Fig. 7.1
Heart rate variability levels in patients with subsequent heart failure events (With permission from Ref. [10])
Subsequently, several novel HRV methods were introduced into device-based diagnostic packages, each demonstrating prognostic value of remote monitoring of patients with HF [14]. The limitations of HRV measurements are important to understand. The measurement cannot provide direct guidance for medication changes intended to maintain clinical stability and, as such, can only provide a remotely available “early warning system” that identifies either patients who chronically are at high risk for hospitalization (basal HRV) or are in the process of decompensation (dynamic HRV). Additionally, HRV cannot be measured in patients with atrial fibrillation or with >75 % of sensed heart beats as “non-sinus” initiated [9].
This crucial first step, however, was important in stimulating the development of monitoring systems designed to remotely enable practitioners to improve surveillance of patients with symptomatic HF. The search continued for a clearer signal that could provide remotely obtainable information concerning the pathophysiology of decompensation with congestion in HF patients. The ideal signal would appropriately follow progressive changes in body volume, which are typically associated with decompensation and hospitalization, inform clinical decision making by guiding changes in medication dosing, and allow confirmation of successful treatment with the goal of maintaining stability rather than reacting to the consequences of severe decompensation.
7.3 Intrathoracic Impedance Measurement
Measuring intrathoracic impedance with an implanted device was the next step in pursuit of a more direct marker of lung fluid and excess volume in HF patients at risk for decompensation. The concept was based on a very simple calculation of electrical impedance of a subthreshold impulse as it traversed the thorax from the right ventricular (RV) lead of an implanted electronic device to the pulse generator [5]. The theoretical advantage to this approach was that the vector distance between the RV and the pulse generator was fixed, which led to the hypothesis that a change in impedance would only occur in the presence of a change in lung fluid composition. Early studies in small numbers of HF patients demonstrated that intrathoracic impedance measurements correlated well to pulmonary capillary wedge pressure and the amount of fluid removed from acutely decompensated patients. Subsequent small trials suggested that meaningful changes in intrathoracic impedance measurements were apparent up to 14 days prior to hospitalization in decompensating patients. When coupled with other device-based diagnostics, such as HRV, activity, or nighttime heart rate, intrathoracic impedance changes were associated with increased hospitalization risk in the near term.
Unfortunately, this measurement was unable to guide medical therapy in hopes of maintaining stability in patients with HF. In an early terminated prospective randomized trial called DOT-HF performed at multiple European sites, changes in intrathoracic impedance were tied to a patient alert system generated by the implanted device [15]. The intent of the trial was to act on patient alerts by intensifying diuretic therapy and averting decompensation leading to hospitalization. The lack of clear understanding of false-positive and false-negative rates led to confusion which resulting in increased rate of hospitalizations in the monitored group compared to standard of care [15]. This disappointing result led to early termination of the PRECEDE-HF trial, which was a US-based investigation of the impact of intrathoracic impedance on HF outcomes.
The collective experience with implanted device-based intrathoracic impedance measurements remains unclear. Predictive statistical evaluation may help clarify what other events, such as pneumonia, COPD exacerbation, non-critical volume shifts, or device abnormalities, change the impedance signal without an excess in body volume. A large multicenter randomized trial is ongoing in Europe to further evaluate the value of intrathoracic impedance and guide its use in remote monitoring of patients with HF [16], but preliminary reports in an abstract presentation found no benefit of intrathoracic impedance in reducing hospitalizations in 1,002 patients followed for over 12 months [late breaking clinical trials, European Society of Cardiology, London, 2015].
7.4 Implantable Hemodynamic Monitoring
Traditional history and physical examination monitoring of patients with HF in clinical settings are designed to estimate body volume and organ perfusion. As over 90 % of hospitalizations involve excesses in body volume resulting in signs and symptoms of congestion, monitoring body volume is the key element of frequent assessment of high-risk patients [17]. HF disease management strategies typically involve frequent physical assessment by HF specialists with the hopes of early detection of volume accumulation that may lead to changes in diuretics or maximization of neurohormonal interventions.
The obvious limitation of these expert evaluations is that the examination and assessment cannot be done frequently or remotely. Patients are typically instructed to weigh themselves daily as a surrogate of body volume with the hypothesis that sudden increases in body weight would lead to medication changes in time to avoid acute decompensation and hospitalization. The common theme of all these clinical tools is to estimate cardiac filling pressures to guide medical management. Unfortunately, each maneuver, including physical examination and daily weights, is insensitive for detecting significant changes in filling pressures. In one study the sensitivity of a 2 kg body weight change in 72 h for predicting impending hospitalization was measured at 9 % [18–22]. Therefore, traditional clinical tools, even when remotely available as with body weight, are insensitive for assessing cardiac filling pressures which rise predictably as patients progress to decompensated state.
In fact, other typical parameters monitored in patients with HF, such as intrathoracic impedance and serial BNP measurements, were validated as surrogates of cardiac filling pressures (PCWP) [23]. Until recently, sensor technology suitable for the hostile environment of the human body was not available. However, in the mid-1990s Steinhaus and colleagues tested the first fully implantable hemodynamic monitoring system, which included a pressure transducer and oxygen saturation probe implanted in the right ventricular outflow tract (RVOT) in patients with HF [24]. This feasibility study found that the oxygen saturation sensor failed at a fairly high rate leading to removal of this component in subsequent designs. The Chronicle device was then developed using the basic framework of a pacemaker with a single lead housing the transducer implanted in the RVOT using a passive tine tip that also measured the local electrogram. The lead and transducer were then attached to a battery and memory system housed in a pulse generator that was implanted subcutaneously [25–27]. The implantation of this device was associated with typical complications of single lead pacemakers such as lead dislodgement, pocket infection, and system failure. Ultimately, the polyurethane lead design of the sensor was found to have an unacceptably high rate of single-source failure after 4 years of follow-up, and the system development was abandoned [28]. The clinical trials associated with this device, however, provided a rich database that described HF pathophysiology in a manner that was never seen before. A summary of clinical investigation using implantable hemodynamic monitoring systems and outcomes is shown in Table 7.1.
Table 7.1
Summary of clinical investigation using implantable hemodynamic monitoring devices
Trial | Level of evidence | Mean duration of follow-up | Annualized reduction in HF hospitalization rates | Patient population |
---|---|---|---|---|
COMPASS Feasibility [26] | Historic control | 17 months | 57 % (p < 0.01) | NYHA classes III–IV HFrEF n = 32 |
COMPASS-HF [29] | Randomized prospective single blinded | 6 months | 21 % All HF events (p = 0.33) 36 % time to first (p < 0.01) | NYHA classes III–IV Any EF n = 274 |
REDUCEhf [28] | Uncompleted randomized single blinded | 12 months | N/A | NYHA classes II–III ICD indication N = 400 |
Randomized prospective single blinded | 6 months primary 17 months supplemental | 28 % (p < 0.001) 37 % (p < 0.0001) | NYHA class III Any EF N = 550 |
The Chronicle device measured right ventricular systolic, diastolic, and mean pressures and had an algorithm to estimate pulmonary artery (PA) diastolic pressure (Fig. 7.2). PA diastolic pressure estimate was the pressure in the RVOT at the time of maximum dP/dt, which corresponded to the opening of the pulmonary valve. Heart rate and other derivatives of the pressure signal were recorded beat to beat, averaged, and stored in a user-defined time bin. Hemodynamic information was sent by a radio-frequency (RF) uplink to a bedside device that transmitted the information to a secured website for investigator and provider review. The first clinical experience with this device proved it to be precise and accurate over time [25]. In addition, it became clear that pressures increased as patients decompensated and returned to baseline with successful treatment of decompensated HF [26]. Significant hospitalization reductions, using historic control analysis, were observed in the feasibility patients when pressures were validated and then used to guide medications. This supported the hypothesis that implantable hemodynamic monitoring may be a superior means to manage high-risk patients with persistently symptomatic HF.
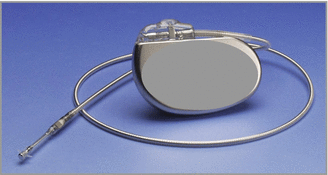
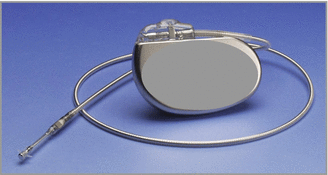
Fig. 7.2
Implantable hemodynamic monitoring system used in the COMPASS-HF trial (Adapted from Ref. [29])
The first prospective, randomized, single-blinded, implanted control trial evaluating the strategy of hemodynamic guided HF care was called COMPASS-HF, which enrolled 274 patients with NYHA class III or IV HF symptoms and a hospitalization in the previous 6 months [29]. The primary end point of the trial was a composite of HF-related events that included emergency department visits requiring intravenous HF care, intravenous medication interventions in the office, and HF-related hospitalizations evaluated after 6 months of hemodynamic-guided care in the treatment group vs. standard of care in the control group. The trial was designed with 80 % power to detect a 30 % reduction in all primary end points assuming a control group rate of 1.2 events/6 months. These assumptions were quite ambitious and led to a grossly underpowered study unable to adequately test the hypothesis. In contrast to the predicted event rates, the COMPASS-HF control group did very well with 0.85 events/6 months, which rendered the treatment group’s 21 % reduction in all events (0.67 events/6 months) nonsignificant [29]. A retrospective time-to-first-event analysis revealed a significant 36 % reduction in the primary end point. Additionally, approximately 17 % of the patients had NYHA class IV symptoms at enrollment. These patients seemed to have an increased event rate in the treatment group. Ambulatory NYHA class III patients seemed to benefit from hemodynamic monitoring, but this was a retrospective subgroup analysis in a trial that did not statistically meet its primary end point. The findings, then, supported the hypothesis, but did not provide sufficient evidence for benefit to outweigh the risks associated with implantation of the device.
The COMPASS-HF trial provided a unique and never-before-seen perspective into cardiovascular pathophysiology. These significant contributions illustrate the value of “listening to the heart.” Continuous hemodynamic evaluation of ambulatory HF patients demonstrated that elevated cardiac filling pressures were common and elevations had prognostic value [30]. Very importantly, the COMPASS-HF experience found that there were two components to hemodynamic-guided care and both must be addressed for ultimate clinical success. Whereas it was clear that pressures progressively and predictably increased from baseline as patients decompensated [3, 4, 6, 7], only reacting to changes was not complete. Stevenson and colleagues reported that pressure levels were clearly related to subsequent risks for HF events [30]. Their retrospective analysis from COMPASS-HF found that patients entering the trial with elevated pressures who had their pressures treated and lowered were among those with the lowest event rates. Patients entering the trial with high pressures that persisted throughout the trial had the highest event rates, which were equal to patients who entered with lower pressures that experienced persistent increases over time. The final group, patients with pressures that stayed low from implant to the end of follow-up, were also at low risk. This concept led to a better understanding of the clinical relationship between filling pressures and perfusion. Although perfusion is important to monitor, most patients with chronic HF do not require high pressures to maintain cardiac output. In fact, the evidence is clear that higher filling pressures are detrimental and lead to high risk for decompensation [30]. The retrospective data by Stevenson and colleagues developed a significant hypothesis that was to form an important component of the CHAMPION Trial protocol.
Further insight into the pathophysiology of HF was provided by Zile and colleagues who demonstrated that the hemodynamic characteristics of patients with HF and reduced ejection fraction (HFrEF) were similar to those patients with HF and preserved ejection fraction (HFpEF) [3]. This was an important point as the only consensus agreement about therapy for HFpEF patients was control of volume (i.e., filling pressures). Albeit pressures tended to change with a steeper pressure/time relationship in HFpEF patients, they still significantly increased 17–21 days before decompensating patients were hospitalized. This further supported the general hypothesis that this important and underserved group of HF patients may benefit from hemodynamic-guided HF management.
Further development of the Chronicle device included adding a single-chamber defibrillator to the circuitry to evaluate the impact of hemodynamic-guided HF management in patients with an indication for ICD therapy. The REDUCEhf trial was then designed to evaluate up to 1200 HF patients followed for 12 months with a similar end point of HF events used in COMPASS-HF [27]. Unfortunately, the lead failure problem in the Chronicle system was discovered after 400 patients were enrolled in the REDUCEhf trial, but the trial did discover that patients with NYHA class II HF following a previous hospitalization had a much different event rate in the year following implantation of the monitor. Event rates in the NYHA class II patients were very low after implantation (0.24 events/patient/year) suggesting that hemodynamic monitoring in these patients, if the goal was to reduce hospitalization rates, may not be effective [28]. Additionally, patients with treated arrhythmias tended to have higher filling pressures, although a high-resolution view of pressures temporally associated with ventricular arrhythmias was difficult to establish with the technology [31].
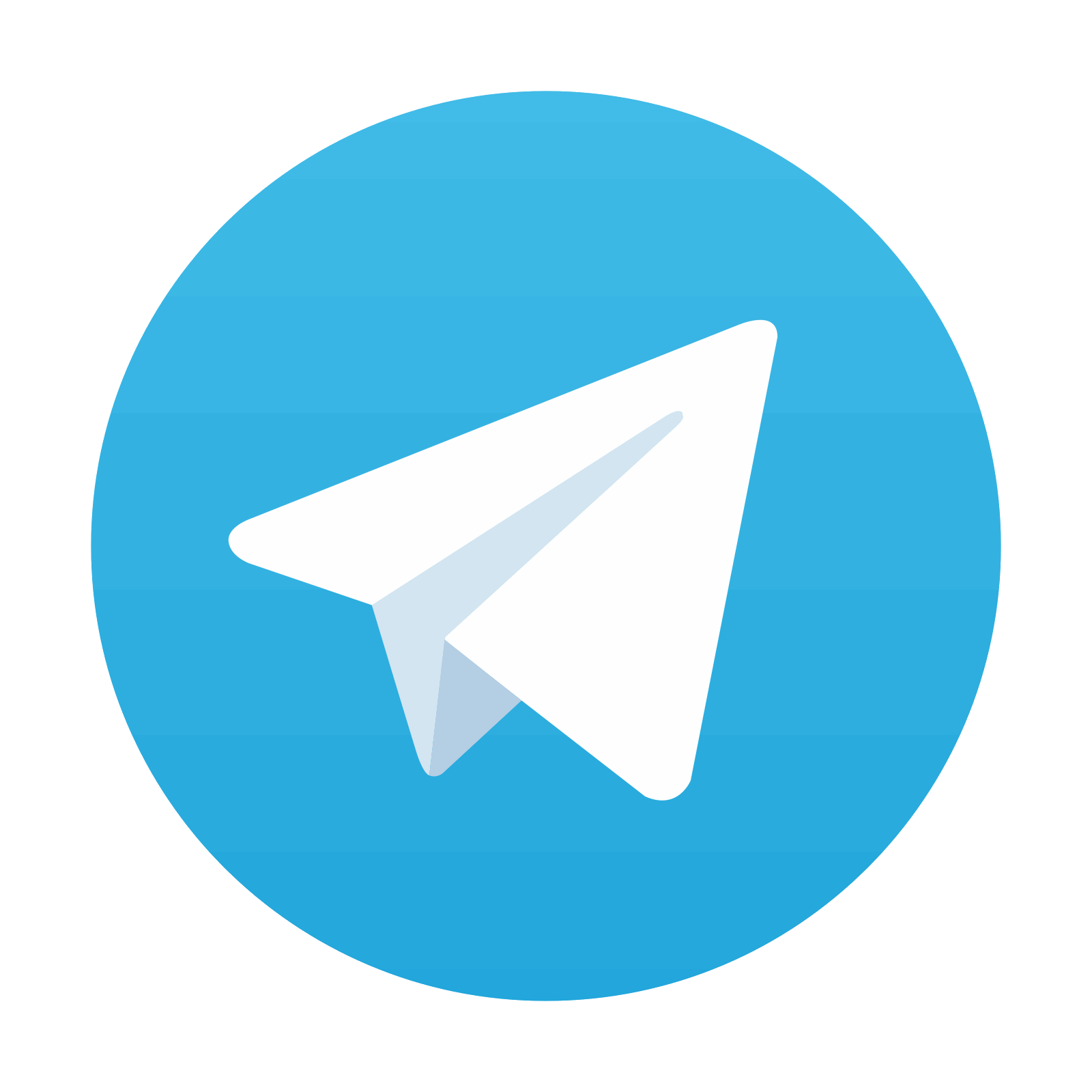
Stay updated, free articles. Join our Telegram channel

Full access? Get Clinical Tree
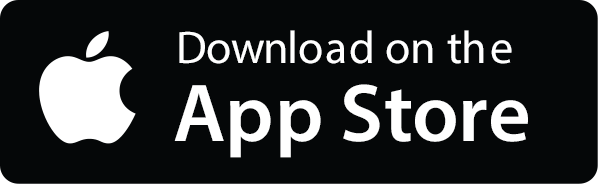
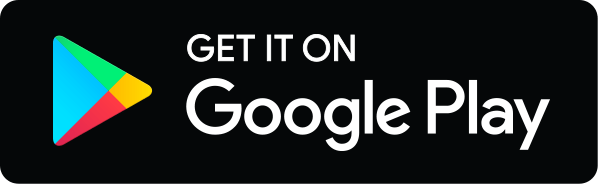