Level
Changes
Consequences
Structural
Systemic arterial vessels
Intimal and medial thickening of large artery walls
Changes in wall matrix (increase in elastolytic and collagenolytic activity, increased collagen deposition, fracture of the elastin lamellae, with gradual loss of arterial elasticity)
Reduced medial smooth muscle cell number and changes in their tone
Increased secretion of proinflammatory cytokines and increased uptake of plasma lipoproteins by endothelial and smooth muscle cells
Elevated systolic arterial pressure and pulse-wave velocity, augmentation of aortic impedance and cardiac mechanical afterload. Atherosclerosis
Myocardium
Alterations of intracellular molecular and biochemical pathways
Stiffening of myocardial cells, mural connective tissue and valves, decreased number of myocytes, increased myocyte size, increased rate of myocyte apoptosis, and blunted adrenoceptor-mediated contractile and inotropic response Fibrotic remodeling (reactive fibrosis and replacement/reparative fibrosis, perivascular fibrosis)
Decreased mechanical and contractile efficiency. Increased left ventricular stiffness
Decreased coronary blood flow
Impaired relaxation and diastolic dysfunction
Coronary arteries
Endothelium-dependent dilation of large epicardial and resistance coronary vessels becomes blunted
Basal and stimulated release of nitric oxide by the coronary endothelium decreases. Coronary vasoconstrictor effect of endothelin-1 increases
Coronary flow reserve gradually impairs as result of both abnormal vasodilatory capacity and elevated cardiac work at baseline
Cellular
Stiffening of vascular smooth muscle cells
Differential expression of proteins involved in the mechanical regulation of vascular function
Changes in cell proliferative potential, increased propensity to undergo cell death, elevated DNA damage, and extensive telomere shortening and dysfunction, oxidative stress-related damage
Arterial rigidity
Cell senescence
Ultrastructural
Accumulation of dysfunctional organelles, DNA mutations, oxidized proteins and lipids into endothelial and smooth muscle cells, cardiomyocytes and fibroblasts owing to impaired autophagy
Cell senescence
- 1.
Structural level. Aging may involve systemic arterial vessels, coronary arteries, and myocardium with different pathophysiological mechanisms and related consequences:
- a)
Systemic arterial vessels. Morphology and function of arterial vessels change with aging. Some changes have a genetic basis, others occur as a result of intrinsic cellular or systemic stimuli. Genetic predisposition determines racial differences, with accelerated arterial aging among African–Caribbean individuals. Moreover, age-related artery change is more pronounced in women, reflecting intrinsic differences in arterial properties between sexes: although men have more prominent, accelerated aging over time compared with women, there are complex effects of hormone deficiency and replacement therapy on CVD. Postmenopausal women exhibit higher aortic rigidity and lower vascular compliance in relation to men, which has a significant impact on their mortality and is probably caused by estrogen deprivation. Even though the smaller diameter of the aortic root has been considered responsible for women’s increased pulse pressure, there is strong evidence that the vascular wall composition is the most important factor responsible for it. Overall, with increasing age, large arteries are known to dilate and thicken their walls, particularly because of wall matrix changes and an increase in elastolytic and collagenolytic activity and in smooth muscle tone. In particular, vessel aging leads to intimal and medial thickening (vascular remodeling), and to the gradual loss of arterial elasticity: reduced medial smooth muscle cell number, increased collagen deposition, and fracture of the elastin lamellae are common characteristics of aging vessels. Overall, these changes may lead to vessel dilation and increased lumen size. Increased collagen and decreased elastin content are promoted, at least in part by the age-associated increase in glycated proteins, matrix metalloproteinase enzyme activity, and trophic stimuli such as angiotensin II signaling. As a final result, vascular stiffness increases with advancing age [4–6], and is in turn the main cause of subsequent elevated systolic arterial pressure and pulse-wave velocity: pulse pressure waves reflect early and peak systolic pressure appears late. Hypertension can further stimulate collagen production with increased vessel stiffness and endothelial cell dysfunction. The final step is the augmentation of aortic impedance and cardiac mechanical afterload. Thus, arterial stiffening triggers a variety of cardiac adjustments, which are additional and similar to the age-related intrinsic changes in cardiac morphology and may, therefore, be expected to worsen cardiac performance. Moreover, aging endothelial and smooth muscle cells also show increased secretion of proinflammatory cytokines and increased uptake of plasma lipoproteins, which ultimately constitute the substratum of atherosclerosis (see below).
- b)
Myocardium. At the heart level, aging is known to determine numerous ionic, molecular, and biochemical changes [7] that affect protein function, mitochondrial oxidative phosphorylation, excitation–contraction coupling, calcium kinetics, myofilament activation, matrix composition and regeneration, cell growth and size, and the apoptosis process. Alterations of the intracellular molecular and biochemical pathways produce modifications in cardiac morphology, which ultimately have an impact on cardiac function. Decreased mechanical and contractile efficiency, stiffening of myocardial cells, mural connective tissue and valves, a decreased number of myocytes, increased myocyte size, an increased rate of myocyte apoptosis, and blunted-adrenoceptor-mediated contractile and inotropic response are all distinctive signs of cardiac aging [8]. Pathological fibrosis in the aging mouse heart is associated with dysregulated resident mesenchymal stem cells arising from reduced stemness and aberrant differentiation into dysfunctional inflammatory fibroblasts. Fibroblasts derived from aging mesenchymal stem cells secrete higher levels of collagen type 1, which directly contributes to fibrosis, monocyte chemoattractant protein-1, which attracts leukocytes from the blood, and interleukin-6, which facilitates the transition of monocytes into myeloid fibroblasts. The intrinsic change in the mesenchymal stem cell phenotype acquired by advanced age is specific to the heart, as mesenchymal stem cells originating from the bone wall or fibroblasts derived from them are free of these alterations [9]. Two types of fibrotic remodeling can potentially occur in the myocardium: reactive fibrosis (also known as diffuse fibrosis), which describes the expansion of existing collagen fibers without a significant loss of myocytes, and replacement/reparative fibrosis, or scar formation (focal fibrosis), which occurs when collagen is newly deposited in the place of necrotic/apoptotic myocytes. Diffuse, reactive fibrosis is a typical feature of the aging heart. However, some amount of replacement fibrosis may also occur; myocyte apoptosis and necrosis increase with age. Conversely, neither reactive nor reparative fibrosis correlates with age in patients with idiopathic dilated cardiomyopathy. The significance of these fibrotic patterns is likely diverse, as the nature or quality of the collagen network may have a differential impact on myocardial stiffness or signal propagation. It is known that reactive fibrosis increases left ventricular stiffness. Accumulation of collagen surrounding blood vessels in the heart is named perivascular fibrosis and may precede or act as an extension of reactive fibrosis. Perivascular fibrosis increases in the aging heart. In patients with non-ischemic heart failure, perivascular fibrosis is associated with decreased coronary flow of the left anterior descending artery, and such impaired coronary blood flow has been demonstrated to correlate with elevated left ventricular wall stress in patients with heart failure. Perivascular fibrosis occurring during aging may have local or paracrine effects on the surrounding myocardium, which in turn could contribute to cardiac dysfunction in elderly subjects. In particular, myocardial fibrosis may be a contributing factor for impaired relaxation and diastolic dysfunction. From this point of view, excess collagen, rather than myocyte hypertrophy, causes myocardial stiffness. Indeed, in experimental models of hypertension-induced diastolic heart failure, collagen deposition, hypertrophic remodeling, and myocardial stiffness are abrogated by an angiotensin II receptor antagonist. In a clinical human setting, contradictory findings have been shown: in some studies of hypertensive patients, those presenting with diastolic heart failure were older and had higher levels of serum markers of collagen turnover; in others, no correlation has been found between diastolic dysfunction and collagen content in the aging heart [10].
- c)
Coronary arteries. Pathophysiological modifications related to aging have also been shown to involve also structure and function of coronary vasculature, which could affect myocardial perfusion. Coronary flow reserve is gradually impaired and such a reduction may be the result of abnormal vasodilator capacity, elevated cardiac work, and myocardial blood flow at baseline [11]. In particular, the endothelium-dependent dilation of large epicardial and resistance coronary vessels becomes blunted [12], basal and stimulated release of nitric oxide by the coronary endothelium decreases [13], and the coronary vasoconstrictor effect of endothelin-1 [14], whose plasma concentrations are raised in the elderly [15], increases, thus finally determining a reduction of the coronary vasodilatory reserve.
- a)
- 2.
Cellular level. Contrary to the traditional paradigm, which associated vascular compliance only with extracellular matrix composition, vascular smooth muscle cells have been recently demonstrated to contribute, by visco-elasticity, to the vessels’ overall mechanics. Under physiological conditions, the mechanical behavior of vascular smooth muscle cells varies according to the position in the arterial tree and intensity of regional mechanical forces, whereas, in the pathological scenario, their cytoplasm stiffening is associated with aging and arterial hypertension, causing arterial rigidity in the absence of extracellular matrix modifications. Thus, almost one-third of the differences found between stiff and distensible vessels are due to the differential expression of proteins involved in the mechanical regulation of vascular function, especially the components of the vascular smooth muscle cell cytoskeleton and extracellular matrix. Aging determines marked changes in vascular smooth muscle cells and inflammatory cells. First, the ability of cells to divide is lost with aging. Such an irreversible change depicts so-called cell senescence. Two types of cell senescence have been described: “replicative” senescence refers to exhaustion of the proliferative lifespan over time, a characteristic of aging, associated with critically shortened telomeres at the chromosomal ends, which then induce a DNA damage response. “Stress-induced premature” senescence is triggered by external stimuli, including oxidizing agents and radiation, which activate the intracellular senescence cascade prematurely. Prematurely and naturally aging cells share several characteristics: changes in cell proliferative potential, increased propensity to undergo cell death, elevated DNA damage, and extensive telomere shortening and dysfunction. In aging vessels, vascular smooth muscle cells undergo reduced proliferation and prolonged population doubling times. Although most of these cells are presumed to be derived from the vessel wall itself, a proportion of cells expressing smooth muscle or endothelial cell markers may be procured from bone marrow-derived progenitor cells and endothelial progenitor cells that migrate and integrate into the vessel wall. They may also be affected by aging, which determines impaired migration and adhesion, owing to the reduced expression of cell surface markers and cytokines for chemotaxis and increased oxidative stress and inflammation. Oxidative stress plays a major role in age-related damage of the cardiovascular system at the cellular level.
- 3.
Ultrastructural level. Aging is depicted by the accumulation of dysfunctional organelles, DNA mutations, and oxidized proteins and lipids into endothelial and smooth muscle cells, cardiomyocytes, and fibroblasts: this phenomenon contributes to alteration of the normal physiological function of the cells. Under homeostatic conditions, abnormal constituents and organelles are eliminated through sequestration into double membrane vesicles, which subsequently deliver the content to a lysosome for degradation. This tightly regulated control of cell quality is ensured by the so-called autophagy, an intracellular pathway responsible for removing senescent and dysfunctional cellular components. Its activation involves the coordination of multiple protein complexes at several different stages: activation, formation, and elongation of the phagophore, engulfment of the cargo, and delivery to the lysosome for degradation. Such a complex activity is regulated by both extra- and intracellular signals, which are centrally inhibited by the mammalian target of rapamycin (mTOR) and activated by 5′ AMP-activated protein kinase (AMPK). When activated, mTOR inhibits autophagy, throughout the PI3K/Akt signaling pathway [16]. Studies have shown that under nutrient-rich conditions, autophagy is depressed. In contrast, during nutrient-limiting conditions, autophagy is activated by the energy sensor AMPK. Once started, autophagy proceeds with the formation and elongation of a small vesicular sac (named phagophore), through lipid acquisition. Lipid sources for phagosomes may derive from the membrane of mitochondria, endoplasmic reticulum, and Golgi, or may be composed of membranes from multiple sources to avoid affecting the homeostasis of a specific subcellular compartment [17]. Probably, different types of autophagy induction lead to the formation of different types of phagosomes. The biogenesis and elongation of the phagosome is regulated by a number of conserved autophagy-related proteins and involves two ubiquitin-like conjugation systems. The growing phagophore elongates until it closes on itself, engulfing the cargo to be degraded. Then, the newly formed structure fuses with a lysosome to form the autolysosome, in which cargo and inner membrane of the autophagosome are subsequently degraded by the lysosomal hydrolases. Although few studies have directly assessed autophagic activity in aging tissues, it is widely accepted that autophagy declines with age and that this impairment is a major contributor to aging. Mice with impaired autophagic capability have an accelerated aging phenotype, including the development of left ventricular hypertrophy, decreased fractional shortening, and premature death [18]. This finding confirms that clearance of damaged proteins is an important cellular quality control mechanism that prevents premature aging, and autophagy may conceivably have an anti-aging effect on the cardiovascular system. There are many potential causes underlying the alterations in cardiovascular function with age, but the accumulation of cytotoxic proteins and dysfunctional organelles contributes to the development of age-related pathological conditions, such as skeletal muscle atrophy, neurodegeneration, and cardiac dysfunction. The underlying mechanisms for the reduction in autophagy in aging tissues are not well understood and probably involve numerous proteins. The most reliable hypothesis is that changes in metabolism and hormonal responses with age might be associated with transcriptional downregulation of autophagy, which could contribute to the age-dependent development of CVD observed. For instance, in rats, long-term excessive fat intake inhibits autophagic flux in the heart and is associated with myocyte apoptosis and cardiac dysfunction [19]. Under such conditions, reactive oxygen species (ROS), which are usually generated in the cell at low levels as a by-product of mitochondrial respiration and have signaling functions, are increased. Excessive ROS negatively affects cellular processes by modifying proteins and lipids and inducing DNA damage. When mitochondria become dysfunctional in aging cells, excessive ROS production contributes to impaired autophagy. If autophagic activity is reduced with age, dysfunctional mitochondria tend to be less frequently removed and to accumulate in aging myocytes.
More recent evidence has shown that microRNAs (miRNAs), which are small noncoding RNAs, post-transcriptionally controlling gene expression by inhibiting translation or inducing degradation of targeted mRNAs, can contribute in the regulation of longevity and senescence. In humans, comparisons of miRNA expression between peripheral blood mononuclear cells of young versus old individuals reveal the downregulation of various miRNAs. Several studies have addressed the regulation of miRNAs during culture-induced senescence of vascular cells. In human cell culture studies, miRNAs were detected in senescent endothelial cells: some miRNAs are up-regulated, others are down-regulated, whereas in the entire vessel wall miRNAs are generally up-regulated. These differences between the profiles of cultured endothelial cells and entire vessels likely reflect the different cellular compositions as, in whole vessels, smooth muscle cells and invaded inflammatory cells, but not endothelial cells, are the predominant cell types. Some specific miRNAs are well known to repress matrix proteins in the heart, and the increased expression of such miRNAs in the aorta of aged mice is associated with a decline in matrix protein expression, such as collagens and elastin, which is considered to contribute to aneurysm formation, a vascular disease typically associated with age. Potential development of therapeutic miRNA targeting strategies is currently further limited by the need to understand whether the deregulated miRNAs during aging are causes or effectors of the age-associated disease or if they may even act as compensatory feedback loops. The knowledge that miRNAs are involved in more complex regulatory pathways in competition with other competitive endogenous RNAs makes the scenario challenging. Given that such interactions likely depend on the number of molecules of each interacting species, new models that take into account quantitative biology are required to understand network modulation and finally develop therapeutic interventions [20].
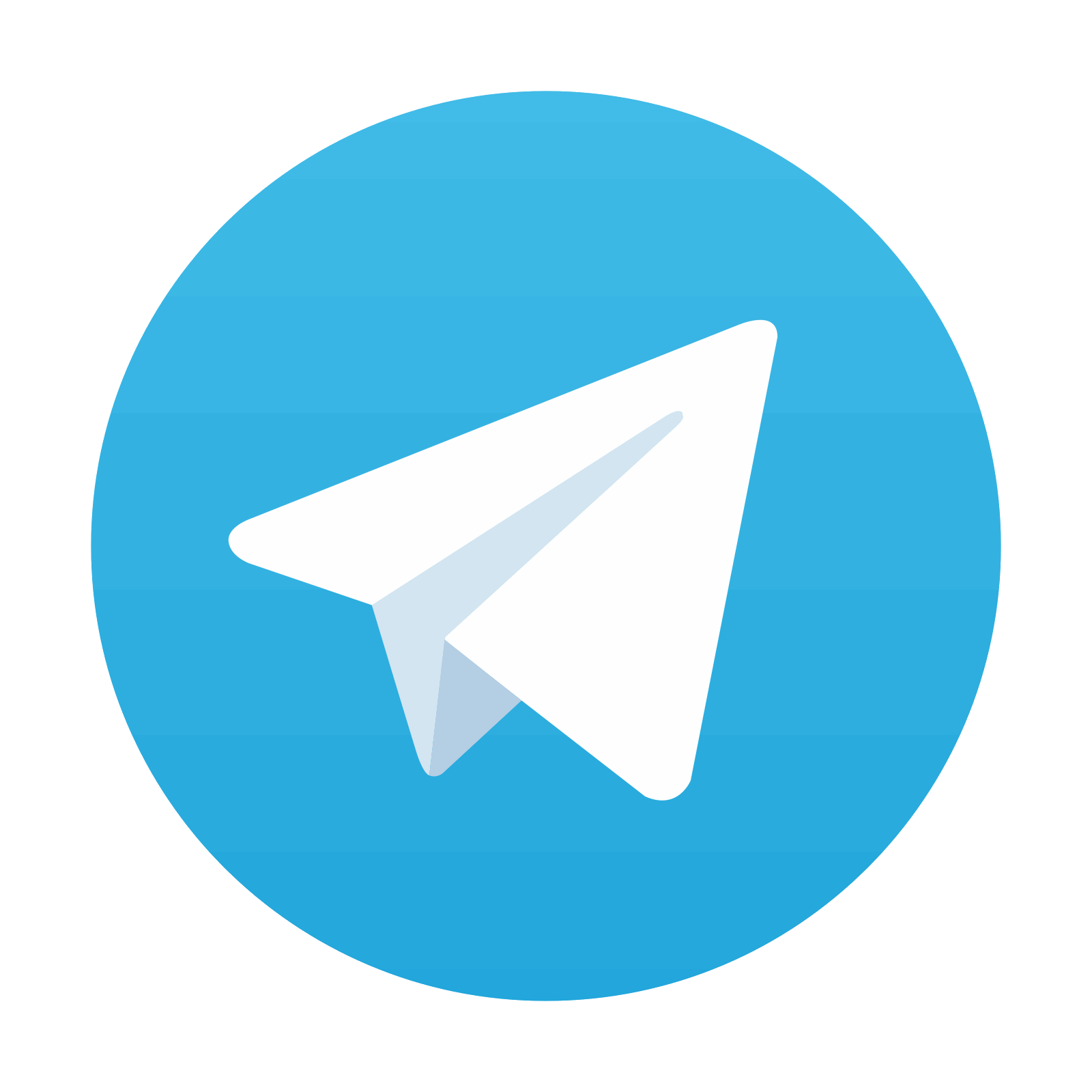
Stay updated, free articles. Join our Telegram channel

Full access? Get Clinical Tree
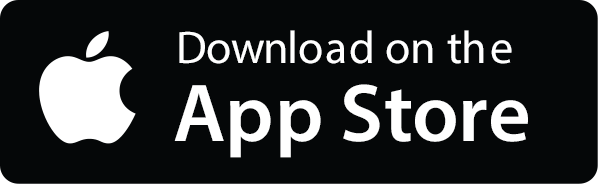
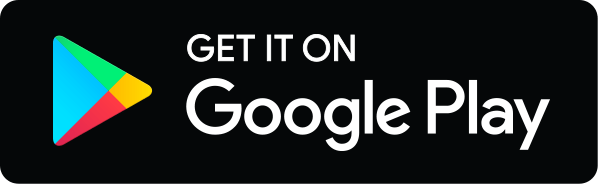