Cardiac positron emission tomography (PET) myocardial perfusion imaging is an outstanding tool for diagnosis and risk stratification of patients with known or suspected coronary artery disease with many important differences compared to SPECT imaging. Its clinical use in assessing patients for CAD as well as other nonperfusion indications is expanding rapidly. Cardiac PET offers excellent diagnostic accuracy and image quality, low radiation exposure as well as a noninvasive means of measuring myocardial blood flow reserve. Cardiovascular PET is becoming an important tool for nuclear cardiologists to consider not only for perfusion imaging, but also for a growing number of other cardiovascular conditions such as myocardial viability, infection, and inflammation. Its role in nuclear cardiology has expanded with the increased and continuous availability of PET radiopharmaceuticals and PET camera systems (see Chapters 3 and 4 on radiopharmaceuticals and instrumentation). This chapter will review the principles of cardiovascular PET, data on diagnostic accuracy, image quality, radiation exposure, indications, and addition of myocardial blood flow (MBF) for myocardial perfusion imaging (MPI), as well as exciting new nonperfusion applications.
PET imaging provides superior temporal and spatial resolution of radioactive atoms as they decay. A PET radioactive tracer, which has been engineered to be taken up in to the organ of interest, is injected into the patient. After it reaches the target organ, the radioactive agent begins to decay and emits a positron (Fig. 10-1). This positron then collides with a nearby electron. The resulting collision causes annihilation of both an electron and a positron, creating a high-energy discharge of 1.02 MeV. This energy is split into two gamma rays of 511-keV energy, which are emitted 180 degrees from each other. For image collection, multiple detectors encircle the patient; absorption from both emissions simultaneously occurs, and events that are not 180% are eliminated. The distance to the annihilation event impacts ultimate image quality. The processing of these simultaneous events forms the basis of PET imaging.1
Cardiac PET instrumentation is discussed in detail in Chapter 4. A PET camera appearance is similar to a computed axial tomography (CT) system. However, in the gantry of the PET camera are multiple detectors placed circularly around the patient (Fig. 10-2). These detectors are grouped into cassettes filled with a specified number of crystals (4,000–24,000). Data from the crystals are then transmitted to photomultiplier tubes similar to Single Photon Emission Computed Tomography (SPECT) imaging. In contrast to SPECT acquisition which requires multiple acquisitions from the same crystals rotating spatially around the patient, PET data acquisition occurs simultaneously surrounding the patient. Most scanners are full-ring design but some have partial-ring detectors with a rapidly rotating gantry.2 An attenuation correction scan with either PET radionuclide line source or computed tomography (CT) is an integral part of the acquisition and is applied to each study.
While there are several myocardial tracers available for PET imaging, only three are presently used for clinical imaging: rubidium-82 (Rb-82), N-13 ammonia (N-13), and fluorine-18 (18F) fluorodeoxyglucose (FDG). For complete review of PET isotopes, see Chapter 3.
Rubidium (Rb)-82 is a potassium analog radiopharmaceutical that is produced with commercially available generators which can be stored on site. It is produced by the decay of strontium-82 (82Sr) which has a half-life of 25.5 days. Rb-82 has a half-life of 75 seconds. The extraction fraction of Rb-82 is estimated to be 65% to 75%, substantially higher than SPECT technetium agents.
The use of Rb-82 is provided by an on-site generator and delivery system, which can be stored conveniently in the camera room. The generator is replaced every 4 to 6 weeks and supplied at a fixed cost, independent of the number of studies performed. Because of the cost involved with the generator, consideration for PET use in a facility should include adequate patient volumes. When in use, the generator is replenished quickly, within 10 minutes via 82Sr to Rb-82 decay. This permits minimal downtime between stress and rest imaging, allowing efficient utilization of the generator. The protocol for stress imaging requires the use of a pharmacologic stress agent because of the short half-life of Rb-82.
Advantages of Rb-82 include the ability to have rapid acquisition times for rest and stress imaging and continuous availability. Another advantage was studied by Parkash et al.3 They used Rb-82 with PET/CT imaging and were able to detect smaller perfusion abnormalities and more accurate detecting multivessel disease. This suggests that PET may be able to detect multivessel disease more accurately than with SPECT-imaging techniques.4
There are also disadvantages to Rb-82 imaging. Due to the half-life of 82Sr, the same generator is used for 4 to 6 weeks, but with reduced activity during the last weeks, which can impact image quality, with cameras using 2D acquisition. This limitation, however, can be overcome by 3D imaging.5 The short half-life of rubidium limits its clinical use to pharmacologic stress and not exercise.
N-13 has been used as a cardiac tracer for over 20 years. Implementation requires the presence of an on-site cyclotron as it has a half-life of 9.96 minutes. N-13 has an extraction fraction of up to 80%. The overall trapping of N-13 is dependent on a properly functioning metabolism and trapping may be decreased in myocytes that are ischemic.6,7 Advantages of N-13 ammonia include the high tomographic counts and a clear blood-to-myocardial delineation. Because of the longer half-life, exercise is possible, in contrast to rubidium. Limitations include the finding that even in normal subjects, the retention of N-13 in the lateral wall is 10% less than other segments creating false-positive results.8 Prominent liver activity in some studies can complicate interpretation of the inferior wall.9 Finally, the necessity for on-site or close proximity cyclotron availability due to short half-life limits its practical use. This has severely limited its use, with perhaps 15 to 20 laboratories using this agent according to recent data. This may change with the availability of a small cyclotron designed for ammonia production only that is much more manageable and can be placed onsite.
FDG is fluorine-labeled with 2-deoxyglucose. It is cyclotron produced and decays into O-18 oxygen. When it decays, its positron range is very short (0.5 mm). This fact, combined with its half-life of 109.8 minutes, allows it to provide images with the highest spatial resolution of any of the commonly used radionuclides.
FDG is a glucose analog and is brought into the myocardium via diffusion across glucose-specific transporters that are located on the myocytes. Once in the cell, it is transformed into FDG-6-P. There are low levels of glucose-6-phosphatase to reverse this step; there the FDG-6-P is essentially trapped in the myocardial cell. The use of F-18-FDG is further elaborated on the viability discussion later in the chapter as well as Chapter 21. Active research is underway to validate F-18 Flurpiridaz, a blood flow agent for the detection of CAD.
The increasing clinical use of cardiac PET MPI is due to several features that clinicians find important for their laboratories as well as patient care. This section will outline demonstrated advantages including imaging quality, diagnostic accuracy, radiation exposure, assessment of MBF, and conclude with a section on which patients are either recommended or preferred for PET MPI.10
Myocardial PET perfusion imaging utilizes high-energy level radiopharmaceutical tracers, with short half-lives. This translates into relatively higher doses being administered (but with lower radiation exposure) and better acquisition characteristics. The result is high myocardial counts, high spatial and contrast resolution, and high signal-to-noise ratio. Improved PET image quality was demonstrated by Yoshinaga et al., in which patients with equivocal SPECT studies were referred for cardiac PET imaging.11 In a very high percentage of patients, the PET study resulted in good to excellent image quality. Using a comparison of similar but matched patients undergoing SPECT or PET, Bateman et al. also reported a significant improvement in image quality with PET over SPECT, with fewer equivocal reports.12
The routine use of attenuation correction plus higher-energy levels of PET tracers substantially reduces attenuation artifacts in images, reducing the potential for false-positive studies for interpretation. This may be particularly useful in obese patients however, in a study by Bateman et al. the specificity of PET was significantly higher in comparison to SPECT, regardless of body habitus.12 Similarly, patients who had equivocal SPECT studies were found to have diagnostically useful PET studies in 98% of the referred patients. Thus, while obese patients or patients with prior equivocal SPECT studies are excellent candidates for PET imaging, the high accuracy among all patients suggests even broader application.10 In addition, the incidence of gut activity affecting interpretation was significantly and substantially reduced with PET in relation to pharmacologic SPECT.12
The literature suggests that PET has high sensitivity and specificity. A meta-analysis of Nandalur et al. demonstrated high diagnostic accuracy from the available PET literature in over 1400 patients (Fig. 10-3).13 Two subsequent and larger studies have confirmed these findings, including a systematic review by Mc Ardle et al. which demonstrated that the sensitivity and specificity utilizing Rb-82 was 90% and 88%, respectively.14 A separate meta-analysis of 11,862 patients by Parker et al., PET MPI, also demonstrated a higher diagnostic accuracy for coronary artery disease than SPECT MPI (Figs. 10-4 and 10-5).15 Both of these studies also compared PET to SPECT literature and found significantly higher diagnostic accuracy for PET. Many of these reported studies in these two analyses used dedicated PET cameras with line source attenuation correction. A study by Sampson et al. also confirmed high diagnostic accuracy with the PET/CT instrumentation.16
A direct clinical comparison of SPECT to PET in similar patients was performed by Bateman et al.12 Over 120 age-, body habitus-, and risk factor-matched patients underwent SPECT or PET imaging and cardiac catheterization. Significantly improved diagnostic accuracy was identified in patients undergoing PET perfusion imaging. Importantly, the ability to identify multivessel ischemia with PET was also significantly higher (74% vs. 41%) than with SPECT perfusion imaging, confirming the findings of Parkash.3 In sub-study analysis, diagnostic accuracy held regardless of body mass index or gender (Fig. 10-6).
Figure 10-4
Meta-analysis of the diagnostic accuracy of PET perfusion. (Data from Parker MW, Iskandar A, Limone B, et al. Diagnostic accuracy of cardiac positron emission tomography versus single photon emission computed tomography for coronary artery disease: A bivariate meta-analysis. Circ Cardiovasc Imaging. 2012;5:700–707.)

Figure 10-5
A systematic review and meta-analysis of PET perfusion. Panel A: PET and Panel B: SPECT. (Data from Mc Ardle BA, Dowsley TF, deKemp RA, et al. Does rubidium-82 PET have superior accuracy to SPECT perfusion imaging for the diagnosis of obstructive coronary disease? A systematic review and meta-analysis. J Am Coll Cardiol. 2012;60:1828–1837.)

Figure 10-6
Cardiac diagnostic accuracy comparing SPECT with PET. (Data from Bateman TM, Heller GV, McGhie AI, et al. Diagnostic accuracy of rest/stress ECG-gated Rb-82 myocardial perfusion PET: Comparison with ECG-gated Tc-99m sestamibi SPECT. J Nucl Cardiol. 2006;13(1):24–33.)

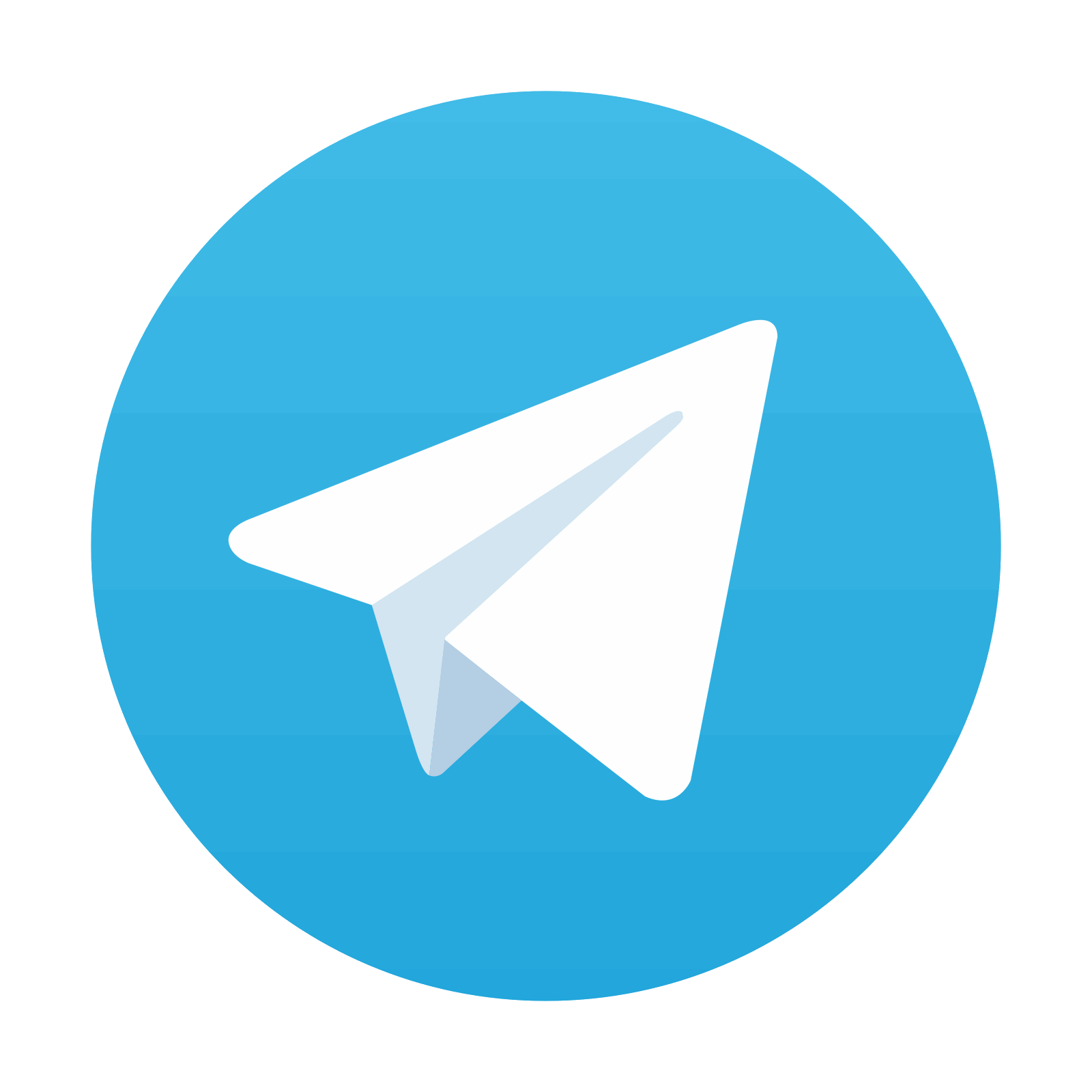
Stay updated, free articles. Join our Telegram channel

Full access? Get Clinical Tree
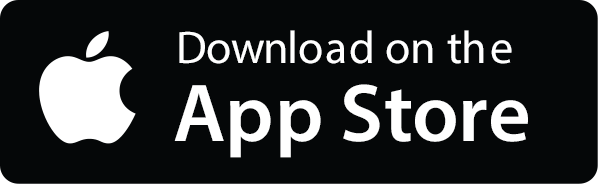
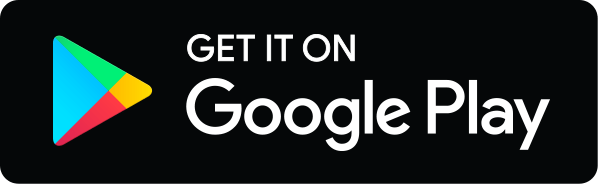
