Agents
Drug class
Cancer clinical use
Type of cardiotoxicity
Frequency
Chemotherapeutic agents
Doxorubicin
Anthracyclines
Breast, sarcoma, lung, bladder, gastric, prostate, leukemia, lymphoma, others
LV dysfunction
Common
Epirubicin
Anthracyclines
Breast, esophageal, gastric
Arrhythmia
Uncommon
Cyclophosphamide
Alkylating agents
Sarcoma, SCT, lymphoma, myeloma, breast
Myopericarditis, arrhythmias
Common
Ifosfamide
Alkylating agents
Testicular, sarcoma, lymphoma
Arrhythmias, LV dysfunction
Common
Cisplatin
Alkylating agents
Lung, bladder, testicular, sarcoma, breast, esophageal, head and neck
Arrhythmias, ischemia, vascular toxicity
Uncommon
5-Fluorouracil
Antimetabolites
Colon, pancreatic, breast, head and neck
Coronary vasospasm, ischemia, arrhythmias
Common
Capecitabine
Antimetabolites
Breast, colon, gastric, pancreatic
Chest pain, ischemia, arrhythmias
Uncommon
Fludarabine
Antimetabolites
Lymphoma, leukemia, stem cell transplant
Chest pain
Rare
Vinblastine
Antimicrotubule
Lymphoma, testicular, lung, melanoma
Ischemia, hypertension
Common
Paclitaxel
Antimicrotubule
Breast, ovarian, lung, sarcoma, bladder, cervical, gastric, esophageal, head and neck
Arrhythmias
Rare
Docetaxel
Antimicrotubule
Breast, lung, prostate, gastric, head and neck
Arrhythmias, LV dysfunction
Uncommon
Biologic agents
Bevacizumab
Antibody (VEGF)
Colon, rectal, cervical, glioblastoma, ovarian, renal, endometrial, sarcoma
Hypertension, LV dysfunction
Common
Trastuzumab
Antibody (HER-2)
Breast, gastric, gastro-esophageal
LV dysfunction
Common
Pertuzumab
Antibody (HER-2)
Breast
LV dysfunction
Uncommon
Alemtuzumab
Antibody (CD-52)
Leukemia, stem cell transplant
Arrhythmias
Rare
Cetuximab
Antibody (EGFR)
Colon, rectal, head and neck, lung, squamous skin
Ischemia, cardiorespiratory
Uncommon
Ramucirumab
Antibody (VEGFR-2)
Colon, rectal, gastric, lung
Hypertension, thromboembolism
Common
IL-2
Immune agent
Melanoma, renal
Capillary leak syndrome, hypotension, myocardial toxicity
Common
INF
Immune agent
Melanoma, renal, lymphoma
Arrhythmias, ischemia
Common
Tyrosine kinase inhibitors
Sunitinib
VEGFR, PDGFR, c-Kit
Renal, thyroid, sarcoma, GIST, PNET
Hypertension, LV dysfunction, thrombosis
Common
Sorafenib
VEGFR-2, PDGFR, RAF-1, c-Kit
Hepatocellular, renal, thyroid, angiosarcoma, GIST
Hypertension, ischemia, LV dysfunction
Common
Pazopanib
VEGFR -1–3, PDGFR, FGFR-1, FGFR-3, c-Kit
Renal, sarcoma, thyroid
Hypertension, LV dysfunction, arrhythmias, ischemia, thromboembolism
Common
Axitinib
VEGFR-1–3
Renal
Hypertension, thromboembolism
Common
Lapatinib
EGFR1, HER2
Breast
LV dysfunction
Uncommon
Imatinib
BCR/ABL, PDGF, c-Kit
Leukemia, GIST, MDS, melanoma, mastocytosis, sarcoma
LV dysfunction, edema
Rare
Dasatinib
BCR/ABL, Src, c-Kit
Leukemia, GIST
Pleural effusion, LV dysfunction, arrhythmias
Uncommon
Trametinib
MEK1/MEK2
Melanoma
Hypertension, LV dysfunction
Common
Vandetanib
VEGFR-2, EGFR, RET
Thyroid
Hypertension, prolonged QT
Common
Ponatinib
BCR/ABL
Leukemia
LV dysfunction Vascular events
Rare
Common
Regorafenib
VEGFR -1–3, KIT, PDGFR-α, PDGFR-β, RET, FGFR-1 and FGFR-2
Colon, rectal, GIST
Hypertension
Common
Cediranib
VEGFR-1–3, PDGFR-α/β, FGFR-1, c-Kit
Various solid tumors
Hypertension
Common
Proteasome inhibitors
Bortezomib
26S
Multiple myeloma, mantle cell lymphoma
LV dysfunction, hypotension
Uncommon
Carfilzomib
26S
Multiple myeloma
LV dysfunction
Vascular events
Uncommon
Common
Decoy receptors
Aflibercept
VEGFR -1, VEGFR-2
Colon, rectal
Hypertension
Common

Fig. 2.1
Manifestations of cardiotoxicity with selected anticancer agents

Fig. 2.2
Proposed therapeutic management of patients with anticancer therapy—induced left ventricular dysfunction
Chemotherapy
Anthracyclines
Doxorubicin
Anthracycline-induced cardiotoxicity was initially reported more than four decades ago during the early clinical development of doxorubicin [7, 8]. The incidence of anthracycline-induced heart failure (HF), based on clinical signs and symptoms, was reported to be less than 3 % [8]. However, the development of noninvasive monitoring techniques enabled the detection of a higher incidence of cardiac dysfunction [9]. The key mechanisms involved in the pathogenesis of cardiac dysfunction are increased reactive oxygen species and alteration of topoisomerase IIb, which are both associated with damage to cardiomyocytes [10, 11]. Acute cardiac dysfunction is reported in 3.2 % of patients receiving anthracyclines [12], and it occurs within several weeks of the initiation of therapy. Acute cardiac dysfunction presents with electrocardiographic abnormalities, including arrhythmias (supraventricular and ventricular), heart block, ventricular dysfunction, increased cardiac filling pressures, HF, and pericarditis-myocarditis syndrome [13–17]. Cardiac dysfunction may present as a reduction in left ventricular ejection fraction (LVEF) in up to 20 % of patients and may not become evident until after the completion of chemotherapy [18–20].
These late-occurring complications are serious and consist of HF that develops within a few months to several years (most frequently within 3 months) after anthracycline therapy [8, 21–23]. However, symptomatic HF can occur more than a decade after treatment, which represents a major clinical concern, especially among survivors of childhood malignancies [22]. Chronic cardiomyopathy can present as asymptomatic diastolic or systolic dysfunction, which frequently progresses to HF. The incidence of cardiac dysfunction is directly related to the cumulative dose of anthracyclines, but it may also occur at low doses [24]. Other risk factors for the development of cardiac dysfunction include being elderly or a child at the time of drug exposure, concomitant administration of other cardiotoxic agents (such as trastuzumab), radiation therapy to the chest, and preexisting cardiovascular disease. Awareness of these risks for cardiac dysfunction may lead to the early identification and treatment of HF [25].
The encapsulation of doxorubicin in a liposomal moiety (pegylated liposomal doxorubicin) allows for the administration of higher cumulative doses with similar efficacy and a lower rate of HF and myocardial damage compared to doxorubicin [26]. Despite the better cardiac safety profile of liposomal doxorubicin compared to doxorubicin [clinical cardiotoxicity (odds ratio [OR] 0.18) and subclinical cardiotoxicity (relative risk [RR] 0.31)] [27], the US Food and Drug Administration (FDA) recommends routine surveillance of LVEF with the use of liposomal doxorubicin.
Dexrazoxane is an ethylenediaminetetraacetic acid (EDTA)-like chelator that binds iron and protects cardiomyocytes from the effects of doxorubicin [28]. The cardioprotective efficacy of dexrazoxane with the use with either doxorubicin or epirubicin has been clinically confirmed [27]; however, some concerns about lower response rate to chemotherapy and secondary leukemia in childhood cancer survivors have arisen [29].
Epirubicin
Epirubicin is less cardiotoxic than doxorubicin, and it is sometimes considered the preferred anthracycline [30, 31]. In comparison to doxorubicin, epirubicin significantly decreased the risks of both clinical (OR 0.39, 95 % CI [0.2–0.78]) and subclinical (OR 0.30, 95 % CI [0.16–0.57]) cardiotoxicity [27]. According to the FDA, the cumulative dose of epirubicin should be limited to 900 mg/m [2].
Alkylating Agents
Cyclophosphamide
Cyclophosphamide , which has been associated with reduced cardiac function, pericardial effusion, and decreased electrocardiographic (ECG) voltage (even without pericardial effusion) [32–34], is used in high-dose regimens that accompany stem cell transplantation [35]. The incidence of cyclophosphamide-associated cardiotoxicity is not clearly dose dependent [32, 33]. Cyclophosphamide also causes hemorrhagic myopericarditis with pericardial effusion that is attributed to endothelial capillary damage [32, 36]. These cardiac complications are mostly conservatively managed, but they can in rare instances lead to tamponade and death [32]. Factors that increase the risk of cyclophosphamide -associated cardiotoxicity include prior radiation therapy to the mediastinum or left chest wall, older age, and prior reduced LVEF.
Ifosfamide
Cisplatin
Cisplatin-induced cardiotoxicity includes supraventricular tachycardia, bradycardia, ST-T wave changes, bundle branch block, acute ischemia with or without myocardial infarction, and cardiomyopathy [39, 40]. Cisplatin is also associated with vascular toxicities, including Raynaud’s phenomenon, hypertension, and cerebral ischemic events. Electrolyte abnormalities, commonly seen with cisplatin -induced nephrotoxicity, can also contribute to cardiotoxicity.
Antimetabolite Agents
5-Fluorouracil
5-Fluorouracil (5-FU) -induced cardiotoxicity can occur in 8–20 % of treated patients [41–43]. Chest pain associated with ECG changes is the most common symptom. Other more significant manifestations include myocardial infarction and arrhythmia. Pericarditis and cardiac arrest are less common. The main pathophysiologic mechanism causing these symptoms is likely coronary artery vasospasm. Other mechanisms include endothelial cytotoxicity, myocarditis, and takotsubo cardiomyopathy [44]. Risk factors include infusion of 5-FU (vs. bolus), preexisting coronary artery disease, and concurrent use of radiation therapy or anthracyclines.
The majority of patients respond to the termination of 5-FU treatment or concurrent antianginal therapy with nitrates; however, death has been reported in a small percentage of patients [45]. Restarting therapy is usually not recommended, unless no other effective therapeutic regimens are available. The available data on calcium channel blockers or nitrates as preventative agents are conflicting [41, 46–49].
Capecitabine
Capecitabine is a prodrug that is metabolized to its active moiety, 5-FU, and has cardiotoxicity similar to that of infusional 5-FU [50]. Patients with a history of 5-FU-induced vasospasm will likely experience recurrent symptoms with capecitabine [51]. The incidence of vasospasm, including chest pain/angina, myocardial infarction, and arrhythmia, in patients receiving capecitabine ranges from 3 to 9 % [50, 52, 53]. The management of capecitabine-induced cardiotoxicity is similar to that of 5-FU-induced cardiotoxicity.
Fludarabine
Chest pain and hypotension have been reported with the use of fludarabine [54]. The combination of fludarabine and melphalan as a conditioning regimen for stem cell transplantation has been associated with cardiac dysfunction [55]. Other antimetabolite agents (cladribine, methotrexate, and cytarabine) have been rarely associated with cardiotoxicity in case reports [56–58].
Antimicrotubule Agents
Vinca Alkaloids
Paclitaxel
Paclitaxel is associated with a low incidence of cardiotoxicity that includes asymptomatic bradycardia and heart block [62, 63]. Routine cardiac monitoring is not required during administration of this agent [62]. Nanoparticle albumin-bound paclitaxel (nab-paclitaxel) has the same cardiotoxicity profile as paclitaxel .
Biologic Therapy
Bevacizumab
Bevacizumab, a monoclonal antibody that binds and inactivates vascular endothelial growth factor (VEGF), exerts its anti-tumor effect by preventing microvascular angiogenesis. The most common cardiac adverse event associated with anti-VEGF therapy is hypertension [67]. Preexisting hypertension may predispose patients to worsened hypertension with bevacizumab [68]. While there has been controversy regarding the cardiotoxicity of bevacizumab [69–71], several studies and meta-analyses have identified an increased incidence of HF and decreased LVEF [71]. Early cardiotoxicity manifests as takotsubo cardiomyopathy-like events [72]. However, the pathophysiology of these events is poorly understood [73]. Bevacizumab-induced cardiotoxicity has been noted in patients with renal cell carcinoma (RCC) [70], breast cancer [71, 74], and glioma [75]. In a meta-analysis, the risk of high-grade congestive HF in patients with breast cancer was higher in those receiving bevacizumab than in those receiving placebo (RR 4.74, 95 % CI [1.66–11.18]; P = 0.001), without a dose-related effect [74]. The risk of cardiotoxicity is increased when bevacizumab is combined with docetaxel or anthracycline [74, 76].
Trastuzumab
Trastuzumab is a monoclonal antibody targeting the human epidermal growth factor receptor-2 (HER2 or ErbB2) and is used for tumors that overexpress HER2 protein, including breast and gastric cancers [77–79]. One meta-analysis demonstrated that trastuzumab significantly increased the incidence of congestive HF (RR 5.11, 90 % CI [3.00–8.72]; P < 0.00001) and that the LVEF significantly declined after trastuzumab administration (RR 1.83, 90 % CI [1.36–2.47]; P = 0.0008) [80]. Trastuzumab cardiotoxicity is thought to arise from a loss of contractility (rather than from cardiomyocyte death) and thus may be reversible [81, 82]. Risk factors for trastuzumab-induced cardiotoxicity include prior anthracycline use (worse with doxorubicin >300 mg/m2) [24, 83], preexisting decreased LVEF, hypertension, elevated body mass index, and older age [84].
Pertuzumab
Similar to trastuzumab, pertuzumab targets HER2 (ErbB2) receptors through prevention of HER2 homodimerization [85–87]. Recent studies show a clinical benefit with the use of pertuzumab and trastuzumab combination regimens [87, 88]. The addition of pertuzumab to therapy with trastuzumab and docetaxel was not associated with increased cardiotoxicity in the CLEOPATRA trial [89], and a cardiac safety analysis of early-phase trials showed no increase in cardiotoxicity above that of trastuzumab [90]. In one study with trastuzumab and pertuzumab, one of 64 patients developed an LVEF below 40 % following the completion of therapy [91], and in another study with the same combination, 3.9 % of patients had a decrease of >10 % in their pretreatment LVEF assessment following therapy [92]. The FDA recommends assessment of LVEF prior to initiating anti-HER2 therapies such as trastuzumab and pertuzumab followed by reassessment at regular intervals (every 3 months and 6 months after discontinuation of therapy).
The general consensus is to discontinue trastuzumab plus pertuzumab therapy and reassess LVEF while considering cardioprotective treatment with accepted HF-based therapies in the event of a decrease in LVEF to <45 % or to 45–49 % with a 10 % or greater decrease from pretreatment LVEF values.
Alemtuzumab
Alemtuzumab is a monoclonal antibody targeting CD52, a cell-surface antigen present on B and T cells that, after binding, leads to antibody-dependent cell lysis. This agent is currently approved for the treatment of B-cell chronic lymphocytic leukemia and relapsing multiple sclerosis [93–95]. One study reported the development of decreased LVEF and/or arrhythmia, including atrial fibrillation and ventricular tachycardia, with the use of alemtuzumab in four of eight patients with mycosis fungoides/Sezary syndrome [96]. Recovery of decreased LVEF was seen after discontinuation of therapy in most patients [96].
Cetuximab
Cetuximab is a human/mouse chimeric monoclonal antibody that targets epidermal growth factor receptor (EGFR), resulting in the inhibition of cell growth, apoptosis, cellular VEGF production, and wild-type KRAS activation [97]. Cetuximab is approved for use in KRAS mutation-negative colorectal cancer in combination with FOLFIRI (irinotecan, 5-FU, and leucovorin) or as a single agent in refractory disease. Cetuximab-associated cardiotoxicity events observed in patients with colorectal cancer are limited. One sudden cardiac death was reported in a study of 128 patients undergoing therapy with cetuximab, oxaliplatin, and 5-FU followed by capecitabine [98]. Cetuximab is also approved for the treatment of squamous cell carcinoma of the head and neck, but an FDA black box warning exists for cardiopulmonary arrest, which has been observed in 2 % of patients treated with cetuximab and radiation and is thought to be associated with electrolyte abnormalities [99].
Ramucirumab
Ramucirumab is an antiangiogenic monoclonal antibody that targets the VEGF pathway by binding and blocking ligand-mediated activation of VEGF receptor-2 (VEGFR-2) [100–102]. In the REGARD trial, the use of ramucirumab was associated with a higher incidence of hypertension in patients treated with best supportive care plus ramucirumab compared to patients who received best supportive care plus placebo (16 % vs. 8 %, respectively). The respective rates of arterial thromboembolism were 2 % and 1 %. One patient treated with ramucirumab developed a myocardial infarction leading to death [100].
Interleukin-2
Interleukin-2 is associated with direct myocardial toxicity [103] and with capillary leak syndrome. These events result in increased cardiac output and decreased systemic vascular resistance with a systemic inflammatory response-like syndrome [104, 105], which can be managed with supportive care [106].
Interferon-Alpha
The use of interferon-alpha is associated with cardiotoxicity, mainly arrhythmias (atrial and ventricular tachycardias and heart block), which are reported in 8–20 % of cases [107–109]. One study in melanoma and RCC reported cardiomyopathy in patients treated with interferon-alpha [67, 110]. By contrast, another study demonstrated that only 1 % of patients had a decrease in LVEF [111].
Targeted Therapy
Sunitinib
Sunitinib is an oral tyrosine kinase inhibitor (TKI) targeting VEGFR, platelet-derived growth factor receptor (PDGFR), c-Kit, and fms-like tyrosine kinase-3. Sunitinib is approved by the FDA for use in RCC, advanced pancreatic neuroendocrine tumors, and imatinib-refractory gastrointestinal stromal tumor (GIST) [112]. A retrospective analysis of patients with GIST treated with sunitinib demonstrated that up to 8 % of patients developed clinically significant HF exacerbations, 28 % of patients had at least a 10 % decrease in LVEF, 9 % of patients had a 15 % or greater decrease in LVEF, and 47 % of patients developed hypertension (>150/100 mmHg) [113–115]. Additionally, hypertension preceded the development of life-threatening HF in selected patients treated with sunitinib, which emphasizes the need for the management of hypertension to prevent HF [116]. As hypertension is a common adverse event of anti-VEGF agents, blood pressure should be closely monitored and aggressively managed during all cycles of anti-VEGF-containing therapy. The decrease in LVEF is likely mediated by direct mitochondrial injury and cardiac myocyte apoptosis through inhibition of rapidly accelerated fibrosarcoma (RAF-1) kinase [113, 117] and inhibition of PDGFR coupled with systemic vasoconstriction, leading to cardiac dysfunction [118]. Sunitinib is also associated with QT prolongation and arrhythmias [119]. Preexisting HF, coronary artery disease, and lower BMI may predispose patients to these adverse cardiovascular effects related to sunitinib [120].
Sorafenib
Sorafenib is a small-molecule TKI that inhibits VEGF through inhibition of VEGFR-2, PDGFR, RAF-1, proto-oncogene B-Raf, fms-like tyrosine kinase-3, and c-Kit. Sorafenib is FDA approved for use in RCC, hepatocellular carcinoma, and well-differentiated thyroid cancer [117]. The cardiotoxicity of sorafenib is less well defined than that of sunitinib. In one study, 2.9 % of patients with RCC treated with sorafenib developed myocardial ischemia compared with 0.4 % of those treated with placebo [121]. In patients with hepatocellular carcinoma, myocardial ischemia was noted in 2.7 % of patients treated with sorafenib compared to 1.3 % of those treated with placebo [122]. One meta-analysis suggested an RR of 1.78 (95 % CI [1.09–2.92]) for the development of hypertension with the use of sorafenib [123].
Pazopanib
Pazopanib , a TKI approved for use in soft-tissue sarcoma and RCC, is thought to act through inhibition of surface VEGF receptors 1, 2, and 3, PDGF receptors, fibroblast growth factor receptor (FGFR)-1 and FGFR-3, c-Kit, transmembrane glycoprotein receptor tyrosine kinase, and interleukin-2 receptor-inducible T-cell kinase [124]. One study showed that 49 % of patients developed hypertension with pazopanib use and 6.6 % of patients showed a decrease in LVEF with therapy compared to 2.4 % of control subjects [125]. Patients receiving pazopanib also showed a concentration-independent QT prolongation [126].
Axitinib
Lapatinib
Lapatinib is an oral TKI approved for use in HER2-overexpressing breast cancer. Lapatinib inhibits EGFR (ErbB1) and HER2 (ErbB2) [79, 131] and has thus raised concerns that is has a cardiotoxic effect similar to that of trastuzumab [132]. Recent studies, including one phase III trial investigating the use of lapatinib and trastuzumab in HER2-positive breast cancer, have shown that this theoretical risk may not be a reality [133]. An additional study examining pooled data from 44 trials involving lapatinib alone or in combination with trastuzumab or anthracycline-based regimens found that fewer than 5 % of patients experienced clinically significant cardiac events and that 88 % of patients recovered to pretreatment levels (when an LVEF reduction was noted) following discontinuation of drug therapy [132]. Despite these findings, current FDA labeling recommends pretreatment LVEF evaluation, as well as discontinuation of lapatinib in the event of a decrease in LVEF to <50 %.
Imatinib
Imatinib is a BCR-ABL TKI and an inhibitor of PDGFR stem cell factor and c-Kit [134]. Shortly after its release, an initial concern about LV contractile dysfunction arose due to a suspected loss in mitochondrial membrane potential thought to be secondary to a cellular stress response induced by imatinib [135]. However, subsequent retrospective studies reviewing toxicities in 1276 patients undergoing therapy for chronic myelogenous leukemia (CML) found that imatinib-associated systolic heart failure was observed in only 0.6 % of patients and that adverse cardiovascular events were seen primarily in elderly patients who had preexisting cardiovascular disease or coronary artery disease [136, 137]. Similar findings were observed in patients receiving imatinib for GIST [138–140].
Dasatinib
Dasatinib is a small-molecule TKI that inhibits imatinib-resistant BRC-ABL kinase [141, 142]. Pleural and pericardial effusions occur with the use of dasatinib, but their mechanisms are unknown. One phase III trial comparing once- with twice-daily dosing of dasatinib in accelerated-phase CML observed 0 and 3 % incidences of congestive HF and 12.7 and 24.5 % incidences of pleural effusion, respectively [143]. Dasatinib use has also been associated with QT prolongation [144].
Trametinib
Trametinib is a selective and potent inhibitor of MEK1/MEK2 that is commonly used for the treatment of advanced melanoma with documented BRAF V600E or V600K mutations. In a phase III clinical trial of patients with melanoma, trametinib was associated with decreased LVEF or ventricular dysfunction in 7 % of patients [145]. In 1 % of the patients, these events were grade 3 cardiotoxicities, leading to the permanent discontinuation of trametinib [145]. In addition, hypertension was reported in 15 % of patients (grade 3, 12 %) [145].
Vandetanib
Vandetanib , a multi-kinase inhibitor targeting VEGFR-2, EGFR, and RET, is an effective and FDA-approved treatment for medullary thyroid carcinoma [146]. In a phase III clinical trial, vandetanib was associated with a higher risk of hypertension than placebo (32 % vs. 5 %, respectively). Grade 3/4 hypertension was noted in 9 % of patients. In addition, vandetanib was found to be associated with prolonged QTc (all grades, 14 %; grade 3/4, 8 %) [146].
Bortezomib
Bortezomib is a first-generation reversible 26S proteasome inhibitor that leads to cell cycle arrest and apoptosis and is approved for use in mantle cell lymphoma and multiple myeloma [147, 148]. An increased incidence of HF and associated symptoms has been seen with bortezomib use; however, one study suggested similar rates of HF in patients undergoing therapy for relapsed multiple myeloma with bortezomib or high-dose dexamethasone [149] and found that the effects, if present, did not appear to be dose dependent [148].
Carfilzomib
Carfilzomib is a second-generation 26S proteasome inhibitor that causes cell cycle arrest and apoptosis and is approved for use in multiple myeloma [150, 151]. Carfilzomib has been implicated in LVEF reduction, instigation of new-onset HF or exacerbation of preexisting HF, and, in limited cases, myocardial infarction. One study demonstrated that 2 of 257 patients had a myocardial infarction shortly after initiation of carfilzomib therapy and 9 (3.4 %) developed grade 3/4 dyspnea; however, the vast majority of patients in this trial had previously been treated with bortezomib [152]. Another study suggested an 11 % incidence of HF-associated symptoms following carfilzomib initiation [153]. It is likely that, similar to that with anthracyclines, the cardiac adverse events seen with carfilzomib are not limited to LV systolic dysfunction but include an increase in thrombotic events as well [154].
Ponatinib
A BCR-ABL tyrosine kinase inhibitor, ponatinib is approved for use in Philadelphia chromosome-positive acute lymphoblastic leukemia and CML [155]. One phase I study demonstrated that 1 % of patients exhibited grade 3/4 HF-like symptoms and 2 % of patients exhibited a prolonged QTc during therapy [155]. Another study suggested serious adverse vascular events, with 3 % of patients having ponatinib -associated arterial thrombotic events and 9 % of patients having arterial thrombotic events observed during therapy (although these were not necessarily considered treatment associated) [156]. Understanding the nature of these events is crucial for the future of this therapy [157].
Regorafenib
Regorafenib is a multi-kinase inhibitor approved for use in metastatic colorectal cancer and GIST that targets VEGF receptors 1–3, KIT, PDGFR-alpha, PDGFR-beta, RET, FGFR-1 and FGFR-2, TIE2, DDR2, TrkA, Eph2A, RAF-1, BRAF, BRAFV600E, SAPK2, PTK5, and ABL [158, 159]. Although HF has not been reported with regorafenib, grade 3/4 hypertension is a frequently reported toxicity [158, 160–162].
Cediranib
Cediranib is a multi-kinase inhibitor targeting the VEGF pathway and angiogenesis through VEGFR-1, VEGFR-2, and VEGFR-3, PDGFR-alpha/PDGFR-beta, FGFR-1, and c-Kit [163]. As with other inhibitors of the VEGF pathway, hypertension is a primary toxicity of cediranib and has been identified in several studies [163–166], with one phase II study showing that 46 % of patients enrolled exhibited hypertension with grade 3 or higher toxicity [167].
Aflibercept
Aflibercept is an antiangiogenic agent acting as a decoy receptor for VEGF-A and VEGF-B and placental growth factor composed of components of VEGFR-1 and VEGFR-2 binding domains attached to the Fc portion of a human IgG-1 [168]. One trial showed a significantly increased incidence of hypertension with aflibercept treatment, with grade 3 adverse events occurring in 19 % of patients compared to 1.5 % of control subjects as well as an increased incidence of arterial-thromboembolic events (1.8 % of patients vs. 0.5 % of control subjects) and venous-thromboembolic events (7.9 % of patients vs. 6.3 % of control subjects) [168]. Other studies have also found an increased incidence of hypertension and thromboembolic events in patients receiving aflibercept [169–171].
Conclusions
The exponential development of novel therapeutics for the treatment of cancer has prompted extensive research to recognize cardiotoxicity associated with the use of these agents. Early detection and effective therapeutic management of adverse events associated with the use of anticancer drugs have led to the safe and successful development of several breakthrough FDA-approved drugs, which have improved the clinical outcomes of patients with cancer. As cardiotoxicity is a major challenge—associated with severe complications and comorbidities—in the development of novel therapeutic agents [172], rigorous monitoring for adverse events has been successful in eliminating antineoplastic agents with severe cardiotoxicity [173].
In the current review, as expected, results from clinical trials in select patient populations were not always similar to the results derived from observational studies. This difference may be attributed to patient heterogeneity and the innate differences between these types of studies. Interestingly, in many instances case reports and case series raised awareness, thereby emphasizing the need to encourage clinical investigators to publish case reports.
In conclusion, collaborative efforts between cardiologists and oncologists have decreased the incidence of severe cardiotoxicity in patients treated with potentially cardiotoxic or novel anticancer agents and promise to eliminate cardiotoxicity associated with the use of new drugs (Fig. 2.2).
References
1.
Thakur A, Witteles RM. Cancer therapy-induced left ventricular dysfunction: interventions and prognosis. J Card Fail. 2014;20:155–8.PubMed
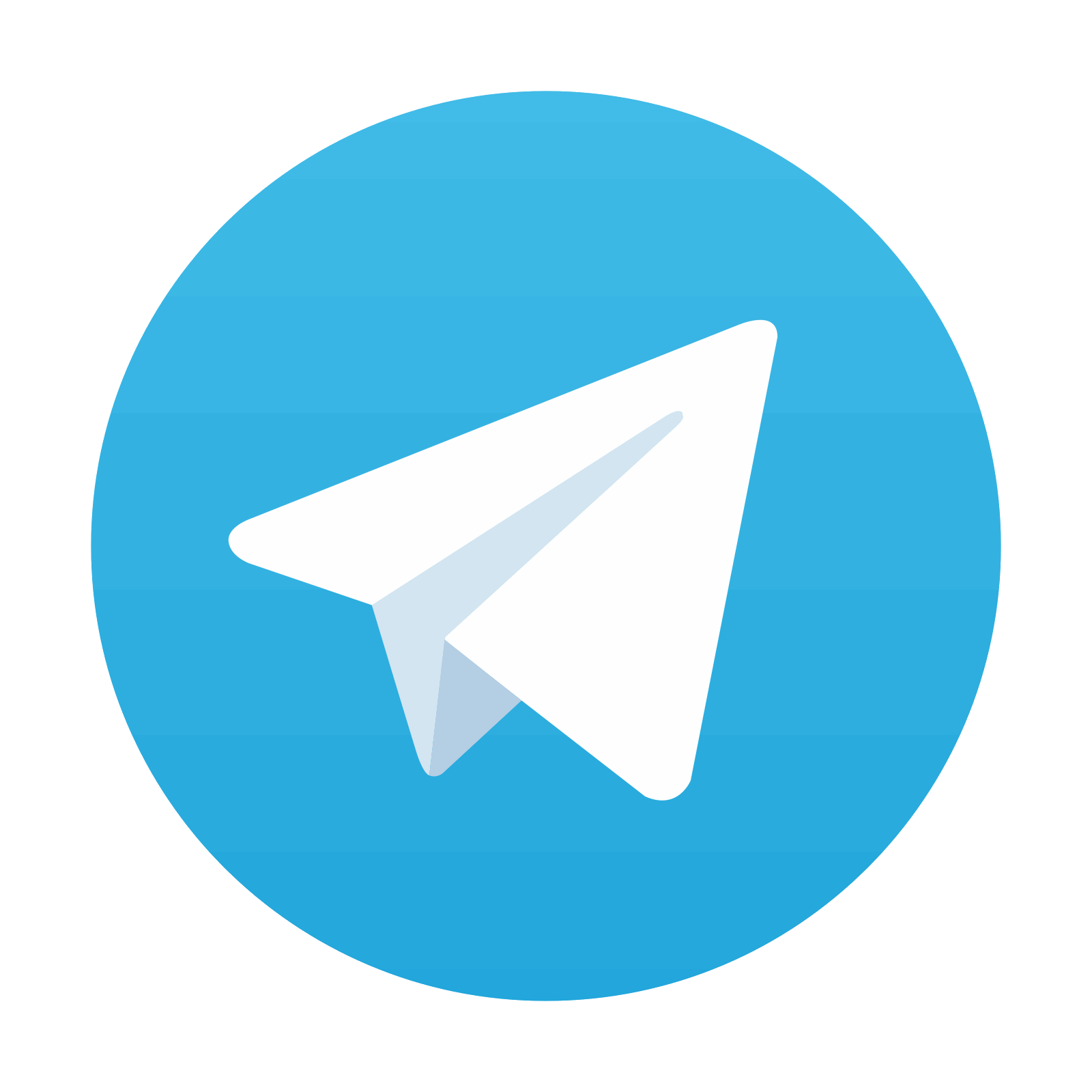
Stay updated, free articles. Join our Telegram channel

Full access? Get Clinical Tree
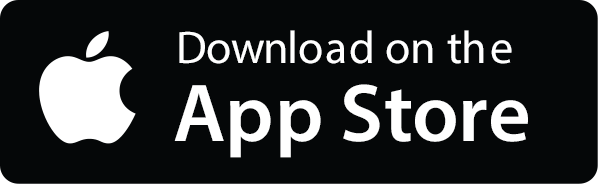
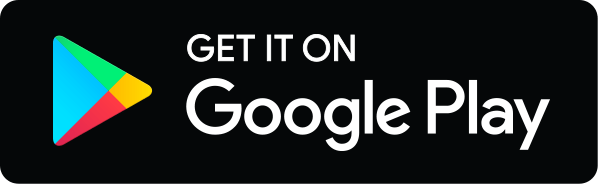