Cardiovascular Computed Tomography
Parag R. Patel
Milind Desai
I. INTRODUCTION.
Cardiovascular computed tomography (CT) has continued to rapidly evolve over the past decade, gaining new and expanded indications for noninvasive assessment of the heart, great vessels, and peripheral vasculature. Technological improvements, including increasing numbers of detectors, improved temporal and spatial resolution, and advanced postprocessing, have broadened the clinical utility of this imaging modality. Advanced multidetector computed tomography (MDCT) scanners and new scanning protocols have significantly reduced radiation and contrast dosages. Numerous considerations are involved in the proper selection of cardiovascular CT protocols, and skilled operators are required to plan and interpret these examinations.
II. BASICS OF CARDIAC CT
A. CT physics.
In CT, images are created by rotating an x-ray source emitting a fanshaped beam of x-rays, which then pass through the body. Some x-rays are absorbed or scattered, but others are transmitted and subsequently sensed by detectors located directly across the x-ray source. In MDCT, the x-ray tube and detectors are mounted on a gantry that rotates rapidly around the patient as he or she passes through the scanner. As in traditional x-ray radiography, different structures attenuate the x-ray beam to differing extents depending on their atomic composition and density, as well as the energy of the incident photons. The data collected by the detectors then go through a complex set of mathematical reconstruction algorithms that create a set of axial images through the technique of backprojection. Each voxel in the resulting axial image is ascribed a specific attenuation value, which is expressed in Hounsfield units (H.U.). Using a reference of 0 H.U. for water, −1000 H.U. for air, and +1000 H.U. for bony cortex, different points are assigned their respective attenuation values. This information is then converted into a grayscale image that can be manipulated by the interpreting physician.
B. Technical challenges for cardiac imaging
1. The fast cyclical motion of the heart requires high temporal resolution to avoid blurring or degradation of images due to cardiac motion artifact. In cardiac CT, image acquisition is referenced, or gated, to the cardiac cycle. Although data can be acquired throughout the cardiac cycle, most image data sets are reconstructed during periods of minimal cardiac motion, typically a brief 100- to 300-millisecond interval in late diastole (60% to 75% of the R-R interval).
2. High spatial resolution is required to image relatively small vessels such as the coronary arteries. Current MDCT scanners (64-slice) provide a spatial resolution of 0.4 mm compared with approximately 0.2 mm for invasive angiography, the gold standard.
3. Respiratory motion artifact can be minimized by having the patient hold his/her breath during image acquisition. Most clinically available scanners can cover the entire heart in 10 to 12 seconds, whereas the newest 256-slice MDCT scanners can cover this area in just one or two heartbeats.
4. Rapid improvements in CT technology and protocoling have outpaced research in the field. Many studies investigating the diagnostic and prognostic yield of information gained from cardiac CT are not based on the newest MDCT scanners—but rather on single-beam/detector systems. Further investigation involving randomized controlled studies has been limited by the ethical issue raised by radiation exposure, although this has also been significantly reduced by innovative scanning approaches.
C. Current CT hardware
1. MDCT involves using an x-ray tube mounted opposite multiple detector rows on a gantry, which is then rotated around the patient at a rapid rate (220 to 400 ms/rotation). The patient is moved at either a fixed or variable speed, or pitch, through the scanner. An increasing number of detectors allows for an increased z-axis (cranial-caudal) coverage, permitting faster scans with improved image quality due to less cardiac and respiratory motion artifact. Temporal resolution is improved by faster gantry rotation, the use of two x-ray tubes and detector arrays mounted at 90° angles to each other (dual-source MDCT), and special reconstruction techniques. Dual-source/dual-energy scanners provide substantial improvements by utilizing dual-source MDCT technology as well as dual-energy sources to improve temporal resolution and decrease scatter. The fastest scanners provide a temporal resolution of 83 to 105 milliseconds. Spatial resolution is largely determined by detector architecture (typically 0.4 mm isotropic resolution), although thicker slices (1 to 5 mm) can be acquired to reduce radiation dose according to the study indication. MDCT can be used for both
cardiac and noncardiac studies, and it is now the most widely used type of CT hardware for cardiac imaging.
cardiac and noncardiac studies, and it is now the most widely used type of CT hardware for cardiac imaging.
2. Electron beam computed tomography (EBCT), although rarely used today, was specifically developed for cardiac imaging. It involves the use of a rapidly oscillating electron beam reflected onto a stationary tungsten target. Because there is no mechanical motion within the gantry, EBCT is capable of very high temporal resolution (50 to 100 milliseconds). EBCT was used primarily for the quantitative detection of coronary artery calcification (CAC).
D. Image acquisition techniques
1. Acquisition modes.
Most current MDCT scanners use spiral retrospectively gated acquisition techniques for cardiac imaging, as this mode provides the greatest flexibility in image selection during different phases of the cardiac cycle and the ability to edit the image data set for artifacts due to ectopic beats. Recently introduced software has made the older prospectively gated axial acquisition mode possible for cardiac imaging in selected patients, and this has resulted in a 60% to 70% reduction in radiation dose.
a. Sequential (axial, “step-and-shoot”) mode. Single transaxial slices are sequentially acquired while the patient table is incrementally advanced between successive rotations of the gantry.
b. Spiral (helical) mode. Data are continuously acquired during constant rotation of the gantry with simultaneous, constant (z-axis) movement of the patient through the scanner. As the tube does not perform a complete rotation in any plane, x-ray data are interpolated from a series of sequential frames to create a single tomographic image.
2. Electrocardiogram (ECG) gating
a. Prospective triggering. The trigger signal is derived from the patient’s ECG based on a prospective estimation of the R-R interval. The scan is usually triggered to begin at a defined point after the R wave, usually allowing image acquisition to occur during diastole. Prospective ECG triggering is one of the most dose-efficient ways of cardiac scanning, as only the very minimum scan data needed for image reconstruction are acquired. Limitations of prospective triggering (or “gating”) include the fact that the acquired data set will be of a limited portion (or phase) of the cardiac cycle only, limiting the opportunity for evaluating image data sets from other cardiac phases. In addition, prospective triggering depends greatly on the regularity of the patient’s heart rate and can result in serious misregistration artifact in the setting of arrhythmia.
b. Retrospective gating. Unlike prospective triggering, retrospective ECG gating collects data during the entire cardiac cycle. Once the scan is complete, data from specific periods of the cardiac cycle are used for image reconstruction by retrospective referencing to the ECG signal. This approach allows reconstructions to be made from multiple segments of the cardiac cycle and allows some assessment of cardiac function via four-dimensional reconstruction. However, retrospective gating requires higher radiation dose exposure, although this can be somewhat mitigated by dose modulation (see subsequent text).
3. Other imaging considerations
a. Segmented reconstruction refers to image acquisition algorithms that use scan data from more than one cardiac cycle for image reconstruction. This can reduce the effective temporal resolution of the scan at the cost of a slight increase in radiation dose.
b. Dose (or tube current) modulation. MDCT scanners may operate with fluctuating tube currents that increase radiation dose during portions of diastole (when diagnostic images are most likely to be obtained) and reduce it
during systole. Dose modulation typically reduces effective radiation dose by approximately 33%, and it is most effective at lower heart rates.
during systole. Dose modulation typically reduces effective radiation dose by approximately 33%, and it is most effective at lower heart rates.
4. Image reconstruction and interpretation.
Images are most frequently viewed from axial and double oblique planes, in which the three-dimensional data set is manipulated by the interpreting physician so that multiple planes can be viewed to assess cardiac morphology and coronary anatomy. Additional postprocessing techniques can be performed to provide further diagnostic information or, more frequently, to present to the referring physician.
a. Multiplanar reformation involves creating straight or curved image planes by cutting orthogonally or obliquely through the three-dimensional acquisition. This aids in evaluating complex three-dimensional structures, such as the coronary arteries.
b. Maximal-intensity projections are created by compressing a predetermined volume of image data into a two-dimensional projection of the brightest voxels. This is similar in principle to the two-dimensional images created by typical invasive angiography.
c. Three-dimensional or volume rendering is an advanced image processing approach that uses semitransparent visualization of the outer contours of volumetric data, giving the appearance of a three-dimensional structure. Although often not as useful for assessing smaller structures, these reconstructions can be very helpful for understanding complex spatial relationships between major intrathoracic structures.
d. Four-dimensional or cine imaging from spiral retrospectively gated images generates cine images of the CT data for evaluating cardiac and valvular function.
E. Contrast-enhanced imaging.
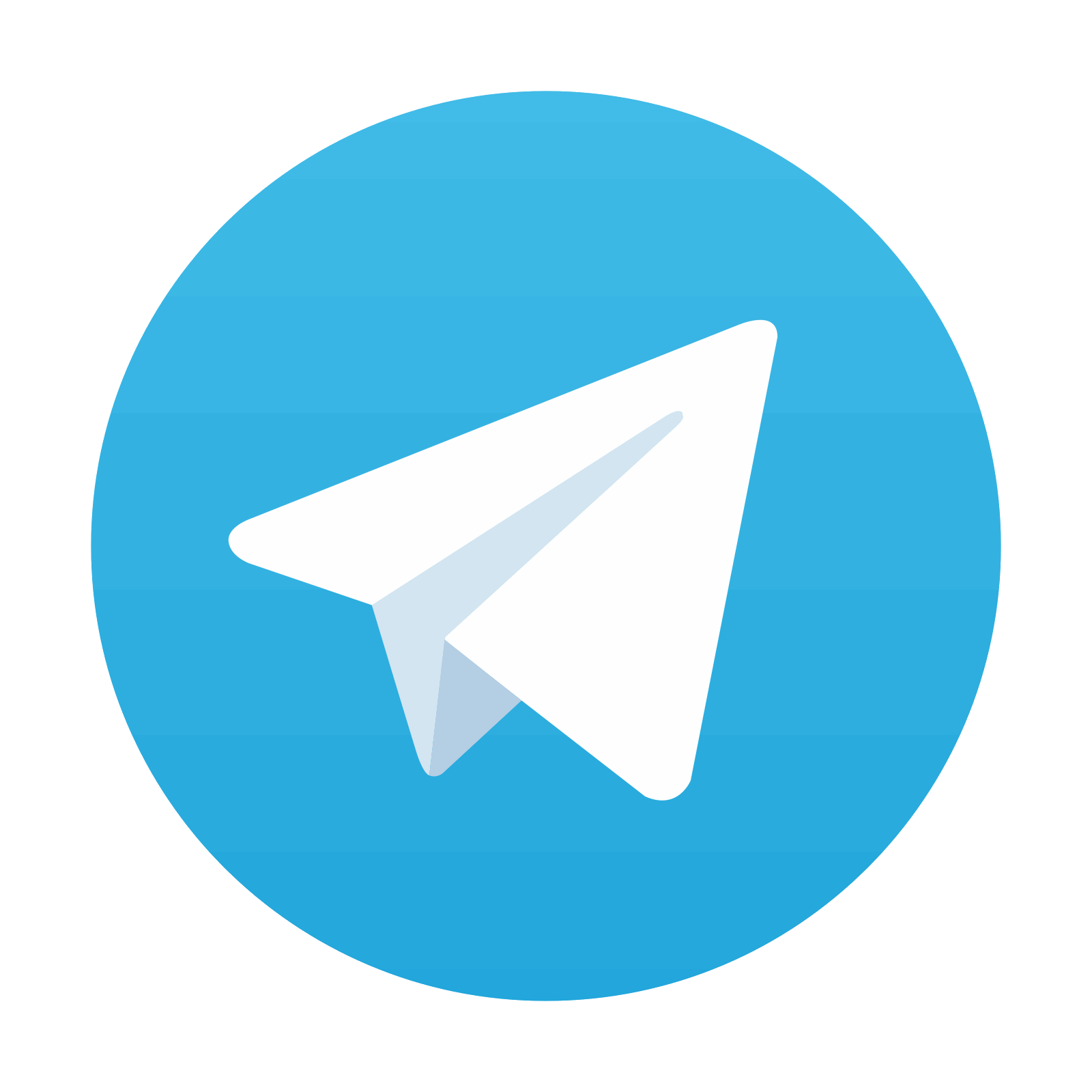
Stay updated, free articles. Join our Telegram channel

Full access? Get Clinical Tree
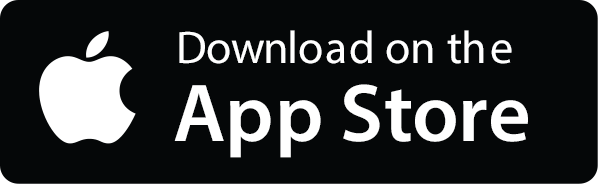
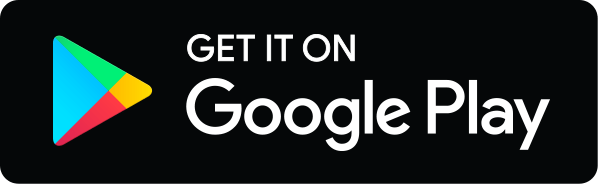