Introduction
Cardiopulmonary bypass (CPB) is used to take over the function of the heart and lungs during cardiac surgery. CPB is achieved by diverting blood away from the right side of the heart to a heart lung machine (HLM) where the blood is oxygenated and processed before being returned to the patient. Bypassing the circulation to the heart and lungs provides a still, bloodless surgical field for the surgeon to operate on the heart. The heart lung machine is operated by a perfusionist, who uses the HLM and its components to regulate the patient’s blood pressure and temperature to optimise perfusion and oxygenation of the patient’s body during CPB.
It is nearly 70 years since the first successful use of cardiopulmonary bypass. Surgical techniques may have fallen by the wayside, lost in the whirlwind development of cardiac surgery, nevertheless, the names of the innovators will live on in the history of heart surgery. Vision, knowledge and cooperation foster ideas that promote design, planning and financial backing. Innovative heart surgery is no different in its conception. A team of surgeons, anaesthetists, perfusionists and nursing staff will work together to develop a plan that is ideally rehearsed during a research phase. Each member of the team meticulously noting every minute step; each stitch, knot, infusion and cannula chosen explicitly.
History of cardiopulmonary bypass
In 1812, while Napoleon’s army was caught up in one of the most disastrous campaigns in military history, Julien Jean César Legallois was developing innovative approaches to organ preservation with oxygenated blood. He correctly prophesied that the human body could be kept alive using artificial extracorporeal blood perfusion methods (Bruce Fye 1995) and these ideas gradually evolved over the next one hundred years.
Toward the end of the 19th century, German physiologists experimented and progressed with early perfusion concepts. The first bubble oxygenator was produced by Von Schroeder in 1882, while in 1885 in Leipzig, Dr Von Frey and Dr Gruber developed a closed perfusion circuit with a rotating screen oxygenator, heater and artificial pump, along with aortic and venous cannulation in a canine model (Von Frey & Gruber 1985).
Cardiac surgery took a significant step on 9 September 1896. Dr Ludwig Rehn performed the first successful heart operation on a young man who had been almost fatally wounded by a stab wound to the right ventricle. Rehn performed the operation via the 4th intercostal space, placing the stitches during diastole (Werner et al. 2012).
As the 20th century dawned, vascular suturing techniques were advanced by Alexis Carell and Matieu Jaboulay, leading to the first animal renal transplant in 1902 (Barker & Markmann 2013).
In 1916 a young medical student, Jay Maclean, accidentally discovered the anticoagulant heparin while working with William J. Howell at Johns Hopkins Hospital in Baltimore USA, while experimenting with pro-coagulant compounds. However, heparin had many untoward side effects and it was not until the 1950s that it was synthesised in a form that was safe to be used during surgery (Shore-Lesserson & Gravelee 2000).
Heart surgery was nevertheless regarded as off-limits by the surgical hierarchy in the early 20th century although there are reports of successful procedures during the First World War (Ashcroft 2014). The 1920s saw experimentation in early mitral commissurotomy by the surgeon Henry Souttar.
In the 1930s John Gibbon and Carell published their separate ideas on the development of an extracorporeal device, but war slowed this development. Nevertheless, the Second World War provided an opportunity for new surgical heart procedures to be attempted. Between 1944 and 1945, Dr Dwight Harken performed 139 operations on the heart and great vessels to remove bullets and shrapnel. Thirteen of these operations were intra-cardiac; yet there was no mortality. This constituted a huge leap in the progress of heart surgery. Harken developed suturing and clamping techniques and aroused interest in the potential future of heart surgery.
After the war, and a fellowship with Mr Tudor Edwards at the Brompton Hospital, he returned to Boston, where he developed the mitral valvotomy operation. There he found himself competing with Dr Charles P. Bailey in Philadelphia, who was trying to develop the mitral commissurotomy at the same time.
Both procedures came under the umbrella of ‘closed heart surgery’ as they were performed without visualising the defects, but by inserting a finger or scalpel into the heart to open a stenosed mitral valve. An extracorporeal circulation was now needed to bypass the heart and lungs and provide oxygenated blood to the systemic circulation, to obtain a bloodless surgical field, to allow cardiac surgery to advance.
After the war the race to develop a heart lung machine recommenced. On 5 April 1951, at the University of Minnesota, Dr Clarence Dennis used a heart lung machine and disc oxygenator for the first time in medical history to attempt to repair an atrial septal defect (ASD) in a six-year-old girl. The procedure was unsuccessful (The United States National Library of Medicine 1927–2003).
On 3 July 1952, Dr Forrest Dodrill bypassed the left ventricle using ‘The Mechanical Heart’ Ventricular Assist Device (also known as the ‘Michigan Heart’) to perform the first mechanically assisted successful mitral valve repair on a 41-year-old male patient. The Dodrill-GMR heart project was a collaborative effort with General Motors Research Laboratory at Harper Hospital in Detroit, Michigan (The National Museum of American History 2018).
Dr Bill Bigelow suggested immersing patients in an ice bath to protect the body during periods of cardiac arrest while operating. This reduced oxygen demand and increased the time available to repair cardiac defects. Early operations were thus performed with inflow occlusion and hypothermia without cardiopulmonary bypass (Rimmer, Fok & Bashir 2014).
On 2 September 1952, at the University of Minnesota, Dr John Lewis repaired an ASD on a five-year-old girl using inflow occlusion under profound hypothermia. It took 2 hours and ten minutes to get the patient’s rectal temperature down to 28°C and 5.5 minutes to repair the ASD. She was rewarmed in a bath with water at 45°C for 35 minutes, by which time her temperature had risen to 36°C (Got 2005).
Cross circulation
Dr Lewis’s friend and competitor at the University of Minnesota during this period was Dr Walton Lillehei. He expanded the new era in heart surgery to repair VSDs and TOF and other anomalies in 1954 using cross circulation (Taha & Shehatha 2014) (see Figure 9.1). Critics of the procedure noted that this was the first time in the history of surgery that a procedure could carry a 200% mortality (Got 2005).
Figure 9.1: Controlled cross circulation, developed by C.W. Lillehei, used on 26 March 1954
Gibbon’s heart lung machine (see Figure 9.2) was built with the support of IBM. It was a screen oxygenator in which venous blood was spread thinly over wire-supported screens in a reservoir with a high oxygen gas flow. Oxygenated blood would pool at the bottom of the reservoir and be pumped into the patient. It was not an efficient way of oxygenating blood.
Figure 9.2: The Mayo Gibbon heart lung machine.
Further improvements to Gibbon’s machine were developed in the Kay and Cross machine (see Figure 9.3) (Cleveland Clinic) and Crafoord and Senning used a rotating disc oxygenator that provided less streaming and reduced the boundary layer but increased foaming and haemolysis (Stoney 2009).
Modern heart surgery really took off with developments by Richard DeWall and Dr Walter Lillehei at the University of Minnesota, where the first clinically viable bubble oxygenator was produced. On 13 May 1955 Dr Lillehei began routine heart surgery using the new device. This changed the course of heart surgery and perfusion as we know it. The device was efficient, cheap, sterilisable and easy to assemble and, when combined with new developments in plastics, it became possible to mass-produce it (DeWall 2003).
Interestingly, the main competition was between two of the great hospitals at this early stage in the history of CPB. The University of Minnesota Hospital and the Mayo Clinic were the only two hospitals in the world to use CPB. Competition became fierce as visitors from all over the world came to learn about heart surgery and the new bypass techniques. At the Mayo Clinic John Kirklin used the upgraded Mayo-Gibbon Machine, which was difficult to use and maintain. However, Kirklin’s success (with 9 out of 10 patients surviving) inspired teams from all over the world to start using CPB to support the circulation during heart surgery (Šušak et al. 2016).
The Rygg bag
Developments in bubble oxygenators by many manufacturers led to devices that were easy to use and disposable. The Rygg bag was one such device that was mainly used in Europe, with great success for many years. Bubble oxygenators eventually won the day (Hessel 2014). By 1976 it was estimated that 90% of cardiac surgery worldwide incorporated the use of a bubble oxygenator. There were many configurations of this type of oxygenator (Lim 2006).
Blood pumps
Gibbon and Lillehei first used Sigmamotor peristaltic pumps to propel blood in the bypass circuit. The roller pump, which is common today, soon superseded these pumps.
Figure 9.3: The centrifugal blood pump,
In 1973, the Biomedicus model 600 became the first disposable centrifugal pump for clinical use. The spinning cones create a negative pressure to draw blood into the inlet, creating a vortex. Centrifugal force imparts kinetic energy to the blood as the pump spins at 2000–4000rpm, spinning the three inner cones (see Figure 9.3). The energy produced in the cone creates pressure and blood is forced out of the outlet. It is preload- and afterload-dependent, with an inverse relationship between flow and resistance at a constant rpm (Safi et al. 2005).
Haemodilution
Bubble oxygenators and disc oxygenators were both used in the 1960s. However, until 1960, CPB circuit prime was composed of whole blood. At the time, this was thought to be the best thing. However, the use of whole blood proved to be a strain on blood banks and this led to haemodilution with crystalloid solutions. Remarkably, mortality rates dropped significantly. Blood usage and haemolysis were also reduced, improving capillary perfusion by the drop in viscosity.
Advent of the membrane oxygenator
It was noticed that membranes used for dialysis turned venous blood bright red so the search started for a highly permeable membrane material that could transfer oxygen and remove carbon dioxide. In 1956, Clowes et al. performed the first operation using a multi-layered ethyl-cellulose flat sheet membrane lung and later a Teflon membrane, which had hydrophobic properties to avoid the wetting-out phenomenon seen after blood protein leaks into the micropores of the membrane material.
However, CO2 removal was difficult due to the hydrophobic nature of the material. Problems with boundary layers and laminar flow caused oxygenation difficulties. Many designs were incorporated into the membranes to reduce the boundary layer and improve mixing by adding shims and cones to the blood path.
Silicone was the next compound to be explored as a diffusion membrane material, but it was not until 1968 that the silicone membrane was developed by Kolobow and used primarily in long-term support. Its gas permeability properties were far better than Teflon, though early trials produced pinhole leaks. This problem was overcome by Burns et al. at the Hammersmith Hospital in 1969. In 1983 the Terumo Corporation produced the first microporous hollow-fibre membrane made from polypropylene (Lim 2006).
Landmark innovations since 1960
• In 1960 Starr performed the first aortic valve replacement with a prosthetic valve; and later the same year D. Harken performed the first mitral prosthetic valve replacement (Russo et al. 2017).
• The age of heart transplantation was approaching. In the early 1960s Norman Shumway at Stanford in California established the technique for transplantation as it is performed today.
• In 1966 De Bakey implanted the first mechanical left ventricle assist device – left atrial to axillary artery. On the tenth day the device was explanted. The patient survived for more than five years.
• In 1967 the first successful heart transplant was performed by Christiaan Barnard in Cape Town, South Africa. Barnard had spent years working with, and learning from, the great heart surgeons in the USA. They were not happy that he received all the press and fame. However, within a year 100 transplants had been performed worldwide.
• Adrian Kantrowitz (famous for his work on developing the intra-aortic balloon pump) performed the second heart transplant in New York. The patient died within 24 hours.
• By 1968 most units had abandoned heart transplantation because of the problems of rejection, but Shumway, Reitz and colleagues persisted at Stanford
• In 1969 Denton Cooley, one of De Bakey’s brightest students, implanted a totally artificial heart into Haskell Karp, a 47-year-old man in severe LV failure. He was on the urgent transplant list but without a suitable donor. Cooley implanted the device, which lasted three days until Mr Karp was transplanted. Unfortunately, Mr Karp died the next day from multiple organ failure.
• In 1969 CABG surgery took a step forward with Favalaro at the Cleveland Clinic and Dudley Johnson in Milwaukee both advocating saphenous vein grafting using CPB. CPB allows improved vision by reducing the quantity of blood in the operating field.
• Use of auto-transfusion devices developed during the Vietnam War.
• The first reported successful use of ECMO in a case of neonatal respiratory failure took place in 1976.
• Blood cardioplegia became popular after the work done by Follette and Buckberg in 1978.
• In 1981 Shumway and his team, including John Wallwork, developed the heart and lung transplantation operation using a combination of cyclosporine and azothioprine. (Sir Roy Calne at Cambridge had done much of the ground work with cyclosporine A in 1977.) Their first patient was successfully transplanted and survived for more than five years.
• In 1982, in Louisville, Kentucky, William De Vries implanted the Jarvik 7 into Barney Clark, a 61-year-old dentist. Robert Jarvik had worked with De Bakey in Texas but had his own ideas on a totally artificial heart. Barney Clark survived for 112 days.
• In 1983 Terumo produce the first microporous hollow-fibre membrane made from polypropylene. The Cobe membrane lung used the z- shaped sheet membrane configuration.
• In 1986 John Wallwork and Sir Roy Calne performed a triple heart-lung-liver bypass in a 35-year-old woman.
• The first domino transplant was performed in 1987 in Baltimore, USA.
• In 1991, in Houston, Texas, the first TCI Heartmate was implanted.
Landmark innovations in the 21st century
• Membrane oxygenators have now become standard in CPB, as many studies have shown the adverse effects of bubble oxygenators.
• The adverse effects of CPB were addressed in the 1990s, and perfusion technique has improved with:
• Better in-line blood gas monitoring
• Heparin bonded circuits that reduce complement activation and preserve platelets
• Leukocyte filtration that is incorporated into the arterial filter as research into oxygen-free radicals has shown that they are generated from activated neutrophils
• Wide use of Trasylol as a tool to preserve platelets and reduce the inflammatory response to CPB
• The development of a new membrane material polymethylpentane that is used in ECMO
• The development of the pulmonary thrombo-embolectomy operation using deep hypothermic circulatory arrest with cerebral perfusion
• The development of the Transmedics Organ Care System that enables transport of the heart ex vivo for human transplantation
• The manufacture of mini-bypass systems that reduce blood product use and haemodilution
The use of bypass has been incorporated in other areas of surgery, including:
• Delivery of limb and hepatic anti-cancer agents
• Neurosurgery, to bypass and repair cerebral aneurysms
• Hyperthermia, in the treatment of human immunodeficiency virus
• Support during liver transplantation
• Repair of aortic aneurysms and dissections.
Looking ahead, cardiopulmonary bypass will continue to be an important tool in the advancement of heart surgery, transplantation and mechanical assist devices. The development of gene modification and stem cell engineering may change the treatment of heart disease, and transplanting bioengineered hearts may become commonplace. However, whatever the future holds, cardiopulmonary bypass, and the unsung skills of the perfusionists who operate them, will definitely be needed for many years to come.
The heart and lung machine
Figure 9.4: A heart lung machine with a basic CPB circuit mounted and heater-cooler connected
Cardiopulmonary bypass is used to divert blood away from the heart and lungs during cardiac surgery. CPB is achieved by connecting the patient to a disposable circuit consisting of polyvinyl chloride tubing, a reservoir, a heat exchanger and an oxygenator. The CPB circuit is mounted on a heart lung machine (HLM), which is a mobile base unit with several pumps in various configurations (see Figure 9.4).
Basic circuits
A basic cardiopulmonary bypass system is shown in Figure 9.5. A venous cannula is placed at the inflow tract to the right atrium and an arterial cannula inserted into the aorta or femoral artery. The cannula is then connected to the CPB circuit which has been primed with fluid to remove all air from the circuit. Blood is diverted away from the right side of the heart into a venous cardiotomy reservoir. From the venous cardiotomy reservoir blood is pumped through a heat exchanger, for regulation of blood temperature, and oxygenator, where the blood is oxygenated and carbon dioxide removed, before being returned to the patient via the arterial cannula.
Tubing circuit
The PVC tubing is used to connect the components of the CPB circuit. The internal diameter of the tubing used is determined by the required flow and pressure at different points in the circuit. The PVC tubing is often surface-coated with various materials, depending on the commercial supplier, to make the tubing more biocompatible to reduce the systemic inflammatory response caused by exposure of the blood to foreign surfaces.
Figure 9.5: Schematic of a basic cardiopulmonary bypass circuit.
For the majority of cardiac surgical procedures, a two- or three-stage venous cannula is used. The cannula is inserted through an incision in the right atrium and positioned so that the hole at the tip of the cannula drains blood from the inferior vena cava (IVC) and the remaining holes drain blood from the right atrium and the superior vena cava (SVC). For procedures that require the chambers of the heart to be opened, bicaval venous cannulation is used where separate single stage cannulas are placed in the SVC and IVC. Femoral venous cannulation can also be achieved by extending a long venous cannula from the femoral vein to the venae cavae. The size of cannula used depends on the size of the patient and the required flow rate.
Arterial cannulation
The arterial cannula is connected to the CPB circuit to return oxygenated blood back to the patient. For routine cardiac surgery, the normal site for arterial cannulation is the ascending aorta. The size and type of arterial cannula used depends on the height and weight of the patient and the required flow rate.
Cardiotomy reservoir
Venous blood from the patient drains into the cardiotomy reservoir, either by gravity or with the assistance of a vacuum applied to the reservoir. The cardiotomy reservoir can be either an open hard-shell reservoir or a closed soft-shell reservoir. Hard-shell reservoirs contain a polyester depth filter and a polyurethane de-foamer for the removal of debris and de-foaming of the blood. Suction blood and vent blood from the surgical field passes through the reservoir filters before being returned to the circulation. Some hard-shell reservoirs have two separate chambers, which allow the separation of activated suction blood. The level of fluid in the reservoir is maintained at a safe level during CPB to prevent accidental delivery of air to the patient. The cardiotomy reservoir is the point of access to the circuit where fluids and drugs can easily be added to the circulation.
Main pump head
The main pump head pumps blood from the cardiotomy reservoir to the oxygenator and back to the patient, effectively taking over the function of the heart in the CPB circuit. The main pump head is either a roller (peristaltic) pump or a centrifugal pump, depending on local practice.
Oxygenator
The oxygenator takes over the function of the lungs in the CPB circuit. Modern membrane oxygenators are made from bundles of microporous polypropylene hollow fibres. The gas phase is on the inside of the fibres and the blood flows through the bundle of fibres. Gas exchange (oxygen delivery and carbon dioxide removal) occurs through the micropores in the membrane. When blood comes into contact with the microporous membrane, a layer of serum proteins covers the micropores, forming a membrane and preventing a direct blood-gas interface.
The surface area for gas exchange in artificial lungs is considerably smaller than that of the natural lung; 1.4–2.2m2 compared with an alveolar surface area of about 70–100m2 for the average adult human. The relative efficiency of the artificial lung, compared to a mammalian lung, is achieved by the continuous flow of gas across the gas exchange surface (similar in function to a bird lung or dinosaur lung) which allows for larger concentration gradients for oxygen (O2) and CO2 between the blood and gas phase. Also, the configuration of the hollow-fibre bundle is designed to optimise the proximity of the blood to the gas exchange surface. After protracted use (>6 hours), plasma leakage can occur through the hollow-fibre micropores, causing a progressive reduction in gas exchange efficiency and eventually making it necessary to change the oxygenator.
The gas supply to the membrane oxygenator is provided through a gas blender and flow meter which is connected to piped oxygen and air. The perfusionist optimises oxygenation and CO2 removal by regulating the fraction of oxygen in the gas mixture and flow rate (l/min) of the gas across the oxygenator membrane. An oxygen analyser is used to continuously measure the oxygen concentration in the gas supply line and alert the perfusionist in the event of an interruption in oxygen supply. A vaporiser can be incorporated into the gas supply to administer volatile anaesthetics, and waste gases can be scavenged from the oxygenator outlet port.
Heat exchanger
The heat exchanger in the CPB circuit is used to regulate the patient’s body temperature. In most circuits the heat exchanger is integrated into the oxygenator unit and is connected to an external heater-cooler which pumps temperature-controlled water through the heat exchanger. Blood and water in the heat exchanger are separated by a polypropylene or stainless-steel surface which facilitates efficient heat transfer between the blood and water. The temperature of the water from the heater-cooler is precisely controlled, which allows careful regulation of the patient’s blood and body temperature.
Arterial line filter
Arterial line filters are usually 40μM screen filters inserted in the arterial line, or integrated with the oxygenator/heat exchanger, to remove particulate micro-emboli and air emboli before the blood is returned to the patient.
Suckers and vents
Suction pumps are used to salvage blood from the surgical field and return it to the circulation. Suction blood can either be returned directly to the circulation via the venous reservoir or collected in a separate reservoir and processed by a cell saver before being returned to the patient. Suction pumps are also used to actively vent excess blood from the heart and lungs during CPB.
Blood cardioplegia devices
Cardioplegia solutions are used to stop the heart during cardiac surgery. This enables the surgeon to operate on a motionless heart and also reduces the metabolic demands of the heart. Most cardioplegia solutions contain a high concentration of potassium (to stop the heart) and are mixed with blood. Blood cardioplegia devices are used to mix the cardioplegia solution with blood from the CPB circuit, which is then warmed or cooled before being delivered to the heart.
Numerous safety devices are connected to the bypass circuit to protect the patient and facilitate the smooth running of the CPB. The safety devices are interfaced with the control system of the heart lung machine and will either provide an audible alarm to alert the perfusionist or, if critical, will regulate the pump flow or stop the bypass. Bypass is terminated either by stopping the main pump head, when using a roller pump, or by applying an automatic servo-driven clamp to the arterial line when using a centrifugal pump.
Positive and negative pressures
Pressures are measured at various critical points in the circuit. Excessive negative or positive pressures will either regulate or stop pump flow in the pump, generating the measured pressure.
Bubble sensors
Bubble sensors are connected to tubing in the circuit and they detect bubble activity in the blood flowing through. Bubble sensors can alert the perfusionist to increased microbubble activity or they will stop the associated pump if a bubble of critical size is detected. An active bubble sensor should always be positioned on the arterial line to protect the patient from air embolus.
Level sensors
Level sensors are applied to external surfaces in the CPB circuit and they detect the presence or absence of a fluid behind them. A level sensor on the cardiotomy reservoir is essential and will stop the bypass if the level in the reservoir falls below the set point.
Temperature alarms
Temperature probes are used to measure patient blood temperature and will alert the perfusionist when set limits are exceeded.
Gas line monitoring
An oxygen analyser is used to continuously measure the oxygen concentration in the gas supply line to the oxygenator and will alert the perfusionist in the event of an interruption in oxygen supply.
Monitoring during cardiopulmonary bypass
Patient parameters (arterial blood pressure, central venous pressure, electrocardiogram and temperature are continuously monitored) and should be visible to the perfusionist during CPB. Urine output is also recorded at regular intervals.
Blood gas analysis
Regular blood samples are taken during CPB and sent for blood gas and electrolyte analysis using a blood gas analyser. Various in-line monitoring systems are available, which can provide continuous blood gas and electrolyte measurement during CPB. The accuracy and range of in-line parameters measured varies by device, and the choice of system used in different departments is largely driven by cost.
The exhaust from the oxygenator can be connected to an anaesthetic monitor to provide an estimate of volatile anaesthetic gas delivery.
Cerebral saturation monitoring
Regional oxygen saturation can be measured in the brain using near-infrared spectroscopy (NIRS). NIRS measures changes in oxygen supply and demand in the brain and a falling rSO2 index provides early warning of potential cerebral ischaemia. Measuring cerebral saturation during CPB is particularly useful when cerebral perfusion is compromised and it can also provide a surrogate indication of systemic perfusion quality.
Management of bypass
Clinical perfusion practice can vary quite significantly between surgical units and individual perfusionists and is largely determined by the experience and knowledge of the perfusionist and local protocols. This section provides an overview of the basic principles common to the management of routine cardiopulmonary bypass and is not intended to provide a detailed discussion of individual perfusion practice.
Heart and lung machine preparation and circuit priming
The heart lung machine (HLM) is thoroughly checked by the perfusionist before setting up the bypass circuit. The configuration of the bypass circuit used will depend on the type of surgical procedure, the height and weight of the patient and local protocols. Other factors (such as the patient having a metal allergy) could also influence the selection of various components within the circuit.
When the bypass circuit has been mounted on the HLM, the integrity of the water to blood barrier in the circuit heat exchangers is checked – either by applying a pressure to the heat exchanger compartment and checking for a fall in pressure or by connecting the water from the heater-cooler and checking for water leaks. The CPB circuit is then primed with fluid, which is recirculated through the circuit, and all air is meticulously removed from the tubing and components by the perfusionist.
The optimum solution for priming the CPB circuit remains a matter for debate but it is usually best to use a pure crystalloid solution, or a mixture of crystalloid and colloid solutions, depending on local protocol. Heparin is added to the prime solution, which is thought to bind to the charged surfaces of the CPB circuit and provide protection from thrombus formation when blood enters the system.
Having primed and de-aired the circuit, the perfusionist sets the optimum occlusion of the roller pumps (main pump head, vent and suction pumps). Finally, the perfusionist checks that the gas supply is connected and that all safety devices (bubble detectors, pressure transducers, level sensors, temperature probes and gas line monitoring) are functioning and correctly allocated to the control system of the heart lung machine.
Pre-bypass check list
The use of a pre-bypass checklist is advocated by the European Board of Cardiovascular Perfusion and the American Society of Extracorporeal Technology. Most perfusion departments use a tick-box checklist to ensure that all necessary preparation of the CPB system has been completed correctly and that back-up equipment is available if needed. An example of a pre-bypass checklist is shown in Figure 9.6.
Figure 9.6: Example of a pre-bypass checklist
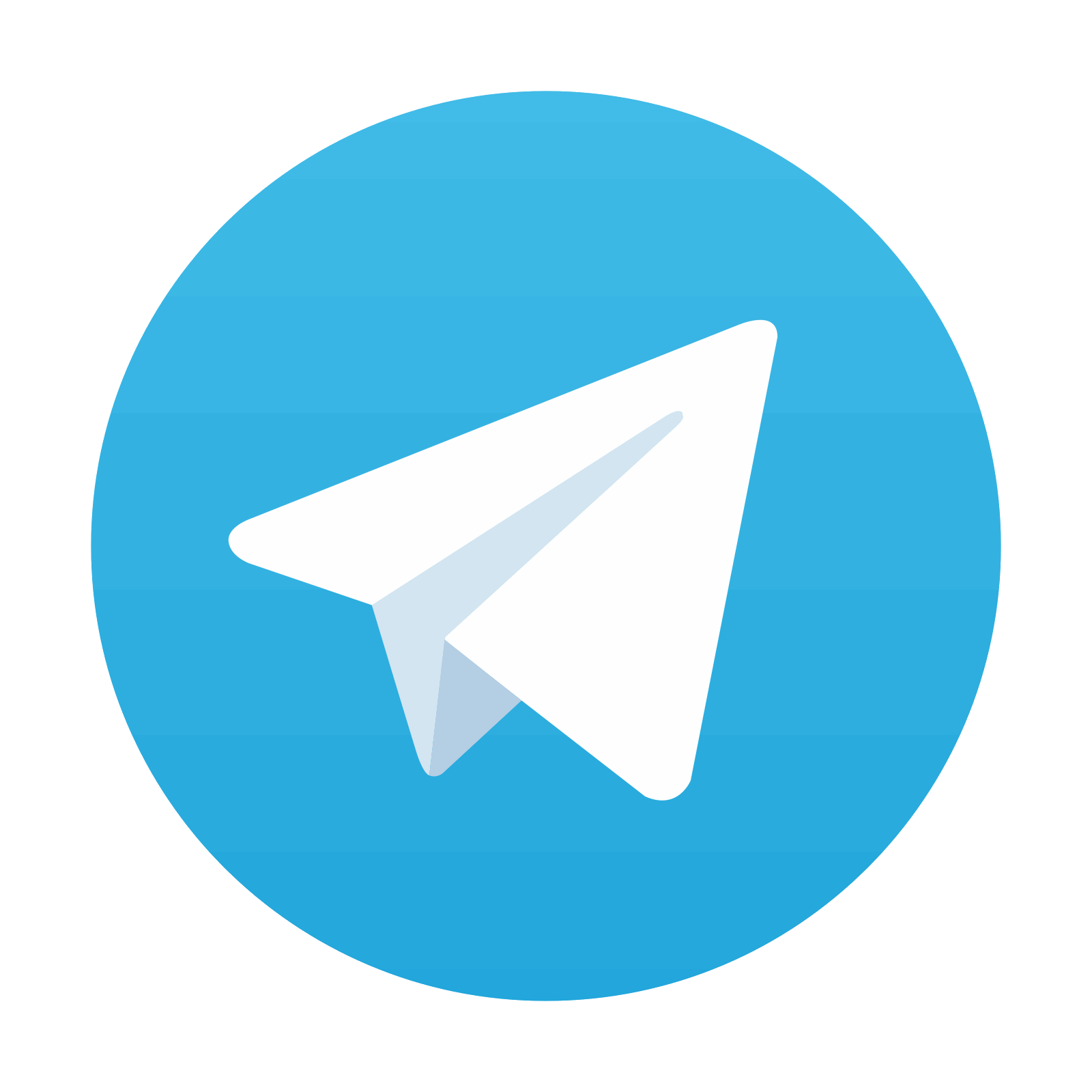
Stay updated, free articles. Join our Telegram channel

Full access? Get Clinical Tree
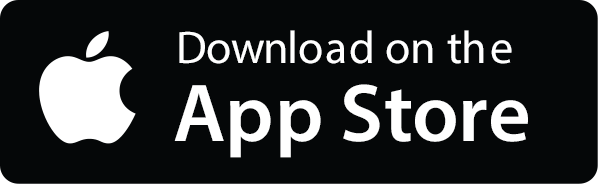
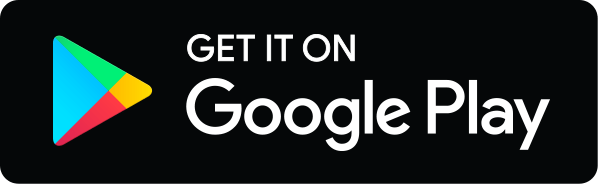