Introduction
Historically, cardiothoracic critical care developed from a need to provide advanced monitoring and organ support for patients who had recently undergone cardiothoracic surgery. While this remains at the core of cardiothoracic critical care, there has been significant diversification. A cardiothoracic critical care unit often cares for patients requiring extracorporeal life support (ECLS) for advanced respiratory and heart failure, transplant recipients and patients who have undergone interventional cardiological procedures.
Cardiothoracic critical care units are staffed by highly skilled and specially trained multidisciplinary teams. Cardiothoracic anaesthetists and intensive care specialists work closely with surgeons, critical care nurses and other allied health professionals. Specific training in cardiothoracic critical care is now available via the Faculty of Intensive Care Medicine.
The aim of this chapter is firstly to help the perioperative practitioner understand early postoperative management of patients in cardiothoracic critical care and secondly to provide a systematic overview of other commonly encountered issues.
Early postoperative management
Most cardiothoracic critical care units have a rapid turnover of patients and the postoperative care of low-risk cases is a nurse-led, protocol-driven process. Over the past two decades, the management of patients following routine cardiac surgery has changed, with early extubation and rapid discharge allowing more efficient use of limited intensive care resources. These ‘fast-track’ protocols have become possible due to improvements in cardiac surgical and anaesthetic techniques, improved myocardial protection, cardiopulmonary bypass techniques and improved management of bleeding.
Anaesthesia for cardiac surgery traditionally involved high-dose opioid techniques, which contributed to prolonged ventilation postoperatively. ‘Fast-track’ cardiac anaesthesia employs a more balanced anaesthetic technique, using smaller doses of opioids and shorter-acting muscle relaxants, thus allowing extubation within 8 hours. Early extubation is thought to reduce postoperative atelectasis as well as improving haemodynamics by restoring normal negative intrathoracic pressure. ‘Fast-track’ protocols also facilitate early ambulation, return to normal diet and a reduction in postoperative complications. This has led to reduced critical care and shorter length of hospital stay as well as a reduction in healthcare costs (Zhu, Lee & Chee 2012).
‘Fast-track’ cardiac surgery is not always successful. Predictors of failure of ‘fast-track’ protocols include:
• Impaired left ventricular function
• Previous cardiac surgery
• Extra cardiac arteriopathy
• Perioperative intra-aortic balloon pump
• Raised serum creatinine (>150mmol/l)
• Emergency and complex surgery
• Excessive postoperative bleeding
• Pre- and intra-operative use of inotropes
• Atrial arrhythmias (Constantinides et al. 2006).
Following surgery, the patient is transferred to the Intensive Care Unit (ICU), which should ideally be located close to the operating theatre. During transfer the patient should have continuous haemodynamic monitoring, and on arrival on the ICU there should be a comprehensive handover to the nursing and medical staff.
In the UK, most patients who have undergone cardiac surgery will be admitted to the ICU sedated, intubated and ventilated. This allows a period of observation to ensure that the patient is cardiovascularly stable and not bleeding excessively.
The aims of early postoperative management are:
• Adequate analgesia
• Normothermia
• Adequate oxygenation and ventilation
• Management of excessive bleeding
• Optimisation of haemodynamics
• Correction of electrolyte and metabolic derangements
• Control of arrhythmias.
Adequate analgesia
With the move to ‘fast-track’ anaesthesia and the use of smaller doses of shorter-acting opioids, analgesia needs to be administered early in the postoperative period. Adequate analgesia is extremely important, following cardiac surgery, to reduce the risk of pulmonary and cardiovascular complications. There is evidence that patients with higher pain scores have a higher incidence of atelectasis, myocardial ischaemia and arrhythmias (Ziyaeifard, Azarfarin & Golzar 2014).
The use of shorter-acting analgesics (such as fentanyl, alfentanil and remifentanil) can facilitate early extubation. In our Institution, we use an infusion of morphine or alfentanil started intra-operatively. Alfentanil is the analgesic of choice if the patient has preoperative renal dysfunction, or if they are at an advanced age or prolonged sedation is anticipated. Following extubation, the infusion is discontinued, and oral multimodal analgesia is administered in the form of regular (and as required) oxycodone, paracetamol and gabapentin.
Side effects of intravenous opioids include nausea, vomiting, respiratory depression and somnolence; these are potential barriers to ‘fast-tracking’. Patient-controlled analgesia (PCA) can be useful for some patients as it provides good analgesia as well as improved patient autonomy and reduced anxiety. PCAs have also been demonstrated to reduce sedation and respiratory depression. The major disadvantage of PCA is a drop in blood analgesic concentrations while the patient is asleep.
Non-steroidal anti-inflammatory drugs (NSAIDs) can be useful adjuncts in managing pain following cardiac surgery, as they improve pain scores and reduce opioid consumption and side effects. However, they should be used with caution, and are often avoided, due to the risk of acute kidney injury, upper gastrointestinal haemorrhage and platelet dysfunction.
Hypothermia
Hypothermia is common following cardiac surgery and is due to vasodilatation, impaired thermoregulation, low ambient theatre temperatures and poor peripheral perfusion.
Hypothermia may result in:
• Delayed extubation
• Increased risk of ventricular arrhythmias
• Increased systemic vascular resistance, which increases afterload and myocardial workload
• Shivering, resulting in increased oxygen consumption
• Impaired platelet function and coagulation cascade function.
The risk of hypothermia can be reduced by utilising forced-air warming blankets, heat and moisture exchangers on ventilator circuits and warmed intravenous fluids.
Adequate oxygenation and ventilation
The aim of ventilation in the early postoperative period is to restore the functional residual capacity (FRC) by using lung protective ventilation and adequate levels of positive end-expiratory pressure (PEEP), which reduces shunt and improves gas exchange. Initial ventilation parameters should be tidal volumes of 6–8ml/kg predicted body weight, respiratory rate 10–15/minute, minimum of 5cmH2O PEEP and fraction of inspired oxygen of 0.5, aiming for PO2 greater than 8–10kPa and PCO2 between 4.5 and 6kPa. All patients will require supplemental oxygen to prevent hypoxia following extubation.
• Adequate gas exchange on less than 50% oxygen
• pH 7.35–7.45
• PaO2 >8kPa.
• PaCO2 4.5–6 kPa.
• Oxygen saturations >95%.
• Normal electrolytes and metabolic status
• Haemodynamically stable
• Absence of excessive bleeding
• Temperature >36ºC
• Awake, alert and obeying commands
• Return of muscle strength and protective airway reflexes.
Pulmonary complications following cardiac surgery are common, with approximately 12% of patients experiencing an acute lung injury and about 1.5% requiring prolonged mechanical ventilation. These complications are associated with significant morbidity, mortality and increased length of stay.
Postoperative pulmonary complications are due to a combination of peri-operative and patient factors. Patients presenting for cardiothoracic surgery often have smoking-related lung diseases and may have poor cardiac function. Intra-operative factors, such as positive pressure ventilation and lung handling, lead to a reduced functional residual capacity and vital capacity and atelectasis. The systemic inflammatory response to surgery and cardiopulmonary bypass leads to increased intravascular and extravascular lung water from increased capillary leakage due to the systemic inflammatory response.
Postoperatively, an inability to cough and deep breathe, due to pain or weakness and poor mobility, also contribute to the inflammatory response. The resulting ventilation-perfusion mismatching and shunt leads to hypoxaemia. Atelectasis is the most common cause of hypoxaemia following cardiac surgery. Lung volumes are still significantly reduced up to a week following surgery.
Regular physiotherapy and incentive spirometry should be used to reduce atelectasis. I-Cough is a simple, multidisciplinary postoperative pulmonary care programme developed in Boston and has been shown to reduce pulmonary complications and re-intubation (Cassidy et al. 2013).
The i-Cough programme makes use of the following elements to prevent postoperative lung collapse:
• Incentive spirometry
• Coughing and deep breathing
• Oral hygiene
• Understanding, i.e. patient and staff education
• Getting out of bed at least three times each day
• Head of bed elevation (Cassidy et al. 2013).
Management of excessive bleeding
The key to avoiding excessive blood loss postoperatively is good surgical haemostasis. Excessive bleeding can be due to ‘surgical’ or ‘medical’ bleeding, or commonly a combination of the two. ‘Medical’ bleeding is secondary to coagulopathy such as defects in the coagulation cascade, platelet dysfunction, low fibrinogen or residual heparinisation. ‘Surgical’ bleeding requires a return to the operating theatre for re-exploration. Common sites of bleeding include vascular anastomoses, cannulation sites and small mediastinal arteries or veins.
The management of excessive postoperative bleeding will be discussed more thoroughly in the ‘Haematology’ section of this chapter (see p. 258).
Optimisation of haemodynamics
Following cardiac surgery, all patients require continuous haemodynamic monitoring to detect changes in blood pressure, intravascular volume status, heart rhythm and myocardial ischaemia.
The most common cause of hypotension following cardiac surgery is hypovolaemia. Other causes include vasodilatation, which is common following cardiopulmonary bypass, and low cardiac output state. More than 20% of patients develop myocardial dysfunction following cardiac surgery. This ‘post-cardiotomy heart failure’ results in hypotension and inadequate oxygen delivery to tissue. Volatile anaesthetics and levosimendan (calcium sensitiser) show promise as cardioprotective agents (Mebazaa et al. 2010).
Factors contributing to ‘post-cardiotomy heart failure’:
• Myocardial stunning
• Inadequate myocardial protection
• Reperfusion injury
• Myocardial ischaemia or infarction
• Incomplete revascularisation
• Metabolic derangement
• Mechanical or conduit issues
• Pulmonary hypertension and right ventricular failure.
Cardiac tamponade must be considered in patients with low cardiac output state following cardiac surgery. Beck’s triad of hypotension, distended neck veins and muffled heart sounds may be difficult to detect. Echocardiography can confirm but not rule out the diagnosis.
Less common causes of hypotension in the postoperative period include dynamic left ventricular outflow obstruction, which typically occurs after aortic valve surgery in patients with left ventricular hypertrophy, acute paravalvular leak and tension pneumothorax.
The aim of haemodynamic management is to ensure that there is adequate organ perfusion. Rapid assessment and diagnosis of the aetiology of hypotension is essential, followed by appropriate therapy, which may include fluid resuscitation, vasopressors, inotropes and insertion of an intra-aortic balloon pump.
Management of these patients requires a good understanding of cardiovascular physiology. Preload should be optimised, and an optimum heart rate can be achieved by atrioventricular pacing. Preload is the left ventricular end-diastolic volume and can be assessed by central venous pressure, pulmonary capillary occlusion pressure and by echocardiography. The Frank-Starling principle describes the relationship between preload and cardiac output. Optimisation of preload may involve increasing intravascular volume with fluids or decreasing it with diuretics or renal replacement therapy.
Fluid management post-cardiac surgery can be very challenging, due to altered haemodynamics and loss of fluid from the intravascular space caused by the systemic inflammatory response to cardiopulmonary bypass. If the patient has an optimum preload and normal or paced heart rhythm, the cause of the hypotension is impaired myocardial contractility, vasodilatation or both. Inotropic and vasopressor drugs may be used to optimise cardiac output and blood pressure. These measures will result in improved haemodynamics in most patients. However, in a small number of patients, some means of mechanical circulatory support may be indicated, such as an intra-aortic balloon pump or extracorporeal circulatory life support (see section in this chapter on ‘Life support systems’, p. 254).
Correction of electrolyte and metabolic abnormalities
The postoperative course for most patients following cardiac surgery is characterised by predictable physiological derangements due to fluid and electrolyte shifts and cardiopulmonary bypass (see Table 12.1).
Table 12.1: The correction of electrolyte and metabolic abnormalities postoperatively according to the fluid and electrolyte shifts of the patient
Hyperglycaemia is common postoperatively, either due to pre-existing diabetes or as a physiological stress response to surgery. Hyperglycaemia is associated with increased incidence of cardiovascular, renal and infectious complications. The aim of glycaemic control is to avoid both hyperglycaemia and hypoglycaemia, so glucose levels should be maintained between 6 and 10mmol/l.
Control of arrhythmias
Arrhythmias following cardiac surgery are common and frequently due to electrolyte abnormalities and conduction defects. Atrial fibrillation (AF) occurs in about 35% of patients following cardiac surgery, most commonly on the second or third postoperative day. AF can be due to electrolyte abnormalities, increased sympathetic tone, exogenous catecholamines, pericardial inflammation and surgical trauma.
Management of AF aims to either restore sinus rhythm or control ventricular rate. Strategies include:
• Correction of underlying pathology such as electrolyte abnormalities
• Fluid resuscitation if appropriate
• Magnesium sulphate
• Amiodarone
• D/C cardioversion
• Rate-controlling drugs such as beta-blockers, calcium channel blockers and digoxin.
AF increases the risk of embolic complications, particularly stroke, and the need for anticoagulation must be assessed if sinus rhythm is not rapidly restored. Early prophylactic beta-blocker use has been shown to reduce the incidence of AF and reduce critical care and length of hospital stay (Hillis et al. 2011).
The respiratory system
Postoperative pulmonary complications are discussed in the ‘Early postoperative management’ section of this chapter (see p. 239).
Acute respiratory distress syndrome
Acute respiratory distress syndrome (ARDS) is a syndrome of widespread inflammatory lung injury. ARDS affects up to 20% of patients following cardiac surgery and is associated with high mortality and increased length of hospital stay (Stephens, Shah & Whitman 2013).
Berlin definition of ARDS:
• Acute onset, i.e. within a week of an insult or change in respiratory symptoms
• Bilateral opacities consistent with pulmonary oedema on chest imaging
• PaO2/FiO2 less than or equal to 300mmHg with PEEP or continuous positive airway pressure (CPAP) at least 5cmH2O
• Not ‘fully explainable by cardiac failure or fluid overload’ (The ARDS Definition Task Force 2012).
The severity of ARDS is categorised by degree of hypoxia, defined by PaO2/FiO2. Increased severity of ARDS is associated with increased mortality:
• Mild – 200 to 300mmHg
• Moderate – 100 to 200mmHg
• Severe – less than 100mmHg.
Lung-protective ventilation aims to minimise ventilator-associated lung injury and is proven to reduce mortality in ARDS (Kimura et al. 2016). Low tidal volumes (6ml/kg predicted body weight) are used and plateau pressures above 30cmH2O avoided. Lower than normal oxygen saturations may be targeted and hypercapnia permitted. Higher PEEP and recruitment manoeuvres may also be useful in patients with moderate or severe ARDS.
Other strategies include neuromuscular blockade, which has been shown to reduce mortality and duration of mechanical ventilation when given in the first 48 hours to patients with PaO2/FiO2 <150mmHg, and conservative fluid management, which has been shown to reduce duration of mechanical ventilation though not mortality (Papazian, Forel & Gacouin 2010).
High-frequency-oscillation ventilation and inhaled nitric oxide may improve oxygenation but have not been shown to improve patient outcomes in ARDS. Patients with potentially reversible refractory hypoxia despite optimum conventional management should be considered for referral to an extracorporeal membrane oxygenation-capable centre.
Ventilator-associated pneumonia
Ventilator-associated pneumonia (VAP) is defined as pneumonia occurring more than 48 hours after initiation of mechanical ventilation. It is the most common healthcare-associated infection in intensive care and is associated with increased mortality, length of hospital stay and healthcare costs.
Diagnosing VAP is challenging because clinical, radiological and laboratory findings are often non-specific. Multiple diagnostic scoring systems are available, utilising varying criteria; however, no scoring system has both high sensitivity and specificity. In some countries, diagnosis is further complicated by the use of VAP rates as a quality indicator with financial penalties for its occurrence.
VAP is predominantly due to micro-aspiration of pathogens past the endotracheal cuff in patients who are relatively immunosuppressed as a result of their critical illness. Strategies to reduce the duration of endotracheal intubation, such as daily sedation holds, are therefore of utmost importance in preventing VAP. Other strategies include nursing the patient in a semi-recumbent position, using endotracheal tubes with subglottic suction ports, oral chlorhexidine and judicious use of stress ulcer prophylaxis.
Early-onset VAP (i.e. up to 4 days after intubation) is likely to be due to an antibiotic-sensitive bacterium. Later-onset VAP is more likely to be due to multi-drug resistant bacteria. Local antibiotic guidelines should be followed.
Non-invasive ventilation and high-flow nasal oxygen therapy
Non-invasive ventilation (NIV) is defined as ‘delivery of ventilatory support via the patient’s upper airway using a mask or similar device’. CPAP is used to improve oxygenation and is commonly used to treat cardiogenic pulmonary oedema. Bi-level ventilatory support is useful in managing patients with hypercapnic respiratory failure. NIV may be beneficial when used prophylactically in patients who have been extubated and are at high risk of re-intubation. The use of NIV to manage established post-extubation respiratory failure is not recommended, as it has been shown to delay re-intubation and increase mortality (Glover & Glossop 2015).
High-flow nasal oxygen has been shown to be as effective as NIV in patients developing acute respiratory failure or deemed to be at risk of developing acute respiratory failure following cardiothoracic surgery (Stéphan et al. 2015).
Tracheostomy
A tracheostomy is a surgical procedure to open the trachea via the neck, into which a tracheostomy tube is placed. In intensive care, a tracheostomy is usually performed to facilitate weaning from mechanical ventilation. Compared with an orotracheal tube, a tracheostomy tube is associated with reduced need for sedation and subsequent improvement in patients’ ability to communicate, reduced laryngeal injury and enhanced access for mouth care. While tracheostomy may be appropriate in some circumstances, it does not reduce mortality or length of hospital stay (Young et al. 2013).
Tracheostomy can be performed percutaneously or surgically. Percutaneous tracheostomy employs a Seldinger technique with serial dilatation, under bronchoscopic guidance. It is performed on the ICU by the ICU team, thus avoiding transfer to theatre. It is associated with less bleeding, infection and cosmetic deformity than the surgical technique. However, surgical tracheostomy is preferred for difficult cases. Percutaneous tracheostomy is relatively contraindicated in patients with impaired coagulation, reduced platelet function and anatomical abnormalities.
Tracheostomy is also associated with a number of serious complications. These include accidental dislodgement, blockage, significant bleeding and pneumothorax. Late complications include swallowing difficulties and tracheal stenosis. Long-term harm and death can occur because of tracheostomy-related complications.
The cardiovascular system
Advanced cardiovascular monitoring
Pulmonary artery (Swan-Ganz) catheters
Definition:
The pulmonary artery catheter (PAC) is a balloon-tipped, flow-directed catheter placed through the right heart into the pulmonary artery (see Figure 12.1).
Indications:
• Measurement of cardiac output by thermodilution
• Remains gold standard for cardiac output monitoring
• Measurement of mixed venous oxygen saturations
• Indicative of oxygen extraction by tissues and so used to estimate balance between oxygen supply and demand
• Central venous oxygen saturations, taken from the superior vena cava, are a possible alternative
• Measurement of right heart pressures and pulmonary artery and occlusion pressures
• Pulmonary artery and occlusion pressure (PAOP) is used to estimate left ventricular end diastolic pressure and preload
• PAOP only accurately estimates left ventricular end diastolic pressure if the PAC is correctly positioned and a number of preconditions are met, e.g. no significant mitral stenosis or regurgitation
• This may be part of work-up for cardiothoracic transplantation.
• Studies in the general ICU population, such as PAC-Man, have not demonstrated any mortality benefit with PAC use (Rajaram et al. 2013) and there are concerns about the potential for harm (see ‘complications’ below). They remain in use on an individual patient basis and in complex cardiac patients, particularly those with elevated pulmonary artery pressures and those at risk of right heart failure. They are still widely used in patients undergoing heart and lung transplantation.
Contraindications:
• Significant tricuspid or pulmonary valvular pathology, e.g. endocarditis or prosthetic valve
• Tumour or thrombotic material in right heart
• Coagulopathy.
Procedure:
• Full aseptic precautions must be maintained throughout
• Large-bore sheath inserted into a large central vein, usually the right internal jugular vein
• Pressure transducer attached to distal lumen
• Catheter slowly advanced with typical waveforms seen as it passes through the right heart
• Balloon near tip inflated once waveform confirms position in right ventricle
• Once the typical pulmonary artery occlusion wave is seen, the balloon is deflated; this is typically at 40–50cm from skin
• The position should also be confirmed on chest X-ray.
Complications:
• Arrhythmias and right bundle branch block
• Lung infarction
• Pulmonary artery rupture
• Injury related to insertion of large-bore sheath
• Damage to tricuspid and pulmonary valves
• Thromboembolism
• Mechanical complications such as knotting
• Infection.
The pulmonary artery catheter is floated from the superior vena cava, giving a central venous pressure (CVP) into the right atrium into the right ventricle, and then into the pulmonary artery where it is wedged to give the pulmonary capillary wedge pressure. Normal pressures: CVP/RA ranges from 5 to 15mmHg; RV 15–25/2–8mmHg; PA 15–25/8–15mmHg; PCWP 2–15mmHg.
Minimally invasive cardiac output monitors
Pulse contour analysis is carried out by, for example, Pulse Contour Cardiac Output and Lithium Dilution Cardiac Output.
These devices relate the arterial pressure waveform to stroke volume and systemic vascular resistance. Calibrated systems, which use lithium or thermodilution to measure cardiac output via the Fick principle, and uncalibrated systems are available. A high-quality arterial waveform is essential and arrhythmias are a potential source of error.
Oesophageal Doppler monitors
The velocity of red blood cells in the descending aorta is measured using Doppler ultrasound via a probe placed in the oesophagus. These devices use cadaveric normograms of aortic diameter and assume 70% of cardiac output is delivered to the descending aorta to estimate the stroke volume. An increase in stroke volume in response to a fluid bolus suggests that the patient is volume-responsive. Oesophageal Doppler monitors have been shown to have high validity for identifying changes in cardiac output in critically ill patients (Drummond & Murphy 2012). They are not suitable for use in awake patients.
Echocardiography
Echocardiography is very useful for assessing the cause of haemodynamic instability and determining volume status, as well as ventricular and valvular function. It can diagnose endocarditis and cardiac tamponade, though it cannot exclude tamponade. Transthoracic echocardiography (TTE) is a non-invasive technique that can be performed in an awake patient. However, positive pressure ventilation, recent cardiac surgery and obesity can make transthoracic imaging challenging. Transoesophageal echocardiography (TOE) can be useful in these circumstances and is superior to TTE for assessment of the mitral valve, left atrial appendage, aortic dissection and endocarditis. TOE requires sedation and has an associated risk of oesophageal perforation for 3 in 10,000 patients, as described in a major series (Hauser & Swanevelder 2018).
Obtaining and interpreting echocardiograph images requires knowledge, skill and experience. There is potential to harm patients if clinical decisions are based on incorrectly interpreted information. TOE accreditation is available via the British Society of Echocardiography and the Association of Cardiothoracic Anaesthetists.
Pharmacological support of the cardiovascular system
Inotropes
Inotropes improve myocardial contractility and can be used to augment oxygen delivery to tissues. Aside from levosimendan, all inotropic agents act by increasing cAMP and intra-cellular calcium concentrations, which increases myocardial oxygen consumption. Catecholamines exert their actions via adrenergic and dopaminergic receptors of the sympathetic nervous system (see Table 12.2).
Table 12.2: The pharmacological inotropic support that may needed for the cardiovascular system after cardiac surgery
Catecholamines | ||
Name and dose | Mechanism of action | Additional considerations |
Norepinephrine (noradrenaline) 0.02–0.2mcg kg-1/min-1 | Predominantly vasoconstriction via α1 Mild inotropy via ß1 | Increased pulmonary vascular resistance Reduced renal and splanchnic blood flow |
Epinephrine (adrenaline) 0.02–0.2mcg kg-1/min-1 | Inotropy via ß1 Dose-dependent effect on vasculature – vasodilatation via ß2 at low doses, vasoconstriction via α1 at high doses | Potent bronchodilatator Increased arrhythmias Causes lactic acidosis |
Dopamine 2.5–25mcg kg-1/min-1 | Inotropy via ß1 Vasoconstriction via a1 at higher doses | Increased renal and splanchnic blood flow via dopaminergic receptors at low doses but ‘renal dose’ dopamine does not protect patients from developing renal dysfunction (Bellomo et al. 2000) Increased arrhythmias compared with noradrenaline when used to treat septic shock (Patel et al. 2010) |
Dobutamine 0–20mcg kg-1/min-1 | Predominantly inotropy via ß1 Vasodilatation via ß2 | Tachycardia common |
Phosphodiesterase inhibitors, e.g. milrinone (dose 0.1–0.75mcg kg-1/min-1):
• Inodilators, i.e. positive inotropic effect and vasodilatation
• Positive lusitropic effects, i.e. they increase myocardial relaxation
• Useful in managing right heart failure due to reduced pulmonary vascular resistance and improved right heart ejection fraction
• Avoid in hypovolaemic patients
• Concomitant vasopressor use is often required.
Levosimendan:
• Novel calcium-sensitising agent
• Increases calcium binding to troponin C within the cardiac myocyte, so exerting a positive inotropic effect without increasing myocardial oxygen consumption
• Additional effects on ATP-sensitive potassium channels, causing vasodilatation
• Very prolonged duration of action compared to other inotropes – effects may persist for up to a week
• Significantly more expensive than other inotropes
• Not widely used in the UK.
Vasopressors:
• Noradrenaline is the most commonly used vasopressor (see above)
• Vasopressin (dose 0–4 IU per hour) is an endogenous hormone with effects on water regulation and vascular tone. Vasopressor effects are mediated via V1 receptors. It is thought to have favourable effects on pulmonary vascular resistance compared with norepinephrine, particularly in treatment of post-cardiopulmonary bypass vasoplegia
• Phenylephrine (dose 0.4-9mcg kg-1/min-1) is a pure vasoconstrictor, exerting effects via α1 agonism.
Vasodilators
Nitroglycerin and sodium nitroprusside (SNP) exert a vasodilatory effect via production of nitric oxide. SNP is highly unstable and must be protected from light. It is also associated with accumulation of cyanide ions. Inhaled nitric oxide is rapidly deactivated and so causes selective pulmonary vasodilatation.
Mechanical support of the cardiovascular system
The use of extracorporeal life support and ventricular assist devices to support the cardiovascular system is considered in ‘Extracorporeal life support’ section in this chapter (see p. 254).
Intra-aortic balloon pump
The intra-aortic balloon pump (IABP) is a counter-pulsation device that consists of a balloon-tipped catheter placed in the descending aorta, usually via the femoral artery. The balloon inflates in diastole to increase coronary artery perfusion and deflates in systole, reducing afterload and encouraging forward flow. The overall effect is to improve myocardial oxygen supply and reduce myocardial oxygen demand.
Indications:
IABP is used as a bridge to recovery or definitive treatment in patients with:
• Cardiogenic shock
• Ongoing myocardial ischaemia
• Structural complications of acute myocardial infarction.
• Aortic regurgitation
• Aortic dissection
• Severe peripheral vascular disease.
Procedure:
• Usually inserted by cardiothoracic surgeon or cardiologist
• Heparinisation considered but often contraindicated, e.g. in case of recent cardiac surgery
• Full aseptic precautions must be maintained throughout
• Inserted via femoral artery
• Tip of balloon positioned 1cm distal to origin of left subclavian artery, either using fluoroscopy or by measuring surface landmarks
• Balloon inflation and deflation is timed using electrocardiogram (ECG) or changes in aortic pressure
• If the balloon was inserted surgically, it should be removed in theatre
• If it was inserted percutaneously, pressure should be applied to the insertion site by a medical practitioner for 20 minutes after removal and a pressure dressing used for 4 hours after removal.
Complications:
• Failure
• Vascular injury, commonly to aorta or femoral artery
• Limb, visceral and spinal ischaemia
• Helium embolus due to balloon rupture
• Thrombosis.
The IABP is often employed in very high-risk patients with low cardiac output state. There is a paucity of high-level evidence to support its use.
Rhythm disturbance and pacing
Atrial fibrillation is discussed in the ‘Early postoperative management’ section of this chapter (see p. 239).
Ventricular tachycardia can be recognised by broad QRS complexes on ECG. They are commonly life-threatening and may degenerate to ventricular fibrillation. Myocardial ischaemia and tamponade may precipitate ventricular tachycardia and should be actively sought.
All patients undergoing cardiac surgery are at risk of bradycardia in the postoperative period. Correction of electrolyte abnormalities and withdrawal of rate-limiting drugs, such as beta-blockers, may be sufficient to manage bradycardia in some patients. Epicardial pacing wires are usually placed at the time of surgery and can be used to pace the patient. If epicardial wires are not present, then transcutaneous pacing (which requires sedation), and transvenous pacing are alternatives. Drugs that block the effect of the vagus nerve on the heart, such as atropine and glycopyrolate, may be effective. Isoprenaline (ß1 and ß2 agonist), epinephrine and dobutamine may also be used for their chronotropic actions.
Cardiac arrest after cardiac surgery
Cardiac arrest following cardiac surgery is not the same as cardiac arrest in the general population; the pathophysiology, environment, staff and equipment available are all different. The Cardiac Advanced Life Support course was developed with reference to these differences. Cardiac tamponade is a common cause of post-cardiac surgery cardiac arrest; re-sternotomy relieves the tamponade and should be undertaken early if other resuscitative measures are not successful. When managed appropriately, survival after cardiac arrest complicating cardiac surgery approaches 50% (Brand, McDonald & Dunning 2018).
Life support systems
Extracorporeal life support
Extracorporeal life support (ECLS) describes the temporary support of cardiac and/or respiratory function with the use of an external circuit, centrifugal pump and polypropylene hollow-fibre membrane oxygenator. If an oxygenator is part of the circuit to provide gas exchange, the term extracorporeal membrane oxygenation (ECMO) can also be used.
ECLS is not new; it uses technology from cardiopulmonary bypass to maintain tissue oxygenation. The successful use of ECMO was first described by Hill et al. (1972) for the management of severe respiratory failure in an adult, leading to worldwide interest in ECMO (Hill et al. 1972). This early success was not replicated; a randomised controlled trial comparing ECMO to conventional ventilation in severe ARDS found that survival was extremely low and there was no difference between the treatment and control groups (Zapol et al. 1979). Subsequent studies also failed to demonstrate a survival benefit, resulting in ECMO being limited to a small number of hospital units as a treatment of last resort (Gattinoni, Carlesso & Langer 2011). In 1989, the extracorporeal life support organisation was founded to maintain a registry of all cases of ECLS occurring worldwide. The registry includes devices used, complications and outcomes; it is a valuable resource, as there is limited evidence in this area.
ECLS involves draining large volumes of blood, which is passed through an oxygenator (to add oxygen and remove carbon dioxide) before being returned to the patient. There are two main types of ECMO – veno-arterial (VA) and veno-venous (VV). In VA ECMO, the blood is returned to an artery and provides both cardiac and respiratory support; whereas in VV ECMO, the blood is returned to a vein and only provides respiratory support.
Veno-venous ECMO
The use of VV ECMO increased dramatically in 2009 when the H1N1 influenza pandemic caused large numbers of young patients to develop severe ARDS and refractory hypoxaemia. In addition, in 2009, the CESAR trial was published in The Lancet. This was a randomised controlled trial comparing consideration for ECMO, versus optimal conventional management, where the primary end point was mortality or severe disability at 6 months. Outcomes were significantly better for the ECMO group, with 63% alive without severe disability at six months, compared to 47% in the conventional management group (Peek et al. 2009).
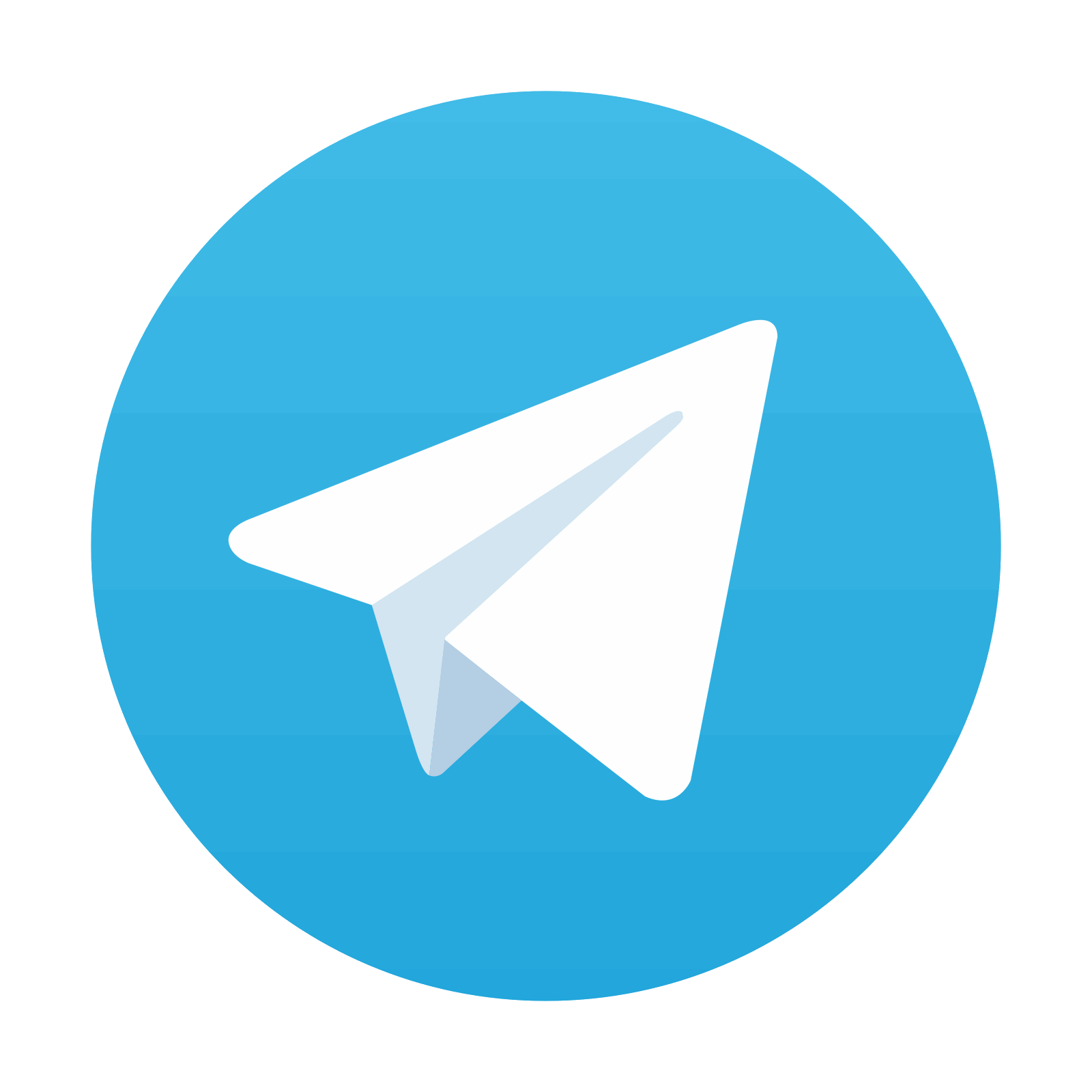
Stay updated, free articles. Join our Telegram channel

Full access? Get Clinical Tree
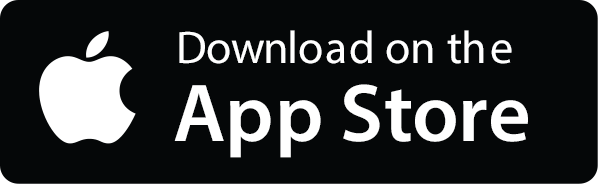
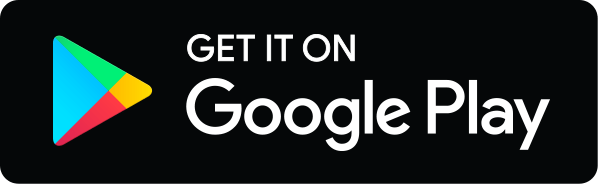