely used material for carotid self-expanding stents; because of its large elastic range, it confers an ability to withstand significant deformations. A variety of nitinol stents with either a closed- or open-cell design are available. The closed-cell design offers superior scaffolding at the cost of reduced flexibility. A single cobalt-based alloy stent with a closed-cell design is currently available (e.g., WALLSTENT, Boston Scientific, Natick, MA). Both the metal composition and design of this stent result in a more rigid stent with excellent scaffolding properties. Carotid stents come in a variety of sizes that match the typical diameter of the ICA and CCA (5 to 10 mm), and are generally 20 to 40 mm long. The nominal diameter of the stent used should be 1 to 2 mm larger than the diameter of the largest treated vessel (usually the CCA). Stent lengths are chosen to provide complete lesion coverage. Initially, all carotid stents were cylindrical. However, tapered stents that conform to the size mismatch between the ICA and CCA and facilitate treatment across the carotid bifurcation are now commonly used. When tapered stents are used, the majority of cases are performed using 6- to 8-mm or 7- to 9-mm-diameter stents that are either 30 or 40 mm long. When cylindrical stents are used, the majority of cases are performed using 8-mm-diameter stents of similar length (i.e., 30 to 40 mm). For most cases, all of the available carotid stents will achieve similar technical success and clinical outcomes. In the remaining cases (~25%), assuming that all stents were available to the operator, the choice of stent should be individualized and is largely influenced by arterial anatomy and lesion morphology.31 For example, stents with the greatest degree of flexibility (i.e., open-cell-design nitinol stents with large open-cell areas and highly flexible interconnecting bridges; e.g., Precise, Zilver) may be optimal for treating lesions in tortuous locations. Calcified lesions should be treated with stents with a high radial force and moderate outward expansive force, as provided by nitinol stents with a closed cell design (e.g., X-Act). Finally, lesions with the greatest risk for distal embolism should be treated with stents that provide greater vessel scaffolding (closed-cell nitinol or cobalt alloy stents; e.g., WALLSTENT, X-Act).
Postdilation
Following predilation, stent deployment, and postdilation, contrast angiography is performed to assess the angiographic result and detect any potential complications. When filter-type EPDs are being used, this practice allows the detection of “slow flow,” which is an important finding that requires special management.32 Slow flow is manifest by delayed antegrade flow in the ICA and may vary from complete cessation of antegrade flow to mild delay of ICA flow compared with the ECA (Fig. 39-9). Most likely this phenomenon is caused by excessive distal embolization of plaque elements that occlude the filter pores, compromising antegrade flow through the filter (Fig. 39-10). The phenomenon is frequent, occurring in 8% to 10% of cases,32,33 and is most commonly observed following postdilation of the stent (~75% of cases) and stent deployment (~25% of cases). Predictors of this event include treatment of symptomatic lesions, increased patient age, and increased stent diameter.32 In patients with slow flow, the column of blood proximal to the filter has not been appropriately cleared of debris embolized from the treatment site by the filter EPD. In an effort to prevent distal embolization of this debris at the time of filter retrieval, aspiration of 40 to 60 mL of blood from the column of blood proximal to the filter using an Export catheter, prior to retrieval of the filter EPD, is recommended. If slow flow is observed following stent deployment, poststent dilation is discouraged, as this would probably exacerbate the degree of embolization from the treatment site.
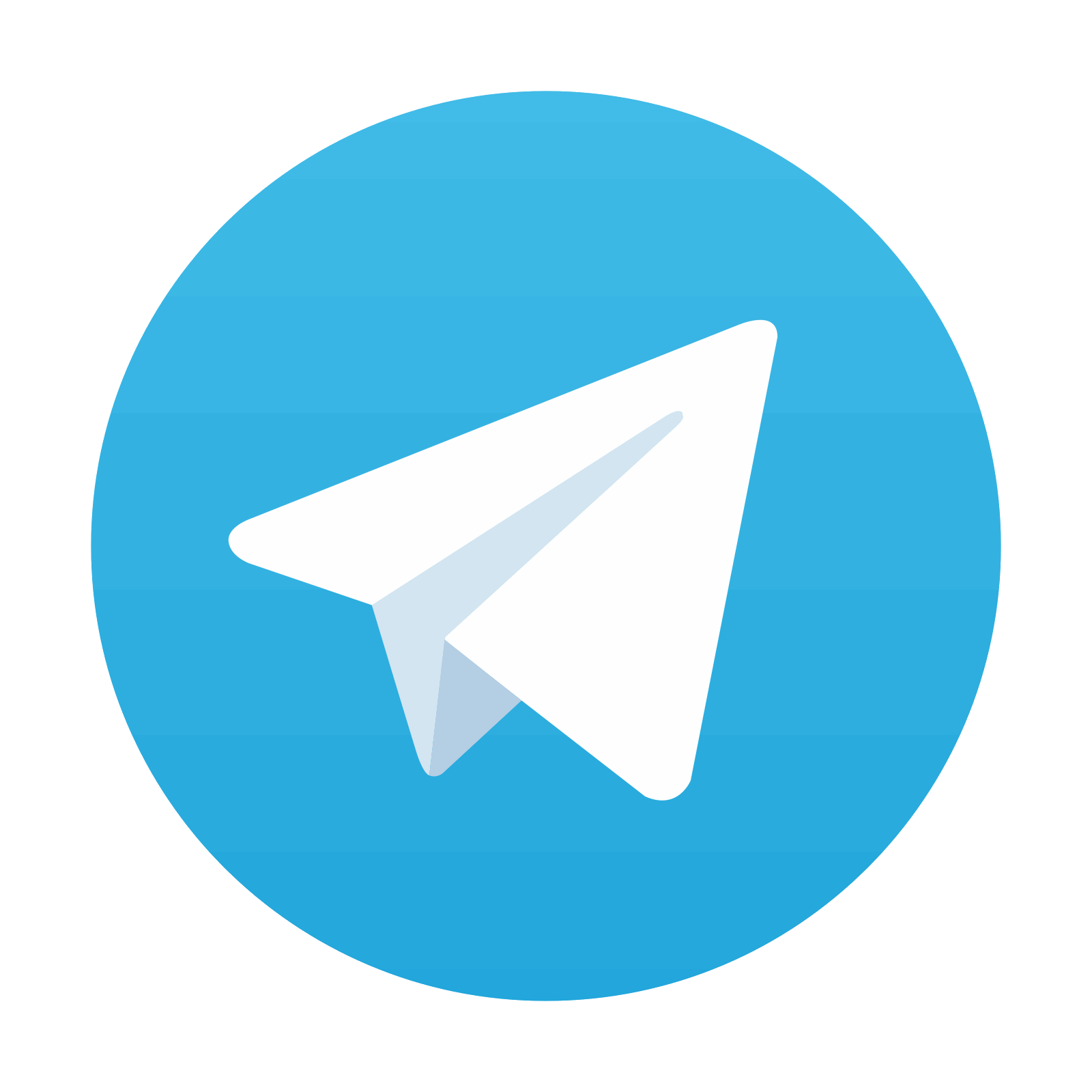
Stay updated, free articles. Join our Telegram channel

Full access? Get Clinical Tree
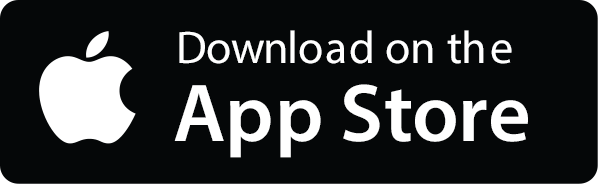
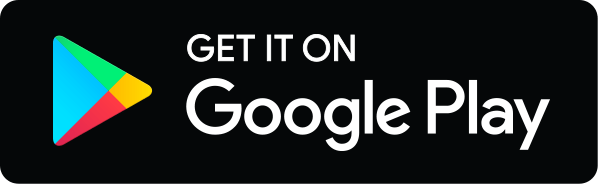