The care of infants, children, and adults with congenital heart disease (CHD) can be complex and challenging. In particular, the inpatient periprocedural setting can pose unique management challenges and represents one of the highest risk periods for the patient with CHD. Successful congenital heart surgery and interventional cardiac catheterization require a comprehensive, well-coordinated, team-based mode of care delivery that incorporates a solid knowledge base with sound clinical judgment. Management of the postprocedural patient should reflect a firm understanding of common congenital heart defects and surgical interventions, cardiopulmonary anatomy and physiology, advanced technical skills, pharmacology, and common complications. Patient and family-centered anticipatory guidance should be effective and confident with similarly comprehensive communication with primary care providers throughout the hospitalization and discharge process. This chapter provides an informative overview in the approach to the care of the postoperative and postcatheterization patient with CHD, specifically focusing on the immediate postprocedure and post–hospital discharge time periods.
The goals of preoperative intensive care unit (ICU) care are focused on stabilizing the patient (often with a ductal-dependent cardiac lesion), maintaining stable hemodynamics, and accurately identifying abnormal cardiac anatomy for appropriate procedural planning. In the current era, many neonates with CHD are diagnosed prenatally. Families should be encouraged to seek obstetrical care at a tertiary center with specialized pediatric cardiac services that allow for safe delivery of the infant while avoiding potentially life-threatening complications related to transfer and delays in care. However, a significant percentage of neonates with CHD continue to present in the postnatal period1 (Table 6-1). Immediate stabilization with initiation of prostaglandins, where appropriate, and transfer to a surgical and interventional cardiac center should be initiated promptly.
Diagnosis in Nursery, n = 73 (%) | Diagnosis After Discharge, n = 16 (%) | |
---|---|---|
Murmur | 38 | 25 |
Cyanosis | 32 | 0 |
Respiratory distress | 7 | 19 |
Shock | 4 | 38 |
Arrhythmia | 3 | 0 |
Other | 3 | 6 |
Multiple symptoms | 14 | 13 |
Once in the ICU setting, preoperative stabilization of the neonate consists of maintaining hemodynamic stability while avoiding preoperative morbidity that may impact the timing or effectiveness of definitive therapies such as surgery or interventional catheterization. Intravenous prostaglandin may be required for the neonate to maintain ductal patency prior to surgery. Hemodynamic support in the form of inotropes and/or mechanical ventilation may be required in the setting of severe cardiorespiratory compromise. Prostaglandin therapy and its associated side effects, including apnea, require close monitoring, although effective administration with low-dose infusions (0.01 μg/kg/min) have been successful without the need for tracheal intubation (Table 6-2).
Side Effect | |
---|---|
Common |
|
Uncommon |
|
Surgical techniques and intraoperative care advancements have extended the limits of patients being exposed to cardiopulmonary bypass (CPB). Technology has allowed for the relative miniaturization of elements of the CPB circuit, thereby minimizing the volume load and inflammatory responses observed after CPB. Yet there are several important considerations with regard to managing the infant and child after undergoing CPB. The challenges of balancing this vulnerable time period with essential life-sustaining interventions are not trivial and require a comprehensive understanding of perioperative factors, including oxygen-carrying capacity (eg, maintaining optimal hematocrit),2 temperature regulation, acid-base balance, and timing of intervention.
Ultrafiltration is a commonly used technique at the end of CPB that removes plasma water and inflammatory mediators from CPB circuit blood volume. This step is performed after separation from the bypass circuit in an effort to further minimize the inflammatory response after CPB. However, it is impossible to completely mitigate the inflammatory response from CPB. Furthermore, the resulting hemodilution from the bypass circuit yields a significantly decreased hematocrit, level of circulating clotting factors, and plasma protein, leading to dysfunction in the prothrombotic and anticoagulation balance of the coagulation system.3 In addition, the relative immaturity of the liver in neonates further exacerbates the dilutional coagulopathy due to diminished production of vitamin K–dependent clotting factors. Impaired thermoregulation requires intensive monitoring of core temperatures after CPB, especially when hypothermia is used as the primary strategy for surgical repair (deep hypothermic circulatory arrest [DHCA]). The immature lung of the neonate and infant predisposes the pulmonary vascular bed and surrounding parenchyma to increased reactivity, potential pulmonary edema, and pulmonary hypertension. The diminished fat and carbohydrate reserves and the higher metabolic rate and oxygen consumption observed in the neonate account for rapid hypoxia in the setting of apnea. The presence of intracardiac or extracardiac shunting can exacerbate the relative flow distribution between systemic and pulmonary vascular beds. Additional immature organ systems include the renal, central nervous, and immune systems. Renal vascular resistance is elevated in the neonate and infant, resulting in limited acid-base balance control, sodium reabsorption, excretion, and dilutional capabilities. Brain maturation is significantly delayed in infants with complex CHD compared with similarly gestational age–matched neonates.4 Additionally, immature neonatal and infant immune defenses result in poor antibody and complement generation as well as dysfunctional mononuclear cells.
Significant surgical and CPB stressors can lead to low cardiac output syndrome (LCOS) after cardiac surgery, predominately affecting neonates and infants but also affecting older children and adults who undergo complex operations with long myocardial ischemic times or in any patient with pre-existing ventricular dysfunction, atrioventricular valve regurgitation, or arrhythmias. To maintain adequate systemic perfusion, an age-appropriate heart rate for the patient’s clinical condition, adequate preload and intravascular volume, normal myocardial function, and appropriate afterload are ideal.
The interplay between diminished cardiac output, increased metabolic demand, inflammatory responses to CPB, and maladaptive responses to stress contributes to a predictable period of low cardiac output approximately 8 to 12 hours after separation from CPB circuit. This condition, often described as LCOS, is reflective of the transient myocardial ischemia and related myocyte swelling and noncompliance observed after CPB. Exposure of circulating blood volumes to the bypass circuit leads to the release of inflammatory factors that induces capillary leak and subsequent alterations in pulmonary compliance, vascular resistances, and loading conditions of the heart. Inadequate cardiac output leads to a period of relative oliguria, decreased skin perfusion, and systemic blood pressure and ultimately results in the requirement of fluid resuscitation, escalation of inotropic support, and vigilance. This transient depression in cardiac output generally improves after the first 24 hours as increased urine output is matched by decreasing inotropic support.5,6 Table 6-3 highlights the hemodynamic profile of conditions that can lead to cardiovascular instability in the postoperative period and should be differentiated from LCOS.
HR | CVP | Pulse Pressure | MAP | Urine Output | Capillary Refill Time | |
---|---|---|---|---|---|---|
Hypovolemia | ↑ | ↓ | Normal | ↓ | ↓ | ↑ |
Tamponade | ↑ | ↓ | Narrow | ↓ | ↓ | Normal/↓ |
LCOS | ↑ | ↓/↑ | Normal | ↓ | ↓ | ↑ |
Residual postoperative lesions will contribute greatly to the degree of LCOS postoperatively. For this reason, intraoperative transesophageal echocardiography is often used to identify significant residual lesions (intracardiac shunts, obstruction to inflow or outflow, valve regurgitation) prior to separation from CPB, thus allowing for repeat correction if indicated prior to discontinuation of support.
Postoperative ICU management should evaluate for significant contributors to decreased intravascular volume as measured by intracardiac lines (right and/or left atrial pressure) and systemic blood pressure monitoring. Systemic arterial catheters can aid in real-time blood pressure measurements while providing ready access for frequent phlebotomy and arterial blood gas sampling.
Contributors to postoperative intravascular volume depletion include hemorrhage, excessive diuresis or inadequate fluid administration, and excessive capillary leak after CPB. Blood products including packed red blood cells, platelets, and fresh frozen plasma should be administered when postoperative bleeding is prolonged or when abnormal coagulation laboratory values are observed. Recombinant factor VII should be administered in cases of significant postoperative hemorrhage resistant to routine blood product administration and repletion of fibrinogen with cryoprecipitate.7 Sudden cessation of bloody output from a previously draining chest tube in conjunction with tachycardia, rising intracardiac pressures, hypotension, and narrowing pulse pressure herald the clinical diagnosis of cardiac tamponade. Simple maneuvers to clear occluded chest tubes are required regularly to prevent fluid accumulation in the mediastinum and pericardial space. Ultimately, re-exploration of the mediastinum is required to restore hemodynamic stability when there is suspicion of tamponade. Complicated surgical procedures associated with long CPB times may result in myocardial swelling and/or excess bleeding. Both sequelae prohibit immediate sternal closure. Hemodynamic instability or ongoing bleeding can be managed more efficiently with delayed sternal closure, although mechanical ventilatory strategies must reflect the changes in functional residual capacity and respiratory compliance that occur with sternal closure. Delayed sternal closure can be associated with transient respiratory deterioration and a similar decrease in cardiac output often requiring a temporary escalation of ventilatory support and increase in inotropic support.8
Pharmacologic therapies are often used for additional hemodynamic support in the postoperative period and can include adrenergic-based therapies, phosphodiesterase inhibitors, and afterload-reducing agents such as α-blockers or direct vasodilators. The mechanism of action and side effect profile of each vasoactive agent should be weighed on an individual basis for each lesion and/or condition in the postoperative period (Table 6-4). Catecholaminergic medications such as dopamine, dobutamine, epinephrine, and isoproterenol can lead to excessive chronotropy and increased inotropic demands, thereby increasing myocardial oxygen consumption. Atrial and ventricular dysrhythmias can be seen with higher frequency when using chronotropic and inotropic agents. Additionally, activation of peripheral α-receptors may lead to increased peripheral vascular resistance with a concomitant decrease in cardiac output. Because of the many undesirable adverse effects associated with the use of high-dose endogenous or synthetic adrenergic therapies, afterload-reducing agents such as phosphodiesterase type III inhibitors (eg, milrinone) are being increasingly used in the postoperative period. Demonstrating both vasodilatory and lusitropic (myocardial relaxation) properties, these agents also act synergistically with β-agonists and have fewer side effects. Vasodilator therapy with afterload-reducing agents such as sodium nitroprusside or phenoxybenzamine can also improve cardiac output in select patient populations who experience elevated systemic vascular resistance in the postoperative period.
Drug | Dose Range | Receptor Binding | Major Side Effects | |||
---|---|---|---|---|---|---|
α1 | β1 | β2 | DA | |||
Dopamine | 2-20 μg/kg/min | +++ | ++++ | ++ | +++++ | Hypertension, ventricular arrhythmias, cardiac ischemia |
Dobutamine | 2-20 μg/kg/min | + | +++++ | +++ | N/A | Tachycardia, hypertension, ventricular arrhythmias, cardiac ischemia, hypotension |
Norepinephrine | 0.01-3 μg/kg/min | +++++ | +++ | ++ | N/A | Arrhythmias, bradycardia, peripheral ischemia, hypertension |
Epinephrine | 0.01-0.1 μg/kg/ min; mg IV every 3-5 minutes (max, 0.2 mg/kg) | +++++ | ++++ | +++ | N/A | Ventricular arrhythmias, severe hypertension, cardiac ischemia, cerebrovascular hemorrhage |
Isoproterenol | 0.01-2 μg/kg/min | 0 | +++++ | +++++ | N/A | Ventricular arrhythmias, cardiac ischemia, hypertension, hypotension |
Phenylephrine | 0.4-9 μg/kg/min; 0.1-0.5 mg IV every 10-15 minutes | +++++ | 0 | 0 | N/A | Reflex bradycardia, hypertension, severe peripheral and visceral vasoconstriction, tissue necrosis with extravasation |
Mechanical support in the form of extracorporeal membrane oxygenation (ECMO) has been used increasingly as an adjuvant therapy when conventional measures such as fluid resuscitation, inotropic support, and afterload reduction fail. Indications include ventricular dysfunction resistant to inotropic support, inability to wean off CPB, severe pulmonary hypertension with resultant right ventricular failure, intractable arrhythmias with compromised cardiac output, and palliations with catastrophic shunt occlusion. ECMO support has become the most widely used mode of mechanical cardiopulmonary support for children with CHD, both before and after heart surgery.9 Prior to initiation of ECMO support, one must consider the need for a dedicated team of ECMO specialists, immobilization of the patient, use of intensive and invasive monitoring, and the risks of coagulopathy, thrombosis, stroke, infection, and multiorgan failure. In addition, patients should be perceived as having a potentially reversible cardiac or pulmonary disease to be considered candidates for ECMO.
Mechanical assist devices, such as pulsatile and axial flow ventricular assist devices, are also being used increasingly as bridges to transplantation (in the case of CHD or cardiomyopathy) or recovery (in select cases of myocarditis/cardiomyopathy). These devices have several advantages including their ease of use, chronic support capabilities, mobility for cardiac rehabilitation, need for low-level anticoagulation, capability for biventricular support without oxygenation, and higher rate of extubation. Risks include thromboembolic complications, increased risk of presensitization in the pretransplant population, infection, and size limitations.
Respiratory mechanics and cardiovascular function are linked primarily through the changes in intrathoracic pressure that alter systemic venous return to the heart, as well as through alterations in pulmonary vascular resistance and left ventricular afterload. Positive pressure ventilation increases intrathoracic pressure during inspiration, thus decreasing venous return to the right atrium and reducing right ventricular preload. This decrease in venous return is mirrored by the left ventricle and leads to decreased stroke volume with a subsequent decrease in cardiac output. Additional alterations in lung recruitment may exacerbate changes in pulmonary vascular resistance. Although pulmonary vascular resistance will reach a nadir at function residual capacity, alveolar overrecruitment above function residual capacity or underrecruitment at residual volume can lead to subsequent increases in pulmonary vascular resistance.
The predominant effect of increased intrathoracic pressure during inspiration with positive pressure ventilation is likely to be decreased systemic venous return with a subsequent decrease in cardiac output. Ventilatory strategies should be chosen carefully to avoid adverse effects on the cardiovascular system. Patients most affected by ventilatory changes are those with significant right ventricular diastolic dysfunction, those at risk for pulmonary hypertension, and those with cavopulmonary anastomoses. Although corrective surgeries may improve pulmonary compliance in cardiac lesions with significantly elevated pulmonary blood flow, pulmonary mechanics and anatomy are also affected by CPB, trauma, and/or intercurrent infection. Changes such as prominent swelling of the mucosa due to hyperemia or edema, excessive or highly viscous secretions, hyperactive bronchial smooth muscle, and/or extrinsic compression by neighboring structures can all contribute to decreased myocardial compliance. In addition, pulmonary edema, pneumonia, and atelectasis most commonly lead to lower airway and alveolar abnormalities.
Respiratory failure may also result from diaphragmatic paresis or outright paralysis, particularly in neonates and infants, who rely on diaphragmatic contraction for chest wall expansion more than older children. Intraoperative injury to the phrenic nerve (usually left-sided) is most commonly caused by direct trauma, although thermal injuries have also been reported.10 Diagnosis is confirmed by fluoroscopy or by bedside ultrasonography demonstrating paradoxical or paretic motion of a raised hemidiaphragm in conjunction with paradoxical abdominal motion. In such cases, surgical diaphragmatic plication is recommended should the patient demonstrate increased work of breathing and/or failure to wean from mechanical ventilation.
Pleural effusions may also interfere with postoperative recovery and can lead to reintubation and prolonged length of stay in hospital. Patients undergoing specific procedures such as right ventriculotomies with subsequent transient right ventricular dysfunction and total cavopulmonary anastomoses (Fontan operation) are more likely to accumulate pleural effusions due to elevated central venous pressures and impaired lymphatic drainage. Evacuation of the pleural space with chest tubes subsequently allows for improved ventilation and less intrathoracic airspace competition.
Although many postoperative CHD patients remain intubated and mechanically ventilated after surgery, specific patient populations may benefit from early extubation. Older children, those without pulmonary hypertension, and those with less complex lesions requiring shorter CPB or DHCA times have been shown to be extubated successfully in the operating room or within hours of arrival to the ICU.11 Several criteria should be evaluated in order to promote a higher likelihood of success of extubation in the postoperative or postprocedural cardiac patient. The patient’s hemodynamic status should be evaluated with the goal of maintaining the patient with a normal heart rate and rhythm as well as adequate systemic perfusion. Higher inotropic support may require delayed extubation in order to decrease the work of breathing and improve oxygen delivery to the myocardium and other vital organs. Hemostasis should also be achieved. Lastly, the patient’s neurologic status should be fully evaluated after the appropriate titration of sedative and analgesic regimens to allow for spontaneous breaths without the presence of respiratory depression or seizure activity.
Pulmonary hypertension is a major cause of morbidity and mortality following cardiac surgery in the pediatric population, especially after repair of CHD with large left-to-right shunts such as unrestrictive ventricular septal defects, complete atrioventricular (AV) canal defects, total anomalous pulmonary venous connection (TAPVC), large patent ductus arteriosus, truncus arteriosus, and dextro-transposition of the great arteries. Triggers of postoperative pulmonary hypertensive crises include hypoxia, hypercarbia, hypothermia, and hypoglycemia. Recent advances in the investigation of the pathophysiology of pulmonary hypertension, including the role of vascular endothelium in the production of vasodilators and vasoconstrictors such as endothelin, have advanced our understanding of pulmonary vascular biology and how pulmonary vascular resistance can be altered following CPB.12 Early surgical repair in younger patients may also have contributed to the lower incidence of pulmonary hypertension in the CHD population over the past decades and should be considered in the current management of patients with early signs of pulmonary hypertension. In addition to avoiding known triggers, analgesia and sedation in the ICU play a large role in avoiding or treating postoperative pulmonary hypertensive crises. Inhaled nitric oxide (NO) is a specific pulmonary vascular smooth muscle relaxant that can lower pulmonary artery pressure in a number of diseases without the unwanted effect of systemic hypotension. Prophylactic and therapeutic use of inhaled NO has been reported after repair of obstructed TAPVC, Fontan operation, complete AV canal repair, and a variety of other anatomic lesions. Possible toxicities of inhaled NO include methemoglobinemia, production of excess nitrogen dioxide, and injury to the pulmonary surfactant system. Additionally, abrupt withdrawal of NO can lead to rebound pulmonary hypertension, thus requiring a slow wean from support. Additional therapies that may attenuate the withdrawal response to inhaled NO that may also treat active pulmonary hypertension include oxygen and the use of sildenafil, a phosphodiesterase type 5 inhibitor.13 Pretreatment with sildenafil, which is well tolerated and available as an oral preparation, produces acute and relatively selective pulmonary vasodilatation while acting synergistically with NO. Hyperventilation was previously proposed as a strategy for the management of pulmonary hypertension but is no longer used due to the compensatory increase in systemic vascular resistance, decrease in cardiac output, reduction of both coronary and cerebral blood flow, and potential for worsening outcome of acute lung injury if large tidal volumes are used. However, adequate ventilation and avoidance of hypercarbia remains important.
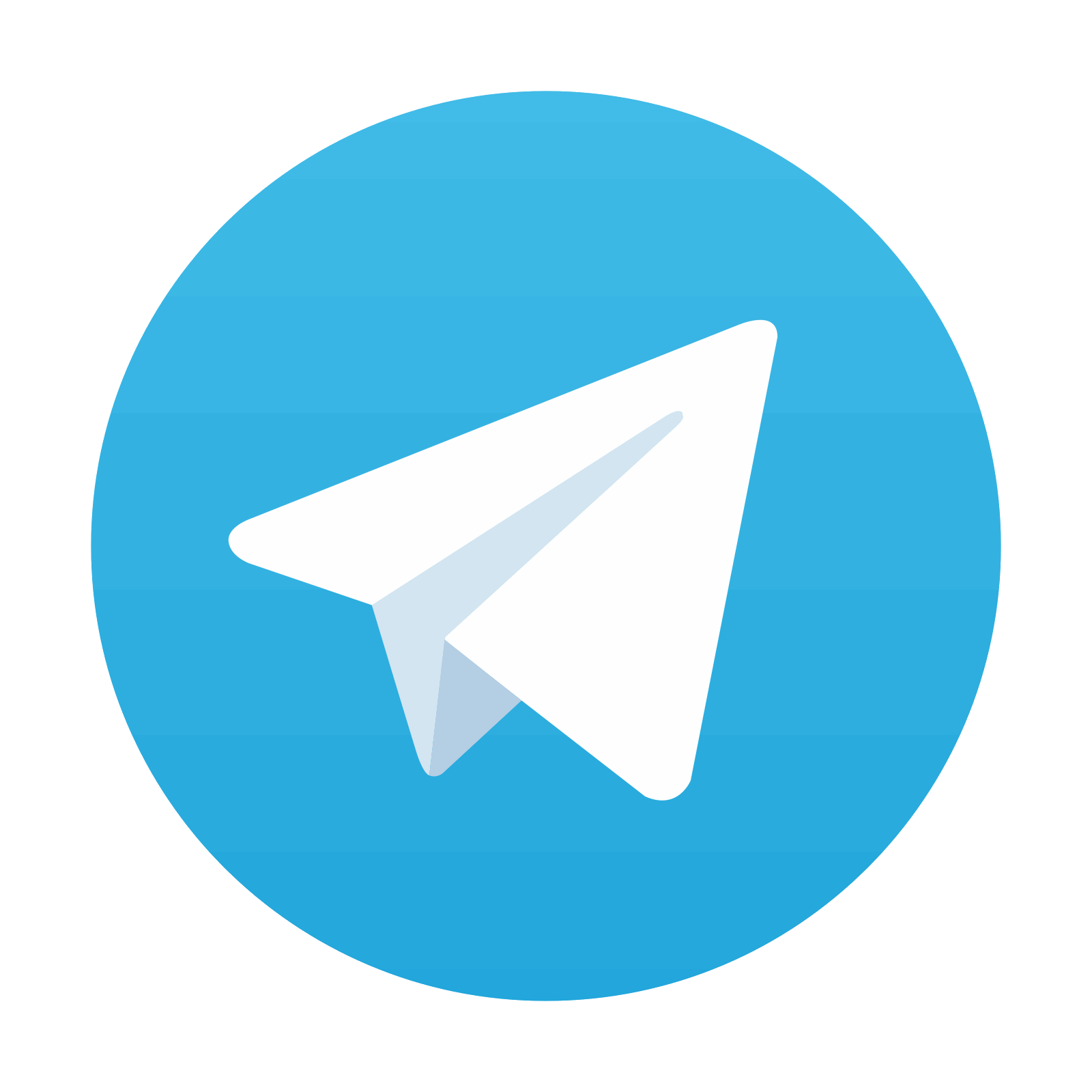
Stay updated, free articles. Join our Telegram channel

Full access? Get Clinical Tree
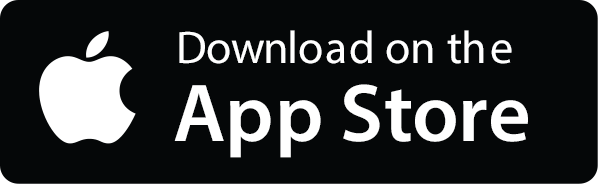
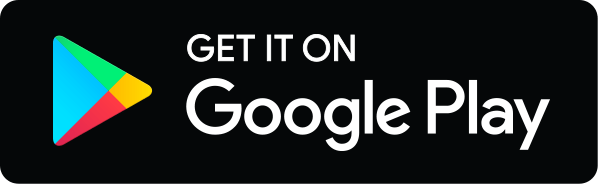