Cardiovascular Magnetic Resonance Imaging
Andrew O. Zurick III, MD, FACC, FASE, FSCMR
Cardiac Magnetic Resonance Imaging
Cardiac magnetic resonance imaging (CMR) is a robust noninvasive imaging technique. Through electromagnetic manipulation of biologic hydrogen protons, CMR can provide assessment of cardiac structure, function, perfusion, tissue characterization, blood flow velocity, cardiac masses, valvular heart disease, pericardial disease, and vascular disease. Continued improvements in hardware and pulse sequence design have allowed for improved image quality, speed of data acquisition, and reliability, further increasing the usefulness of CMR for clinical applications. CMR is similar to echocardiography in that neither utilizes ionizing radiation to acquire high-resolution images, avoiding the exposures inherent in invasive coronary angiography, computed tomography, and single photon emission tomography imaging. CMR is capable of assessing cardiac morphology in any number of x, y, and z axis orientations. In addition, the large field of view in CMR imaging allows assessment of both cardiac and noncardiac pathologies.
Technology of CMR
Magnetic resonance imaging (MRI) (including CMR) is based on the electromagnetic manipulation of biologic hydrogen protons. Hydrogen is the most abundant element present within the human body, present within all tissues, whether in water, adipose tissue, or soft tissue. Each water molecule contains two hydrogen nuclei, each with a single proton, and they behave like tiny magnets. Proton spins can be aligned by application of a powerful magnetic field in the β(0) direction, given the appropriate frequency via the Larmor equation (f = γβ; where f is the precessional frequency, β is the magnet field strength, and γ is the gyromagnetic ratio). A second radiofrequency electromagnetic field can then be briefly applied and then rapidly discontinued. As protons return to their original alignment after the electromagnetic field is turned off (“relaxation”), they generate a net magnetization that decays to its former position with energy loss in the form of a radio signal that can be detected with a radiofrequency antenna and quantified. Image tissue contrast depends on differences in the decay of net magnetization in the longitudinal plane (T1) and transverse plane (T2). Through the application of additional electromagnetic fields (gradient fields), radio waves coming from the body can be spatially encoded, allowing localization within an imaging plane.
Data Acquisition Sequences and Techniques
CMR utilizes two basic imaging sequences: spin echo (“dark blood”) and gradient echo (“bright blood”). Spin echo sequences are commonly used for multislice anatomic imaging, providing clear delineation of the mediastinum, cardiac chambers, and great vessels. Alternatively, gradient echo sequences are used more often for physiologic assessment of function through cine acquisitions. Because of higher possible imaging speeds, gradient echo is more appropriately used for ventricular function and myocardial perfusion assessment and valvular assessment. Phase contrast imaging (PCI) allows quantitative flow velocity and volume flow assessment. All cardiac and most vascular CMR sequences require cardiac electrocardiogram (ECG)-gating. Through data acquisition of segments at different phases of the cardiac cycle, a cine image loop can be created tracking cardiac motion. Perfusion imaging, through the use of intravenous contrast agents, permits assessment of tissue vascularity. In the case of vasodilator stress perfusion imaging, assessment of myocardial ischemia is possible (Figure 18.1). Inotropic stress imaging, typically with intravenous dobutamine, allows assessment of new regional wall motion abnormalities. Predominantly gadolinium-based contrast agents, chelated to other nontoxic molecules for clinical use, are utilized for imaging the cardiovascular system.
Clinical Indications
VENTRICULAR FUNCTION
CMR is highly accurate and reproducible, providing clinically useful measurements of cardiac wall thickness, chamber volumes, myocardial mass, and systolic contractile function. CMR is recognized as the “gold standard” for assessment of left and right ventricular function. Left ventricular ejection fraction, left ventricular end-diastolic volume, left ventricular end-systolic volume, stroke volume, cardiac output, and left ventricular mass can all be reliably quantified. Left ventricular diastolic function can also be reliably interrogated using PCI.
ISCHEMIC HEART DISEASE
CMR is the most sensitive cardiac imaging modality for the assessment of myocardial viability and the extent of myocardial infarction. It is the imaging modality of choice for patients in whom there is a question about whether the distribution of a planned revascularization is viable or not. For this application, compared with nuclear imaging, CMR is much more sensitive in detecting subendocardial viability (and lack of viability) and, obviously, CMR does not require radiation exposure for patients. Gadolinium is excluded from intact myocardial cell membranes and thus is useful in defining areas of infarction. Correlation with anatomic specimens suggests a sensitivity and specificity above 95%. Delayed hyperenhancement (DHE) protocols, most often using phase sensitive inversion recovery imaging, are based on the high signal intensity (“bright”) that results from T1 time shortening due to gadolinium contrast localization within scar tissue (Figure 18.2). Alternatively, first-pass perfusion images that appear hypointense are probably a combination of ischemic and infarcted tissues. The highest likelihood of recovery of contractility impairment exists when the transmural infarction extent, as assessed by DHE, is less than 50% transmural.1 Additionally, anomalous coronary arteries can be identified through the use of CMR. In particular, CMR is well suited to demonstrate the relationship of anomalous coronary arteries to other vascular structures (the aorta and main pulmonary artery) and thus to make decisions on the need and timing of surgery.
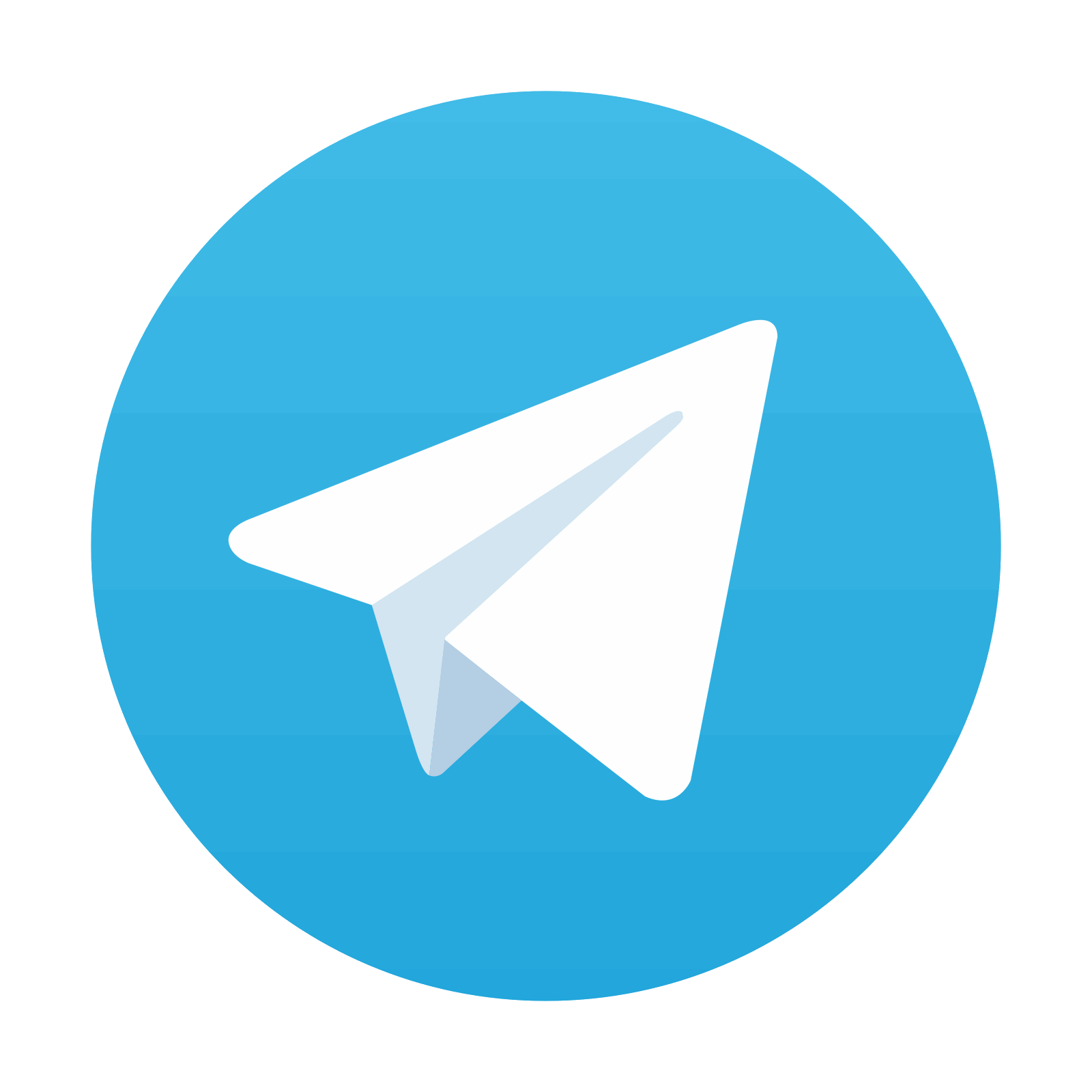
Stay updated, free articles. Join our Telegram channel

Full access? Get Clinical Tree
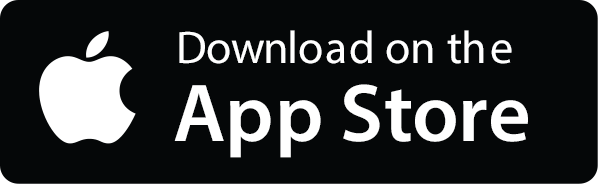
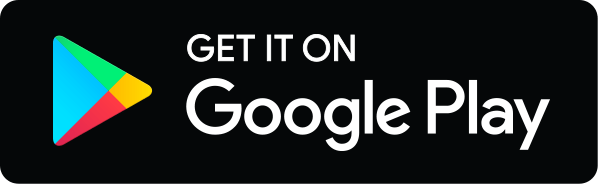
