Fig. 7.1
Role of hypothalamic neuroinflammation in metabolic disorders. In the context of chronic overnutrition, there is an activation of the IKKβ/NF-κB pro-inflammatory pathway, mainly produced by an oxidative stress, by an endoplasmic reticulum (ER) stress, and by a defective autophagy machinery. Glia-neuron inflammatory cross talk is also relevant, and these abnormalities, collectively, lead to hypothalamic neuronal dysfunction and to the development of a spectrum of metabolic disorders
Neurodegeneration and Neurogenesis in Metabolic Syndrome
The relevance of the hypothalamus in the development of MetS, for all that has been said, is unquestionable. Indeed, classical studies showed that the ablation of some ventral nuclei causes overeating and obesity in animals, while lesions produced at a more lateral level lead to anorexia and weight loss. Although scientifically relevant, these data do not explain the pathophysiology and the mechanisms implicated in clinical outcomes. However, recent studies have been showing a connection between neurodegeneration and the development of metabolic disturbances, such as T2D and obesity [49]: in the first place, using adult mice fed with a high-fat diet, it was possible to show a reduction in the number of hypothalamic POMC neurons [49]; and second, chronic overnutrition was shown to be responsible for increasing apoptosis of mature and newborn neurons and neural stem cells in the hypothalamus, this situation being to some extent reversed by caloric restriction [49, 50]. This is especially interesting, taking into account that studies on postnatal hypothalamic development have been able to show that neurons in the ARC undergo postnatal turnover, even in adulthood [50]. Real clinical implications of such an observation are still insufficiently studied, but these data are important to counteract the old dogma which looks at the CNS as a static system in which neuronal damage is absolutely irreversible and irreparable.
Inflammation seems to be the leading cause of hypothalamic neurodegeneration in MetS-related disorders, such as obesity and T2D, and this is a common background shared with typical neurodegenerative diseases. As it was previously discussed, IKKβ/NF-κB pathway is critically involved in the development of hypothalamic damage in metabolic dysfunctions and in neurodegeneration itself, but there are several unanswered questions in this area that make it very attractive to future research. Indeed, the role of inflammation in promoting neurogenesis and repair in metabolic disturbances is not yet fully understood.
It is well established that adult mammalian brains contain multipotent neural stem cells that have the intrinsic ability to generate and to differentiate into several cell types in the CNS, from neurons to oligodendrocytes [51]. These adult stem cells might be able to be involved in adult neurogenesis and in maintaining brain plasticity in response to intrinsic and extrinsic stress. They are predominantly localized in the subventricular zone of the forebrain lateral ventricle and in the subgranular zone of the hippocampal dentate gyrus [52]. The research made in the last decade has proved that the hypothalamus of adult mice has neurogenic activity in basal conditions [53] and after stimulation [54]. More recently, it has been shown that, using a mouse model with genetically induced degeneration of neurons expressing AgRP, de novo hypothalamic neurogenesis exists, leading to the development of new leptin-responsive AgRP neurons [55]. In addition, neurons contained in the ARC of adult mice were demonstrated to have intrinsic physiological turnover activity [50], but it was also discovered that IKKβ/NF-κB pathway activation stimulates an apoptotic program impairing the survival of hypothalamic neural stem cells and that turnover capacity [49]. Thus, it is critical to note that IKKβ/NF-κB-mediated inflammation not only affects neurohormonal pathways and signaling axis in hypothalamic neurons, which are relevant for regulating metabolic physiology, but also hampers neurogenesis and repair mechanisms, leading to the development of metabolic diseases. All this knowledge has been raising a huge scientific interest in hypothalamic repair properties and mechanisms and, logically, in their role for the development of systemic metabolic disorders [56]. Consolidation of this knowledge may have a huge impact on future design of pharmacological and cellular therapies for MetS.
Therapeutic Applications
The concept of brain stress in MetS is a relatively recent establishment and, therefore, the amount of mechanistic studies conducted specifically for developing new potential therapeutic agents is still scarce. However, the recognition of the deleterious role of IKKβ/NF-κB pro-inflammatory pathway activation in hypothalamic cells has contributed to the design of some experiments showing that its inhibition effectively protects against metabolic disorders. Several strategies were used in animal models: (1) pharmacologic inhibition of hypothalamic IKKβ [57]; (2) brain-specific deletion of the same enzyme [20], of one of the signaling effectors of the IKKβ/NF-κB pathway, the SOCS3 protein (suppressor of cytokine signaling-3) [58], or of TLR4 signaling adaptor MyD88 [34]; and (3) whole-body genetic deficiency of TLR4 [33] or NF-κB subunit p50 [59]. Data coming from studies in humans are much more scarce, but there are some reports in the literature that it is worth to consider here. In a retrospective case-control study, acetylsalicylic acid used as an anti-inflammatory drug was shown to promote weight loss in T2D patients [60]. Also, rimonabant (a drug specifically developed for weight loss but already withdrawn from the market) was responsible for a systemic decrease of inflammatory response [61].
Improving endoplasmic reticulum stress can also constitute itself as a therapeutic target in MetS and that was achieved in obese mice by inducing genetic expression of some UPR components and chaperone proteins or administering a pharmacologic endoplasmic reticulum stress inhibitor, such as tauroursodeoxycholic acid (TUDCA) [62]. In fact, this organelle-specific therapy demonstrated to have some measurable biologic effects in humans. TUDCA was able to improve liver and muscle insulin sensitivity in obese subjects [63], and stavudine, a drug with antioxidant properties, was demonstrated to increase muscle insulin sensitivity in humans [64].
Finally, considering MetS-associated CVD, many clinical and epidemiological studies have been done to demonstrate the therapeutic potential of anti-cellular stress drugs. Vitamin E (which has a well-known antioxidant activity), coenzyme Q10, α-lipoic acid, and α-l-carnitine are some examples of substances acting as protectors of myocardial dysfunction, as oxidation-resistant low-density lipoprotein production enhancers and as systolic blood pressure improvers in patients with coronary heart disease [65]. It is known that overnutrition-related metabolic inflammation in the hypothalamus (particularly in POMC neurons) may contribute to the development of arterial hypertension, at least in obese mice [66]. However, this knowledge is so recent that it is still premature to speculate about any therapy which may have an antihypertensive activity and that potentially may have its mechanism of action contained in this pathway.
Indeed, despite the exciting discoveries that have been made over the past years, connecting metabolic inflammation with the brain (particularly with the hypothalamus) and with MetS-related conditions is still a great challenge and many important questions still remain to be answered and to find some support coming from experimental environments. Current understandings of the central inflammatory mechanisms that mediate MetS and its related disorders are still in a very early stage. And this is even more striking considering the possibility of therapeutic intervention on these dysfunctional mechanisms. However, this is a field of great clinical significance, and major research campaigns are being implemented to effectively translate this scientific knowledge into novel and effective treatments against MetS.
Systemic Metabolic Dysfunction in Neurodegenerative Disorders
Alzheimer’s Disease
Alzheimer’s disease (AD) is the most common cause of dementia. This neurodegenerative disorder has a characteristic neuropathology, which consists of senile plaques (aggregates of amyloid β [Aβ] protein surrounded by damaged neuronal processes and reactive glia) and neurofibrillary tangles (intraneuronal aggregates of hyperphosphorylated tau protein) leading to loss of synapses and neuronal death.
The neuroinflammatory processes underlying AD pathology have been the subject of research for the last years, with important advances in the understanding of the intricate interaction between CNS resident cells and the systemic immune response. Microglia has arisen as the main character involved in communication between local and systemic inflammation. The major regulator of this microglial priming is the composition of the CNS microenvironment. Local neuronal damage and accumulation of misfolded proteins (such as seen in AD) can reduce inhibition or directly activate microglia, which produce a maladaptive response and perpetuate neuronal death. Aging, the major risk factor for developing AD, is characterized by a chronic low-grade systemic inflammation appearing as a result of the decline in the function of the adaptive immune system and persistence of an intact innate immune system (upregulation of Th1 pro-inflammatory response and relative decline in Th2 anti-inflammatory response). With increasing age, microglia also adopts an activated phenotype.
ApoE4, the best established genetic risk factor for developing sporadic late-onset AD [67, 68], besides its important role in lipid transport [68], has also been associated with the degree of microglial activation in response to Aβ depositions. In AD, the blood-brain barrier (BBB) becomes leaky because of Aβ accumulation along the brain blood vessels (cerebral amyloid angiopathy), causing associated vascular inflammation and impaired clearance of Aβ across the BBB [69]. Acute (e.g., infection) and chronic systemic inflammation (e.g., atherosclerosis, obesity, and diabetes) have been held responsible for the microglial priming and polarization to a sustained neurodegenerative phenotype as well [70].
We will now refer to the systemic inflammatory and metabolic dysfunction that could perpetuate this neurodegenerative process within the CNS and briefly comment on novel therapies with an emphasis on modifiable lifestyle changes that could prevent cognitive impairment or improve cognitive performance.
A number of pro- and anti-inflammatory cytokines have been associated with AD and risk of overall dementia, including TNF-α, IL-1, IL-6, IL-7, IL-10, IL-15, and IL-18, although their relationship with plausible biological explanations are still matter of research. Acute inflammatory states (e.g., infection) have demonstrated the connection between circulating pro-inflammatory cytokines and the development of behavioral and psychological symptoms (“sickness illness”) among patients with AD, demonstrating that raised TNF-α and IL-6, but not C-reactive protein (CRP), were associated with an approximately twofold increased frequency of neuropsychiatric sickness behavior in AD patients, independent of the development of delirium [71]. On the other hand, one of the most studied chronic inflammatory states that have been associated with the risk of AD and other dementias is the MetS. Association between MetS and cognitive performance is dependent of age. In younger adults, MetS increases the risk of dementia, but in older adults over the age of 75, MetS is associated with better cognitive performance and reduced risk of dementia [72, 73].
When lipid consumption exceeds the metabolic capacity of macrophages, lipotoxicity and accumulation of metabolites within adipose and non-adipose tissue compartments such as oxidized LDL, free fatty acids (FFA), cholesterol, and ceramide, among others, induce chronic inflammation by promoting macrophage infiltration and activation [74, 75]. Activated macrophages produce excessive secretion of pro-inflammatory cytokines (e.g., TNF-α, IL-1β, monocyte chemoattractant protein-1 [MCP1], CRP), inducing insulin resistance by interfering with insulin/insulin-like growth factor 1 (IGF-1) signaling, causing increased circulating FFA and reduced glucose uptake. Insulin is one of the most consistent components of the MetS that could predict cognitive performance. In healthy controls, higher insulin levels predicted more rapid decline in attention and verbal memory over 2 years, in opposition to early AD patients in whom higher insulin levels predicted better cognitive performance [76]. Insulin affects neuronal performance and cognition by regulating ion channels, neurotransmitter receptors, and synaptic transmission in the AD-diseased brain [77]. It provides direct negative feedback to hypothalamic nuclei that control whole-body energy and glucose homeostasis, and dietary fat is able to induce hypothalamic inflammation resulting in insulin/leptin resistance [78]. Insulin dysregulation has also been associated to reduce brain glucose utilization, neurofibrillary tangle formation, and Aβ aggregation by insulin-degrading enzyme inhibition in AD patients [77]. Therefore, systemic inflammation and insulin resistance may represent convergent mechanisms through which comorbid metabolic disorders promote the development of AD [78].
There has been special interest in the role of obesity and adipokines and the risk of dementia. Obesity is also characterized by a chronic low-inflammatory state mediated by the production of IL-1 and IL-6. The hippocampus has been shown to be especially vulnerable to IL-6, affecting brain functions such as synaptic plasticity and neurogenesis, disrupting learning and memory processes. Higher levels of plasma IL-6 are also correlated with lower hippocampal gray matter volume. Adipokines or adipocytokines are signaling molecules secreted by peripheral white adipose tissue, and they have been associated with dysregulation of nutrient utilization, inflammation, endothelial dysfunction, hypertension, and atherogenesis and have also been held responsible for the link between obesity and dementia. For example, leptin has been shown to affect hypothalamic function, learning, and memory processes controlled by the hippocampus and to regulate energy expenditure and food intake [79], and adiponectin is involved in the modulation of inflammatory responses, decreasing the expression of inflammatory cytokines (TNF-α), increasing the expression of anti-inflammatory molecules (IL-10, IL-1 receptor antagonist), and decreasing activation of the pro-inflammatory NF-κB signal pathway. Adiponectin also modulates energy expenditure in the CNS (modifying sensitivity to insulin in the brain) and at the periphery, and it regulates food intake and several other metabolic processes. It modulates memory and cognitive impairment and contributes to the dysregulated glucose metabolism and mitochondrial dysfunction observed in AD [77].
Plasma cholesterol could also be a factor involved in the production of Aβ and in the development of neuropsychiatric symptoms in AD. Triglyceride levels have been correlated with depression [80], and it has been described a “longevity phenotype” characterized by large LDL and HDL serum levels, associated with homozygosity for a genetic variant of cholesterol ester transfer protein (CETP) and preservation of cognitive function [81]. Some authors have also correlated neuropsychiatric symptoms with biomarkers of cardiovascular risk (total cholesterol being the strongest marker in males) and with biomarkers of systemic inflammation (IL-15 being the strongest marker in women) [80].
All the aforementioned risk factors are common between AD and vascular dementia, and some data from large cardiovascular cohorts have shed some light on the impact of biomarkers of systemic inflammation in the development of AD and cognitive impairment. In the Rotterdam study, elderly subjects with higher levels of α1-antichymotrypsin and IL-6 had an increased risk of incident dementia and AD [82]. In the Framingham study, subjects with the highest production of IL-1β and TNF-α had an increased risk of developing incident AD [83]. Finally, in a population-based study, higher levels of baseline TNF-α and intermittent increases triggered by acute factors (like infection) were associated with a more rapid progression of cognitive decline [84].
Although there is a better understanding of the mechanisms underlying the association between systemic inflammation and neurodegeneration in AD, many therapeutic approaches have failed to demonstrate real benefits in preventing or slowing the progression of the disease. Long-term nonsteroidal anti-inflammatory drugs (NSAIDs) could have a protective effect against the development of AD, probably due to the regulation of cyclooxygenase-1 (COX-1) and cyclooxygenase-2 (COX-2) activity [85] or regulating microglial reactivity [86], although prospective clinical trials have failed to demonstrate therapeutic benefit with the use of naproxen, celecoxib, or rofecoxib [87].
In a recent meta-analysis exploring the benefit of statins in dementia, Wong et al. found that statin use could have a beneficial role in AD patients (RR: 0.70, 95 % CI [0.60, 0.83]) [88]. Some of this protective role could be in part secondary to lower levels of cholesterol, although their anti-inflammatory effect (activation of heme-oxygenase/biliverdin reductase system and inhibition of isoprenylation of small GTP-binding proteins) and other pleiotropic properties may also be involved [89].
Pilot trials with intranasal insulin administration suggested promising cognitive improvement and modulation of Aβ deposition in patients with mild cognitive impairment (MCI) and AD [90, 91]. Antioxidant therapy (e.g., coenzyme Q, vitamin E, vitamin C, and α-lipoid acid) has demonstrated significant decrease in cerebrospinal fluid F2 isoprostane (biomarker of oxidative damage) with no long-term clinical benefits. More recently, immunotherapy and inhibition of TNF-α is being investigated as a potential candidate for AD treatment. Some studies suggest that TNF-α haplotype could be a disease-modifier gene in patients genetically predisposed to AD, and there have been some reports of anti-TNF agents (e.g., infliximab, etanercept, pentoxifylline) that have shown some improvement in the cognitive performance of AD patients, although definitive clinical trials are still lacking [92].
Finally, diet and exercise are among the lifestyle changes associated with reducing biomarkers of systemic inflammation and showing promising results on neurodegeneration. High-calorie Western diet, rich in saturated fatty acids and cholesterol, is associated with the development of AD-like cognitive impairment [93]. Animal models suggest that such diets could cause increased amyloidosis and altered synaptic plasticity and behavior, and high-fat diet and excessive sucrose intake have also been shown to enhance tau pathology in mice [70]. On the contrary, many studies suggest that adherence to a Mediterranean diet may be associated with a reduced risk of developing AD. Some authors have found a correlation between diet and levels of blood inflammatory biomarkers, but others have failed to determine whether this protective factor is involved with inflammatory or metabolic pathways, probably due to different experimental and statistical approaches [94]. Exercise promotes better sleep and cardiorespiratory fitness, reduces obesity, and modulates cortisol levels. Aerobic exercise training has shown several benefits, such as the following: (1) to improve resting cerebrovascular reactivity to hypercapnia; (2) to reduce arterial pressure; (3) to decrease total cholesterol and triglycerides blood levels; (4) to lower body mass index (BMI); (5) to promote a better glycemic control; (6) to increase IGF-1 levels and regional brain volume in older adults, specifically at the hippocampus level; and (7) to promote brain neurogenesis and to reduce chronic low-grade inflammation (decreasing TNF-α and IL-6), and this could be a link between exercise and prevention of AD and overall dementia [95, 96].
In summary, although aging and apoE4 status appears to be the greatest and most studied and validated non-modifiable risk factors for developing sporadic AD, systemic inflammation and cardiometabolic risk factors are probably the link between AD and vascular dementia and could be a promising therapeutic target for novel treatment approaches and for early prevention strategies, including exercise and healthy lifestyle changes.
Multiple Sclerosis
Multiple sclerosis (MS) is a chronic immune-mediated disease characterized by acute and chronic inflammation within the CNS, associated to focal demyelination (MS plaques) and progressive neuroaxonal degeneration. It is the leading cause of nontraumatic neurological disability among young adults, and although the precise etiology is still unknown, genetic predisposition associated to environmental factors (low levels of vitamin D, smoking, infections) has been proposed to be the trigger of a pathogenic immune response against CNS antigens, developing and perpetuating MS distinct inflammatory processes [97, 98].
The complete understanding of the immunopathogenesis of the disease is out of the scope of this chapter, but, briefly, cellular and humoral components of the immune system are compromised in MS pathology: the innate immune system is involved in several steps, including the activation of myelin-reactive T lymphocytes by antigen-presenting cells (including B lymphocytes) at the periphery and the development of membrane attack complexes within the CNS; the adaptive immune system is also affected, where T cells that specifically recognize myelin fragments induce tissue damage and contribute to lesion formation. Active MS plaques are characterized by T cell, macrophage and activated microglial infiltration, and the presence of immune mediators, including adhesion molecules, chemokines, and cytokines [99], which are responsible for generating BBB leakage, destruction of myelin sheaths, oligodendrocyte damage and cell death, glial scar formation, inflammatory infiltrates and axonal damage, and, at the end, neuronal loss [100]. Neuronal and axonal degeneration in MS is a slow process that is probably initiated by acute lymphocytic infiltration and subsequently driven by chronically smoldering and diffuse parenchymal myeloid and meningeal lymphocytic inflammation [97]. As in AD, the microglia also plays a key role in the inflammation-induced neurodegeneration occurring during both acute and chronic stages of MS. These cells have been associated to the formation of cortical lesions in MS, and they also play an important role in the promotion of neuroprotection, downregulation of inflammation, and stimulation of tissue repair. As explained above, the microglia undergoes changes in morphology and function with normal aging, resulting in a decline of its ability to repair CNS damage, making axons and neurons more vulnerable as people grow older [101]. The microglia is also known to be affected by acute and chronic systemic inflammation, responding to circulating cytokines and producing immune mediators that lead to tissue damage, which prolongs the neurodegenerative-perpetuating phenotype of these cells [102].
Although local persistent inflammation within the CNS (e.g., lymphoid leptomeningeal follicles) is probably associated with sustained disability, systemic chronic inflammation has also been held responsible for the progression of neurodegenerative processes among these patients, with a new target for novel therapeutic interventions waiting to be confirmed. Furthermore, the presence of systemic inflammation and increased cardiometabolic risk among MS patients has also been a motive of research. We will now refer to the biomarkers of cardiometabolic risk and inflammation that have been associated with this disease.
In a study of 833 clinically stable patients, Martins et al. found differences in serum Th1 cytokine concentrations (increased IFN-γ and IL-2) and monokines (an increase in TNF-α and IL-1β and a decrease in IL-8, a neutrophil attractant) and no differences in IL-6 levels between MS patients and healthy controls. Anti-inflammatory Th2 cytokine levels were also found to be different (higher levels of IL-4 and IL-13 in MS patients, with no differences in IL-5 concentrations) and these changes were not related to the MS clinical phenotype. The increase of both pro- and anti-inflammatory cytokines in patients with MS seems to be consistent with the simultaneous inflammatory and restorative processes underlying the disease [99].
Chronic inflammation is a relevant factor for the development of atherosclerosis, contributing to the increased risk of overall vascular risk and added disability among patients with MS. There have been contradictory findings when evaluating vascular risk in patients with MS, although it seems that these patients are in higher risk of developing vascular disease than the general population [103]. In a cohort of male veterans with MS, the subset of people aged 50 years or older was found to be at higher risk of developing T2D, hypertension, hypercholesterolemia, coronary heart disease, and stroke than general population [104]. Other studies report higher risks of stroke and cerebrovascular disease, especially during the first years after MS diagnosis and among young and middle-aged patients and increased risk of venous thromboembolism, including deep vein thrombosis and pulmonary embolism, but lower prevalence of coronary artery disease including myocardial infarction [105], with special attention in women [106]. In 2010, Marrie et al. showed that vascular comorbidities were present in more than 50 % of patients (37 % had hypercholesterolemia, 30 % arterial hypertension, 7 % heart disease, 6 % T2D, and 2 % peripheral vascular disease). Vascular comorbidities at any time during the disease course were associated with a 1.5-fold increase of the risk of ambulatory disability [107].
Serum lipid levels’ dysregulation has been described to be present among MS patients, who frequently show increased levels of triglycerides and oxidized LDL and reduced concentrations of HDL, with an interesting correlation between oxidized-LDL levels and the expanded disability status scale (EDSS) score, as reported by Palavra et al. [108]. The North American Research Committee on Multiple Sclerosis (NARCOMS) registry study reported that 37 % of patients with MS suffered from hypercholesterolemia, which was a higher value than that coming from the general population [103], although some studies reported figures with a much lower prevalence. The brain is believed to be particularly susceptible to lipid dysregulation and oxidative damage, due to its composition with high content of lipids and high oxygen consumption [109]. Associations between HDL or triglyceride levels and brain contrast-enhancing lesions in clinically isolated syndrome (CIS) or early MS patients have also been found [110], but further research is needed to confirm these data.
In this matter, statins have emerged in the MS field, calling attention to their pleiotropic immunomodulatory and anti-inflammatory effects, independent of their effect on lipid profile. Although several clinical trials have taken place testing the role of statins as monotherapy or in combination with disease-modifying therapies, there have been some conflicting results (summarized in a recent meta-analysis by Ciurleo et al.) [111]. This is probably in part because of diverse drug regimens and different methodological and statistical models used, including results with favorable outcomes (such as reduction of gadolinium-enhancing lesions, reduction of relapse rate, reduction of brain atrophy and disability progression, as it was recently shown in a study involving secondary progressive MS patients [112]), as opposed to no significant reduction in relapse rate or disease progression or even increased magnetic resonance imaging (MRI) and clinical disease activity and antagonism of interferon-beta function in some cohorts [111, 113]. Further investigation and reproducible results should be awaited before the regular use of statins in MS patients in daily clinical practice, as highlighted by Sena et al. [114–116]. Although there are well-known adverse effects associated to the use of this family of drugs, it appears that they will achieve an acceptable safety and tolerability profile [111].
As in AD, obesity and adipocytokines have also been related to MS. In the best-known animal model of the disease (experimental autoimmune encephalitis [EAE]), caloric restriction was associated to higher levels of serum adiponectin (anti-inflammatory adipokine) and reduced susceptibility and severity of EAE. In addition, leptin, a pro-inflammatory adipokine, appears to be a requisite for EAE induction [117]. Accordingly, leptin-deficient mice have been shown to present myelin with an abnormal biochemical composition and also a decrease in CNS myelination, thus contributing to make these animals resistant to the disease [6, 117].
In recent studies, women with MS have been shown to have higher BMI than recommended by the World Health Organization (50 % being overweighed, of which 50 % were obese), but this is not different from higher BMI seen in normal controls [103]. Furthermore, an interesting association between obesity (especially in childhood and adolescence) and an increased risk for developing MS has been observed, although this association was only significant for females, showing a dose-dependent relationship [118]. Other theories associating obesity and MS include the relationship between the coexistence of HLA-DRB1*15 and obesity (increased risk for developing MS, OR = 3) or the absence of HLA-A*02 and presence of obesity (increased risk for developing MS, OR = 15) [119]. The association between obesity, lipid profile, and levels of serum vitamin D has also been reported, stressing that higher levels of vitamin D are associated with a reduced risk of MS in early adulthood and that total body fat is inversely related to the levels of circulating 15-hidroxy-vitamin D [120]. This vitamin is known to produce immunomodulatory and antioxidant effects, regulates the expression of cyclooxygenases, interferes with transcription factors such as NF-κB, regulates activation of signaling cascades (such as MAP kinases, among others), and targets a myriad of cell types such as monocytes/macrophages, dendritic cells, and B and T lymphocytes. All of the above have given rise to a large amount of studies evaluating the effect of vitamin D supplementation in MS patients with promising results [120].
Glucose metabolism could also be impaired in patients with MS and higher risks of T2D are reported in literature [103]. The association between T2D and higher disability has also been shown in the NARCOMS cohort [107]. In a recent study of 110 patients with MS and 175 healthy controls, a higher proportion of insulin resistance among MS patients (40 % vs. 21 %, OR = 2.48) was found, and those patients with insulin resistance showed higher EDSS scores, IL-6 and IL-17 levels, as well as markers of oxidative stress than MS patients with normal glucose metabolism. In a multivariate analysis, EDSS score was also associated with central adiposity and BMI, and hyperinsulinemia was related with cognitive impairment in MS [121].
< div class='tao-gold-member'>
Only gold members can continue reading. Log In or Register a > to continue
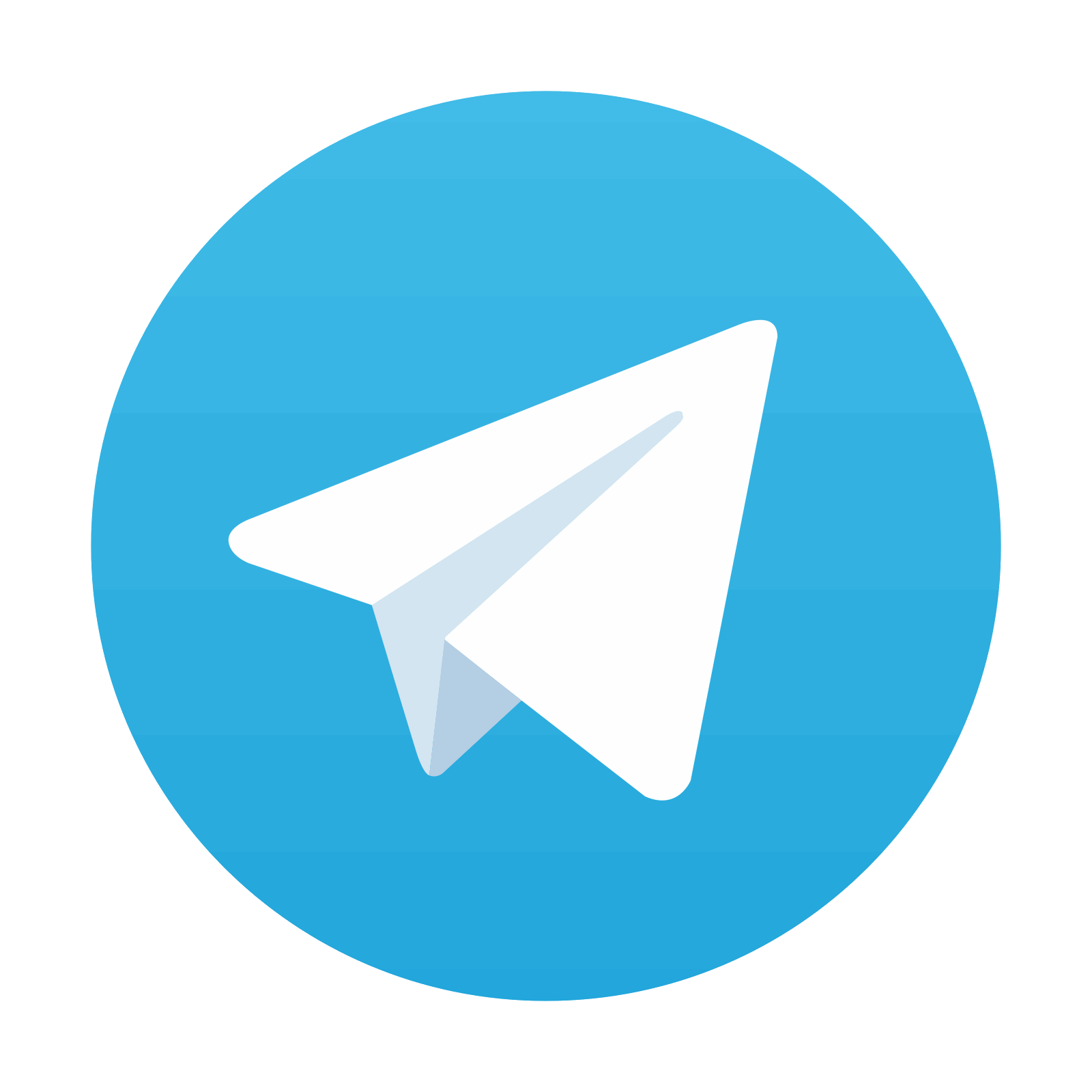
Stay updated, free articles. Join our Telegram channel

Full access? Get Clinical Tree
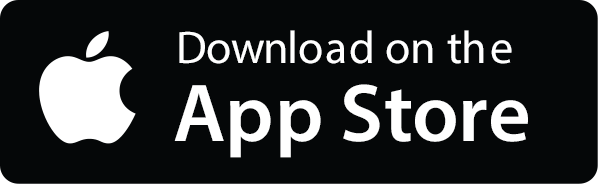
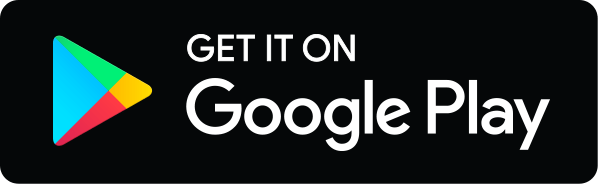