Cardiovascular MR in Infants and Children
Mark A. Fogel
INTRODUCTION
Cardiovascular magnetic resonance (CVMR) had been applied to infants and children for nearly 30 years and is firmly established in the evaluation of anatomy, physiology, and function in congenital heart disease (CHD) (1,2,3,4,5,6,7,8,9,10 and 11). In this age range, CVMR is generally used as a complement to echocardiography and angiography; it nevertheless is a gold standard in a number of areas including ventricular volumes, mass (12,13) and vascular rings (14,15 and 16).
CVMR in infants and children utilizes nearly all the various techniques discussed in this textbook; however, it is a free standing discipline for numerous reasons, divided into a few broad categories:
Anatomy: A rigorous and systematic approach to anatomy of native CHD and of repaired CHD is very different from heart disease in the adult. Cardiac segments (atria, ventricles, and great vessels) may not be in the proper position and connections between them may be altered (as in transposition of the great arteries, where the aorta is connected to the right ventricle and the pulmonary artery is connected to the left ventricle). Venous anatomy too may not be connected properly (such as total anomalous pulmonary venous connection, where the pulmonary veins are connected to any structure other than the left atrium). Communications may present where they should not be (e.g., ventricular septal defect where there is a communication between the right and left ventricles), and some cardiac structures might not even be present or present in a markedly hypoplastic form (e.g., hypoplastic left heart syndrome, where the left ventricle is markedly hypoplastic). As surgery and catheter-based therapies play such an important role in CHD, the postinterventional anatomy must be familiar to the physician performing the CVMR examination.
Physiology/function: In the world of the infant and child with CHD, there are many times that a unique physiology is present and a result of the altered anatomy. Evaluation of shunts (e.g., in a patient with a truncus arteriosus referred to CVMR, where one great vessel arises from the heart to give rise to the systemic, pulmonary, and coronary systems) or the complex physiology of transposition of the great arteries plays a critical role in the assessment of any disease entity in pediatrics and has little parallel in the adult world. In addition, assessment of ventricular function in ventricles with strange and unusual shapes are routine in the practice of CVMR in children (e.g., L-looped ventricles which have a “left sided” right ventricle and a “right sided” left ventricle) and demand unique solutions. Assessment of the postoperative physiology where the surgeon, for example, creates an anastamosis between the cava and the pulmonary artery (when repairing a single ventricle) must be dealt with. CVMR must be adapted to fit these needs and the physician interpreting the study needs to understand the complex physiology. Even without gross anatomical alterations, function can be severely altered such as in an infant with a left ventricular aneurysm or with a dysplastic tricuspid valve (Figs. 9.1 and 9.2).
Technical challenges: Infants and children present a unique challenge in the world of CVMR; they require increased spatial resolution because of their small size and increased temporal resolution because of their high heart rates. When imaging adults, tradeoffs can be made between those two competing parameters to make imaging quicker and simpler without sacrificing diagnostic accuracy. As pulse sequences were generally designed to image adults, these tradeoffs can be performed in infants and children to a limited degree. In addition, because of the need for increased spatial resolution, signal-to-noise ratio (SNR) considerations play an important role and are always taken into account. Further, children under 7 to 9 years of age usually require sedation which can make sequences designed for breath-holding useless. Since breath-holding is the mainstay of adult CVMR, “work-arounds” have been developed to successfully image the pediatric patient. These CVMR modifications must be understood for children to be imaged properly.
Limitations of CVMR in infants and children must be understood by the imager; some to keep in mind are the following:
As alluded to above, sedation and general anesthesia for young patients must be administered to allow a child to remain motionless for a 45- to 60-minute scan; this has recently become less of an issue with the advent of the “feed and swaddle” technique which has been successfully used in infants at 6 months of age (17) (Fig. 9.1). In addition, even though they may have the cognitive ability to hold still, cooperation by the preteen or teen may be problematic (e.g., breath-holding).
As surgery and catheter-based interventions play an important role in CHD, wires, stents, coils, and clips may all cause artifacts if they are near the structure(s) of interest.
As many critically ill infant and children are treated in the intensive care unit, the lack of portability of CVMR is certainly disadvantageous; moving the patient to the CVMR suite can be a challenge.
Children with arrhythmias, especially after surgery, may not allow proper triggering while other patients may have bizarre T waves or bundle branch blocks which may not have the same effect. Although this can be problematic, it is less of an issue now that “single shot” CVMR, “real time” cine CVMR, and sequences with “arrhythmia rejection” are realities.
5. Pacemakers and ICDs are still a relative contraindication and patients with them usually do not undergo CVMR, although there is some data to suggest that this may be safe (18).
TECHNICAL CONSIDERATIONS IN CVMR OF INFANTS AND CHILDREN
As there is a need for high spatial and temporal resolution in infants and children along with the inability to breath-hold, “work-arounds” are required for CVMR sequences, which are designed for adults, to work.
SPATIAL RESOLUTION
To increase the spatial resolution, small voxels are needed; however, this can be problematic because of the poor SNR. Employing parallel imaging generally makes this even worse. Imaging without SNR considerations will result in a poorquality image which may not be diagnostic. To increase SNR, strategies have been employed either in isolation or in
combination, such as utilizing phase oversampling, decreasing the bandwidth, employing multiple averages (excitations), and avoiding techniques such as parallel imaging or partial phase Fourier. These increase imaging time but are worth it for high quality.
combination, such as utilizing phase oversampling, decreasing the bandwidth, employing multiple averages (excitations), and avoiding techniques such as parallel imaging or partial phase Fourier. These increase imaging time but are worth it for high quality.
At times, for the smallest voxels, even these approaches are not enough. In those instances, the imager must use larger voxels and this is done by either decreasing the matrix size (e.g., from 256 or 192 to 128 in the frequency encoding direction) or increasing the field of view—both of which can nevertheless yield diagnostic images yielding pixels of 1.5 to 2 mm or less. Slice thicknesses generally do not go below 2.5 to 3 mm because of SNR considerations. With gadolinium images, spatial resolution can be submillimeter (see gadolinium section).
When imaging small children, small fields of view may be needed (Fig. 9.1—a 3-day-old) and at certain settings, sequences are written that do not allow for the fields of view to decrease very low (e.g., 150 mm in a 3-kg baby). Similarly, smaller slice thicknesses than adults are needed and once again, the sequence parameters may not allow for that change at a specific setting. However, relaxing one parameter may allow another to be changed. The imager must find combinations by trial and error of changing, for example, bandwidths, TR, matrix, segments of k-space (“views” on some scanners), field of view, and slice thickness to allow for optimal imaging for the size of the patient. There are idiosyncrasies in each manufacturer’s code and it would be prohibitive to list all the combinations here. By relaxing some parameters (e.g., longer TR), other parameters may be allowed to be optimized to image the smaller patients (e.g., smaller field of view).
TEMPORAL RESOLUTION
The high heart rates in infants and children (not uncommonly, 120 to 170 beats/minute) must be taken into account for a successful CVMR scan in this age group. As for the number of phases to be acquired, as a general rule of thumb:
For an RR interval of <500 milliseconds, 18 to 20 phases should be obtained
For an RR interval of 500 to 750 milliseconds, 20 to 25 phases should be obtained
For an RR interval of 750 to 1,000 milliseconds, 25 to 30 phases should be obtained
For an RR interval of >1,000 milliseconds, 30 to 35 phases should be obtained.
To obtain this type of temporal resolution at fast heart rates, the TR needs to be low and to accomplish this, in general, the number of segments (views) needs to be low as well. This does not add very much time to the scan, since despite the small number of segments obtained per heartbeat, each heartbeat comes quicker at high heart rates relative to slow ones. If the TR and the number of segments are not low enough, in retrospectively gated imaging, the walls of the heart will appear blurry and with a double or triple shadow (does not “freeze” the motion of the wall). Adequate temporal resolution can be obtained at fast heart rates with three segments at a minimum and TRs in the teens and 20s milliseconds.
In retrospective gating, it is important to remember that the MR scanner is continuously acquiring data and recording the ECG. After all the lines of k-space are acquired, each line of k-space is then placed into “bins” in the closest phase of the cardiac cycle using interpolation. If one is performing interpolation between phases, it is important that the number of calculated phases should not exceed twice the measured phases (essentially the RR interval (milliseconds)/TR (milliseconds) where TR is the line TR × lines of k-space obtained); there should be two measured points between each interpolated point to obtain robust data. The formula used is:

where TR is defined as the line TR × lines of k-space obtained. This is especially important in velocity mapping for accurate data to be obtained.
At times, “single shot” imaging in children can be used because of respiratory motion or arrhythmia, where all the lines of k-space are acquired in one heartbeat. A typical “single shot” imaging sequence is the half fourier acquisition single shot turbo spin echo (HASTE) sequence mentioned above, but other sequences such as static steady-state free precession (SSFP) and cine SSFP can be performed in “single shot” mode; cine SSFP utilizing single shot imaging is “real time” cine. Perfusion sequences are another example of this type of imaging. If the heart rate is too fast, it is sometimes advantageous to obtain the image over two heartbeats (i.e., imaged at the end of two heartbeats [2 × RR interval]); this is commonly used in HASTE, perfusion or single shot viability imaging in children with heart rates in the triple digits.
CORONARY IMAGING
When CVMR for coronaries in small children are performed, timing is very important. A high temporal resolution fourchamber and left ventricular outflow tract cines should be obtained first and the quiescent phase of the cardiac cycle should be identified; generally, the imager focuses on the atrioventricular valve and aortic annular motion in the four-chamber and left ventricular outflow tract views, respectively. It is not uncommon to have the quiescent phase be at end-systole and not enddiastole in children. In addition, because the quiescent phase is generally short, the “shot time” for coronary imaging needs to be short (decreased number of segments are needed in general as decreasing the resolution in a small child will not work).
INABILITY OF PEDIATRIC PATIENTS TO BREATH-HOLD
To perform CVMR in patients who cannot breath-hold or remain motionless in the scanner, some institutions advocate general anesthesia in all patients; in this way the anesthesiologist can do the breath-holding. This takes a noninvasive imaging modality and makes it invasive, adds to the expense and is less physiologic than free breathing. Deep sedation with free breath during CVMR has been successfully utilized for many years in infants and children with little untoward effect (19), and in infants <6 months of age, using no medication (17). General anesthesia should be reserved for those with cardiorespiratory compromise, those who failed
deep sedation or patients who must undergo more than one examination at the same visit where deep sedation will not last for that extended period of time.
deep sedation or patients who must undergo more than one examination at the same visit where deep sedation will not last for that extended period of time.
Imaging the free-breathing infant or child with CVMR (Figs. 9.1,9.2,9.3,9.4,9.5,9.6,9.7 and 9.8) utilizes (a) multiple excitations to “average out” the respiratory motion (generally three to five excitations are needed which also increases SNR), (b) navigatorbased techniques, or (c) single shot or real time cine imaging.
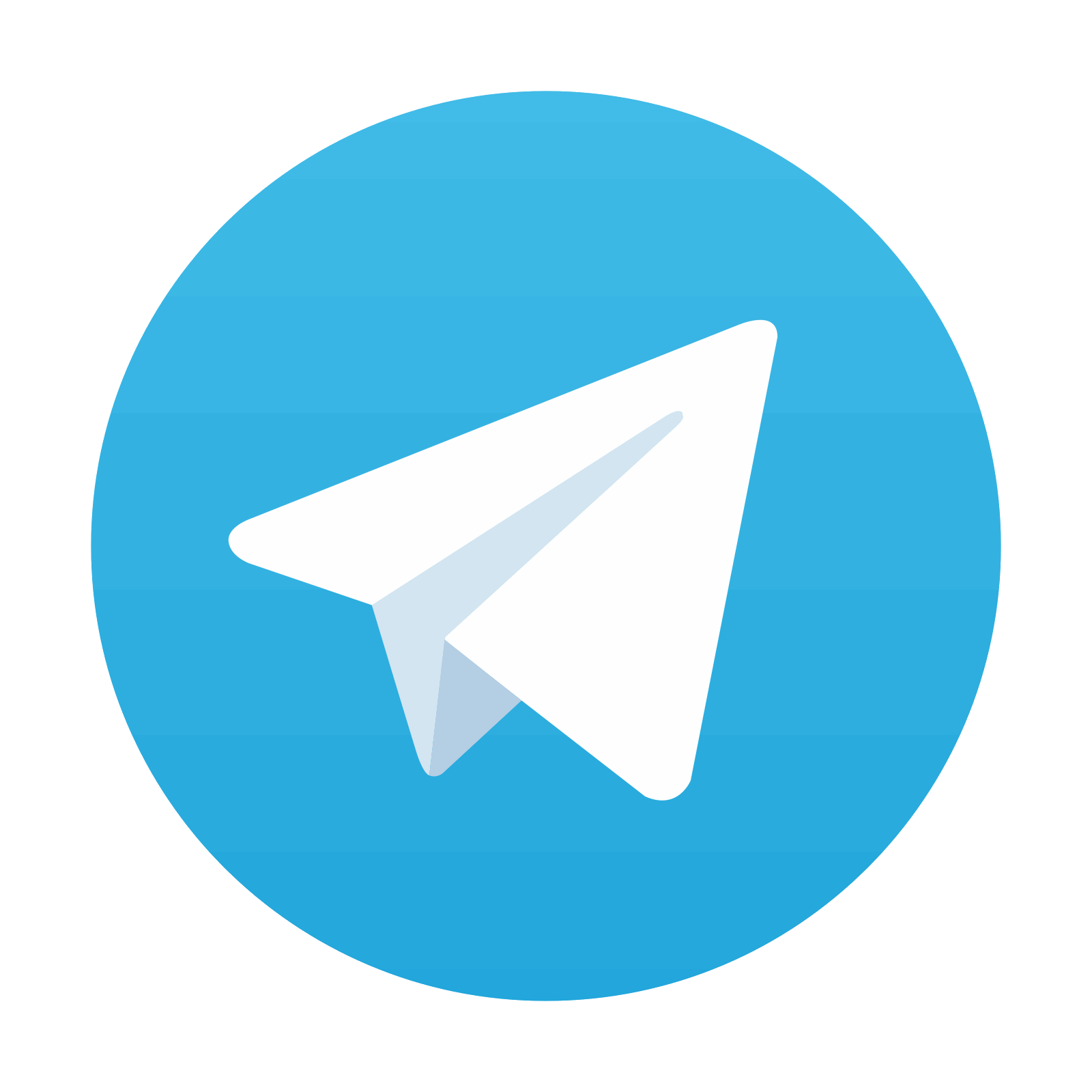
Stay updated, free articles. Join our Telegram channel

Full access? Get Clinical Tree
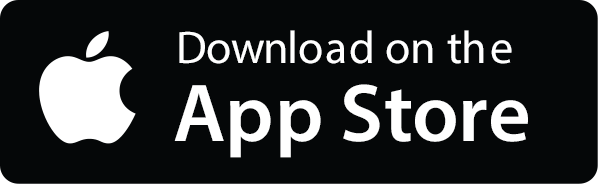
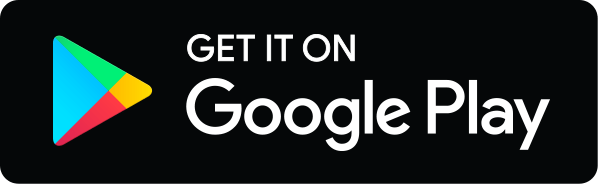