Abstract
Background
In an open-chest model of acute infarct, epicardial delivery of hepatocyte growth factor (pCK-HGF-X7) gene improved left ventricle (LV) function. This study was designed to test (a) the efficacy of HGF gene in infarct scar delivered under magnetic resonance (MR) guidance and (b) the potential of multiple MR sequences in assessing the effects of pCK-HGF-X7 (treatment) and pCK-LacZ (control) genes on myocardial structure and function.
Materials and methods
Swine (six per group) were subjected to myocardial infarct, under X-ray fluoroscopy, and developed LV remodeling at 5 weeks. Multiple clinical magnetic resonance (MR) imaging sequences were performed before delivery of gene (at 5 weeks after infarction) and 5 weeks after delivery of gene. Under MR guidance, the active endovascular catheter was introduced into LV to transendocardially deliver 3.96×10 11 viral copies of pCK-HGF-X7 or pCK-LacZ in the border and core of the infarct scar. Histological evaluation of the infarct scar was performed 5 weeks after delivery of gene.
Results
At 5 weeks after infarction, there was no significant difference in measured cardiovascular MR parameters between the groups. The pCK-HGF-X7 gene caused significant improvement in the following parameters ( P <.05 for these parameters): three-dimensional (3D) strain (radial, circumferential, and longitudinal) and perfusion (maximum upslope, peak signal intensity, and time to peak) compared with control pCK-LacZ at 5 weeks after delivery of the genes. The ejection fraction was higher in pCK-HGF-X7-treated (43±1%) than in pCK-LacZ control (37±1%, P <.05) animals. These changes are associated with a decrease in infarct scar size (11.3±2.0% in pCK-LacZ control and 6.7±1.3% in pCK-HGF-X7-treated animals, P <.01) and infarct transmurality in four out of five infarct scar segments ( P <.05) on delayed contrast-enhanced MR imaging. Microscopic study confirmed the increase in capillary ( P <.05) and arteriole ( P <.05) density of infarct scar in pCK-HGF-X7-treated animals compared with pCK-LacZ control animals.
Conclusions
Hepatocyte growth factor gene (pCK-HGF-X7) delivered under MR guidance into infarct scar ameliorated global function and 3D strain, increased regional perfusion and infarct resorption, and enhanced angiogenesis/arteriogenesis. This feasibility study provides novel approach and analysis methods and instrumentation for delivering and evaluating new locally delivered therapies.
1
Introduction
Congestive heart failure has become a widespread public health concern; there are approximately 5 million patients in the United States . Following infarction, the left ventricle (LV) undergoes structural remodeling, resulting in functional deterioration . Despite significant advances in pharmaceutical, surgical, and interventional therapies, positive results in angiogenesis and cardiac repair are limited. Growing evidence from preclinical and clinical studies suggests that gene therapy has the potential to enhance angiogenesis and improve LV function. Assessment of LV function after gene therapy has hinged on invasive analysis, and most of the focus was on the cellular and molecular changes . For example, investigators established the fundamental mechanisms of action of hepatocyte growth factor (HGF) that include angiogenesis, arteriogenesis, myogenesis, reduction of collagen deposition, and apoptosis .
Our group and others demonstrated in an open-chest model the improved LV function and perfusion at 8 weeks after delivering the plasmid pCK-HGF-X7 gene into the epicardium of acute infarct . More recently, a minimally invasive approach under MR guidance has been introduced for delivering plasmid vascular endothelial growth factor (VEGF) gene in acute infarct . In routine clinical settings, a minimally invasive approach is critical for rapid recovery, reduction of morbidity and mortality, and cost savings to the health care system. To our knowledge, the efficacy of pCK-HGF-X7 and pCK-LacZ genes delivered transendocardially into the border and core of infarct scar, under MR guidance, has not been demonstrated. Therefore, this study was designed to test (a) the efficacy of the effects of plasmid pCK-HGF-X7 and pCK-LacZ genes on infarct scar delivered under MR guidance and (b) the potential of multiple MR sequences in assessing the effects of these genes on myocardial structure and function.
2
Materials and methods
2.1
Therapeutic gene and delivery system
The plasmid pCK-HGF-X7 gene (ViroMed, Seoul, South Korea), was used as a therapeutic agent, and pCK-β-galactosidase (LacZ) gene was used as a control. The components of pCK-HGF-X7 gene and their functions have been previously described .
Six steerable, deflectable guiding catheters with a retractable needle were used for transendocardial delivery of the genes (SurgiVision, Baltimore, MD) . The endovascular catheter had a nitinol needle for puncturing the border and core of the infarct scar. The catheters were fitted with multiple MR radiofrequency receiver coils and were actively tracked to the target (infarct scar) under MR imaging ( Fig. 1 ).

2.2
Experimental protocol
The study conformed to the Guide for the Care and Use of Laboratory Animals (NIH Publication No. 85-23, revised 1996), and approval was obtained from Institutional Animal Care and Use Committee (IACUC). Pigs ( n =18, 30–32 kg) were premedicated using acepromazine/ketamine and anesthetized using isoflurane/oxygen. A percutaneous balloon catheter (Boston Scientific, Natick, MA) was advanced distal to the second diagonal branch under X-ray guidance, and the artery was occluded for 90 min followed by reperfusion . Coronary angiograms were repeatedly acquired to ensure complete occlusion/reperfusion.
A total of 18 animals were studied, of which two animals died during the coronary occlusion/reperfusion and four died during the navigation of the endovascular catheter in LV at 5 weeks after infarction. The remaining animals were divided in a blinded fashion into two groups: the pCK-HGF-X7-gene-treated group ( n =6) and the pCK-LacZ control group ( n =6). These 12 animals completed the study and follow-up. The specially designed endovascular catheter was used to deliver 3.96×10 11 viral copies of the pCK-HGF-X7 (therapy, n =6) or pCK-LacZ (control, n =6). The number of injected viral copies in the border and core of infarct scar was 50% greater compared to those injected in acute infarct, and the genes were delivered over a larger infarct area by doubling the number of injection sites from four to eight (0.3–0.4 ml/site) at a rate of ∼20 s/site . All procedures were performed under sterile conditions. All animals received lidocaine (3.3 mg/kg iv, during occlusion/reperfusion and gene injection) and trimethyl sulfate (15 mg/kg po, for 3 days after intervention).
Noninvasive clinical MR imaging sequences were performed at 5 weeks after infarction before delivery of gene and 5 weeks after delivery of the genes. The first MR imaging session was performed to establish a baseline. Magnetic resonance contrast media were delivered via the left ear vein for first-pass perfusion of gadolinium diethylenetriaminopentaacetic acid (0.1 mmol/kg Gd-DTPA, 3 ml/s) using power injector. An additional dose of 0.05 mmol/kg Gd-DTPA was delivered for delayed contrast-enhanced MR imaging (DE-MR imaging).
2.3
Cardiovascular MR imaging
The hybrid XMR suite (Philips, Best, the Netherlands) featuring an X-ray catheterization laboratory and a 1.5-T short-bore cardiovascular MR scanner with an in-room display monitor and console for viewing and interactive scanning enables LV catheterization under real-time imaging and for delivery of gene and its efficacy in infarct scar. Multiple MR sequences were simultaneously used in each session:
- 1.
cine MR imaging for measuring LV volumes, cardiac output, stroke volume, mass, wall thickness, and percent systolic wall thickening (radial strain),
- 2.
tagged MR imaging for measuring circumferential strain,
- 3.
phase-contrast velocity-encoded MR imaging for measuring longitudinal strain,
- 4.
first-pass perfusion MR imaging for measuring regional myocardial perfusion,
- 5.
DE-MR imaging for measuring infarct size and infarct transmurality, and
- 6.
MR fluoroscopy for navigating the endovascular active catheter to the target.
2.4
Image analysis
Segment software was used by investigators blinded to treatment to determine LV volumes, ejection fraction, mass, perfusion, infarct size, and transmurality and for measuring longitudinal and systolic wall thickening (radial strain) . Regional wall thickness at end systole and end diastole of infarct scar (the target) and remote segments (LV free wall) were obtained, using DE-MR imaging as guidance. A 0° starting point at the posteroseptal groove was used in the above parameters for each individual slice in the set (clockwise direction). The software calculated the mean centerline of 8 LV segments (45° per segment). Percent systolic thickening at all time points were determined on three slices (eight segments per slice). A total of 576 segments was measured for segmental systolic wall thickening covering 5 weeks after infarction and 5 weeks after delivery of gene time points using Segment software, while HARP software was used blinded to treatment for measurement of peak systolic circumferential strain . For image registration of the circumferential strain, the LV and the anteroseptal groove were used as the anatomic landmark . The radial, circumferential, and longitudinal strain were assessed in the same apical slices containing the infarct, shown on DE-MR images. Automatic signal intensity threshold ±2 S.D. above remote myocardium was used to define the infarct and infarct transmurality .
2.5
Histopathology
After the last imaging session at 5 weeks after delivery of genes, the animals were euthanized and the freshly excised hearts were sliced and stained with triphenyltetrazolium chloride (TTC) to delineate the infarct scar. Magnetic resonance images were registered with TTC slices in both groups. Large tissue samples that include infarct scar (the target) and remote myocardium (free LV wall) were obtained. Following fixation and paraffin embedding, 5-μm-thick tissue sections were stained with hematoxylin and eosin, Masson trichrome, and biotinylated Bandeira simplicifolia isolectin B 4 (Vector Lab, Burlingame, CA) . Standard immunoperoxidase method and biotinylated isolectin B 4 localized vascular endothelial cells. Vascular density and myocyte diameter were determined in a blinded fashion using previously described methods .
2.6
Statistical analysis
Differences in data obtained at 5 weeks after infarction and 5 weeks after delivery of gene in both pCK-HGF-gene-treated and pCK-LacZ control groups were compared using one-way analysis of variance (ANOVA) for repeated measurements followed by post hoc test when an overall significance was detected. The following parameters were compared using two-way ANOVA, followed by a Scheffe post hoc analysis: (a) body weight, heart rate, and arterial blood pressure; (b) LV function [ejection fraction, LV volumes, stroke volumes, cardiac output, three-dimensional (3D) strain]; (c) first-pass perfusion parameters (maximum upslope, peak signal intensity, and time to peak); (d) infarct transmurality and size; and (e) capillary and arteriole densities as well as myocyte diameters. Data were expressed as mean±S.E.M. P <.05 was considered statistically significant.
2
Materials and methods
2.1
Therapeutic gene and delivery system
The plasmid pCK-HGF-X7 gene (ViroMed, Seoul, South Korea), was used as a therapeutic agent, and pCK-β-galactosidase (LacZ) gene was used as a control. The components of pCK-HGF-X7 gene and their functions have been previously described .
Six steerable, deflectable guiding catheters with a retractable needle were used for transendocardial delivery of the genes (SurgiVision, Baltimore, MD) . The endovascular catheter had a nitinol needle for puncturing the border and core of the infarct scar. The catheters were fitted with multiple MR radiofrequency receiver coils and were actively tracked to the target (infarct scar) under MR imaging ( Fig. 1 ).
2.2
Experimental protocol
The study conformed to the Guide for the Care and Use of Laboratory Animals (NIH Publication No. 85-23, revised 1996), and approval was obtained from Institutional Animal Care and Use Committee (IACUC). Pigs ( n =18, 30–32 kg) were premedicated using acepromazine/ketamine and anesthetized using isoflurane/oxygen. A percutaneous balloon catheter (Boston Scientific, Natick, MA) was advanced distal to the second diagonal branch under X-ray guidance, and the artery was occluded for 90 min followed by reperfusion . Coronary angiograms were repeatedly acquired to ensure complete occlusion/reperfusion.
A total of 18 animals were studied, of which two animals died during the coronary occlusion/reperfusion and four died during the navigation of the endovascular catheter in LV at 5 weeks after infarction. The remaining animals were divided in a blinded fashion into two groups: the pCK-HGF-X7-gene-treated group ( n =6) and the pCK-LacZ control group ( n =6). These 12 animals completed the study and follow-up. The specially designed endovascular catheter was used to deliver 3.96×10 11 viral copies of the pCK-HGF-X7 (therapy, n =6) or pCK-LacZ (control, n =6). The number of injected viral copies in the border and core of infarct scar was 50% greater compared to those injected in acute infarct, and the genes were delivered over a larger infarct area by doubling the number of injection sites from four to eight (0.3–0.4 ml/site) at a rate of ∼20 s/site . All procedures were performed under sterile conditions. All animals received lidocaine (3.3 mg/kg iv, during occlusion/reperfusion and gene injection) and trimethyl sulfate (15 mg/kg po, for 3 days after intervention).
Noninvasive clinical MR imaging sequences were performed at 5 weeks after infarction before delivery of gene and 5 weeks after delivery of the genes. The first MR imaging session was performed to establish a baseline. Magnetic resonance contrast media were delivered via the left ear vein for first-pass perfusion of gadolinium diethylenetriaminopentaacetic acid (0.1 mmol/kg Gd-DTPA, 3 ml/s) using power injector. An additional dose of 0.05 mmol/kg Gd-DTPA was delivered for delayed contrast-enhanced MR imaging (DE-MR imaging).
2.3
Cardiovascular MR imaging
The hybrid XMR suite (Philips, Best, the Netherlands) featuring an X-ray catheterization laboratory and a 1.5-T short-bore cardiovascular MR scanner with an in-room display monitor and console for viewing and interactive scanning enables LV catheterization under real-time imaging and for delivery of gene and its efficacy in infarct scar. Multiple MR sequences were simultaneously used in each session:
- 1.
cine MR imaging for measuring LV volumes, cardiac output, stroke volume, mass, wall thickness, and percent systolic wall thickening (radial strain),
- 2.
tagged MR imaging for measuring circumferential strain,
- 3.
phase-contrast velocity-encoded MR imaging for measuring longitudinal strain,
- 4.
first-pass perfusion MR imaging for measuring regional myocardial perfusion,
- 5.
DE-MR imaging for measuring infarct size and infarct transmurality, and
- 6.
MR fluoroscopy for navigating the endovascular active catheter to the target.
2.4
Image analysis
Segment software was used by investigators blinded to treatment to determine LV volumes, ejection fraction, mass, perfusion, infarct size, and transmurality and for measuring longitudinal and systolic wall thickening (radial strain) . Regional wall thickness at end systole and end diastole of infarct scar (the target) and remote segments (LV free wall) were obtained, using DE-MR imaging as guidance. A 0° starting point at the posteroseptal groove was used in the above parameters for each individual slice in the set (clockwise direction). The software calculated the mean centerline of 8 LV segments (45° per segment). Percent systolic thickening at all time points were determined on three slices (eight segments per slice). A total of 576 segments was measured for segmental systolic wall thickening covering 5 weeks after infarction and 5 weeks after delivery of gene time points using Segment software, while HARP software was used blinded to treatment for measurement of peak systolic circumferential strain . For image registration of the circumferential strain, the LV and the anteroseptal groove were used as the anatomic landmark . The radial, circumferential, and longitudinal strain were assessed in the same apical slices containing the infarct, shown on DE-MR images. Automatic signal intensity threshold ±2 S.D. above remote myocardium was used to define the infarct and infarct transmurality .
2.5
Histopathology
After the last imaging session at 5 weeks after delivery of genes, the animals were euthanized and the freshly excised hearts were sliced and stained with triphenyltetrazolium chloride (TTC) to delineate the infarct scar. Magnetic resonance images were registered with TTC slices in both groups. Large tissue samples that include infarct scar (the target) and remote myocardium (free LV wall) were obtained. Following fixation and paraffin embedding, 5-μm-thick tissue sections were stained with hematoxylin and eosin, Masson trichrome, and biotinylated Bandeira simplicifolia isolectin B 4 (Vector Lab, Burlingame, CA) . Standard immunoperoxidase method and biotinylated isolectin B 4 localized vascular endothelial cells. Vascular density and myocyte diameter were determined in a blinded fashion using previously described methods .
2.6
Statistical analysis
Differences in data obtained at 5 weeks after infarction and 5 weeks after delivery of gene in both pCK-HGF-gene-treated and pCK-LacZ control groups were compared using one-way analysis of variance (ANOVA) for repeated measurements followed by post hoc test when an overall significance was detected. The following parameters were compared using two-way ANOVA, followed by a Scheffe post hoc analysis: (a) body weight, heart rate, and arterial blood pressure; (b) LV function [ejection fraction, LV volumes, stroke volumes, cardiac output, three-dimensional (3D) strain]; (c) first-pass perfusion parameters (maximum upslope, peak signal intensity, and time to peak); (d) infarct transmurality and size; and (e) capillary and arteriole densities as well as myocyte diameters. Data were expressed as mean±S.E.M. P <.05 was considered statistically significant.
3
Results
3.1
Cardiac interventions
Cardiac interventions were performed under (a) X-ray for coronary artery occlusion/reperfusion and (b) MR guidance for transendocardial delivery of gene. Animals ( n =16) showed myocardial infarct 5 weeks prior to delivery of gene and ( n =12) 5 weeks after delivery of gene on DE-MR imaging. The pCK-HGF-X7 and pCK-LacZ animals showed no significant difference in body weight, heart rate, and mean arterial blood pressure at any time point ( Table 1 ). The advancement of the endovascular active catheter from the aorta into the LV chamber and then to the target is shown in Fig. 1 . The brightness of the endovascular catheter in the dark background LV chamber facilitates the navigation of the catheter to the target. The injection was performed after turning off the active coils to eliminate the heating produced by coil activation.
Parameters | 5 weeks after infarction | 5 weeks after therapy | ||
---|---|---|---|---|
pCK-LacZ | pCK-HGF-X7 | pCK-LacZ | pCK-HGF-X7 | |
Body weight (kg) | 43±2 | 41±1 | 54±1 | 52±1 |
Heart rate (bpm) | 92±3 | 98±7 | 86±3 | 88±3 |
Mean blood pressure (mmHg) | 69±5 | 67±4 | 70±4 | 69±3 |
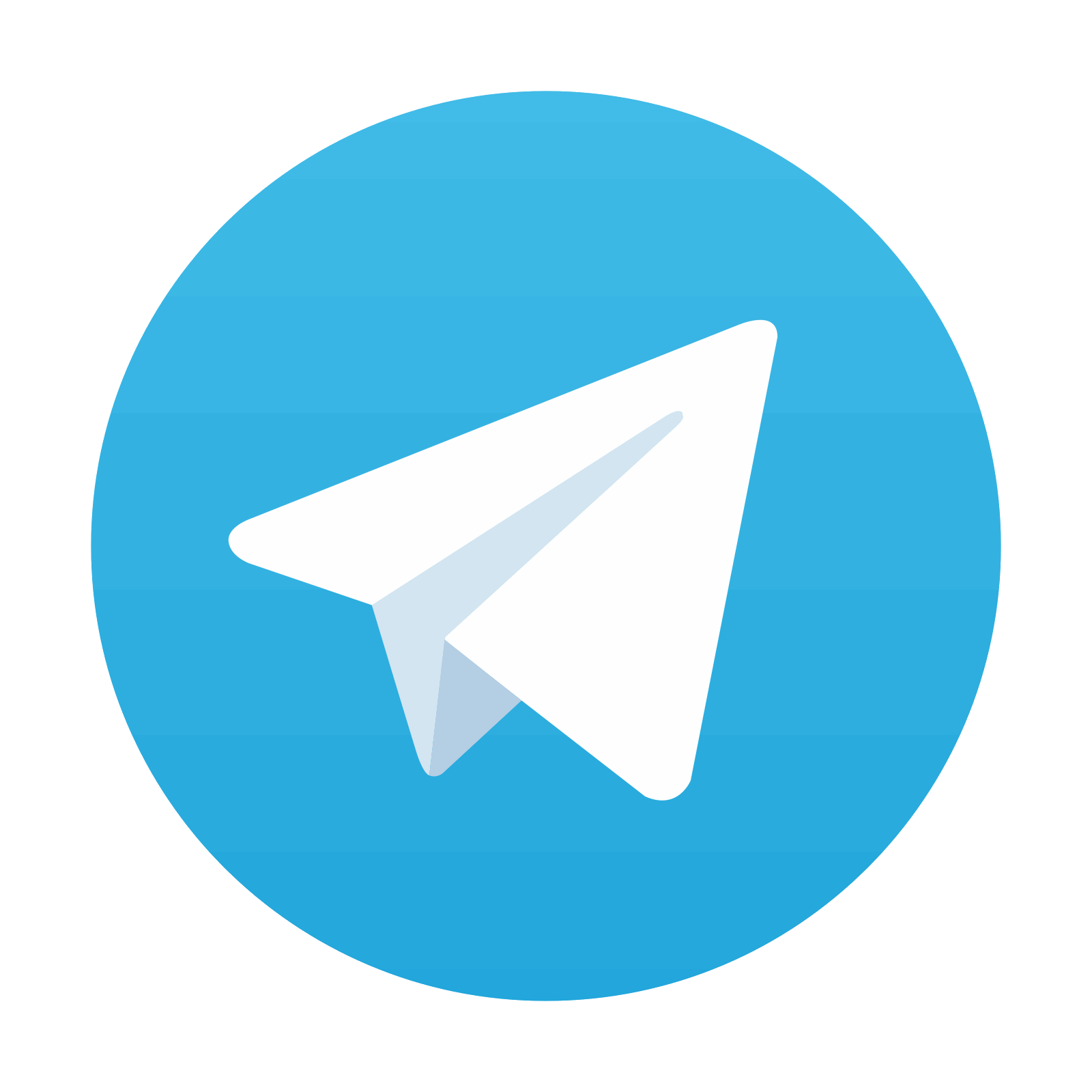
Stay updated, free articles. Join our Telegram channel

Full access? Get Clinical Tree
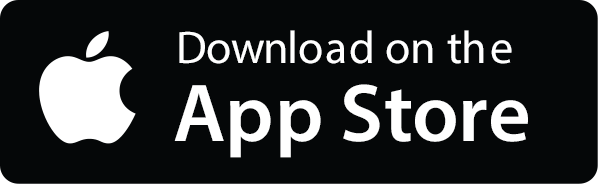
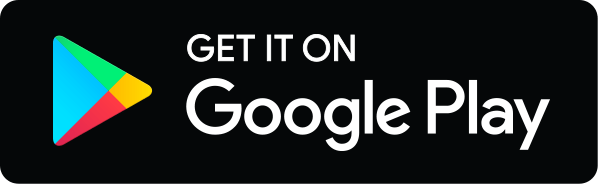
