Cardiogenic Shock in the Setting of Acute Myocardial Infarction
Zaza Iakobishvili
David Hasdai
Cardiogenic shock is defined as a state of tissue hypoxia caused by reduced cardiac output in the presence of adequate intravascular volume (1). Cardiogenic shock may be the consequence of cardiovascular disorders other than acute myocardial infarction (AMI) and occurs even in the presence of well-preserved left ventricular function (2). Due to the broad clinical spectrum of cardiogenic shock, no single laboratory or clinical marker can define fully this condition. No universally accepted cut-off value of systemic cardiac output constitutes the state of shock. In several series, cardiac index measurements of 2.2 L/min per m2 or less were considered as confirmatory of the diagnosis (3, 4, 5, 6, 7, 8, 9), whereas others have considered measurements of 1.8 L/min per m2 or less as indicative of cardiogenic shock (10). In addition, measuring methods may vary. The most accepted method of cardiac output determination uses pulmonary artery catheters. Because this procedure carries minimal, but measurable risks, it is not universally used in patients with cardiogenic shock (11).
Tissue hypoxia is difficult to assess by bedside assays, thus surrogate clinical and laboratory indices are sought. These indices cannot determine the gravity of tissue hypoxia and cannot be used as single determinants of shock state (1).
Systemic hypotension is regarded as essential to the diagnosis of cardiogenic shock. The severity of hypotension differs among the investigators—common cut-off values for systolic blood pressure are <90 mm Hg (3, 4, 5, 6, 7, 8, 9) or <80 mm Hg (10,12,13). Some clinicians consider the diagnosis of cardiogenic shock in patients with blood pressure greater than 90 mm Hg, if they require medications and support devices to maintain normal hemodynamic parameters. A patient initially may have clinical signs of cardiogenic shock in the presence of systolic blood pressure measurements greater than 90 mm Hg (9). In these patients (usually with preexisting hypertension), a drop of 30 mm Hg or more in the systolic blood pressure often is included in definition of shock.
The method of blood pressure measurement also is important. Brachial cuff pressure measurements often are inaccurate in states of shock. Arterial blood pressure is more accurately monitored using intra-arterial cannulae.
This brief discussion underscores the difficulty of diagnosing cardiogenic shock by numerical and laboratory values alone; thus, cardiogenic shock primarily is diagnosed based on clinical findings. The diagnosis can be supported by measured hemodynamic values. For the diagnosis of cardiogenic shock, any reduction in cardiac output accompanied by signs of systemic hypoperfusion must be present. Systemic signs of hypoperfusion may include an altered mental status, cold clammy skin, and oliguria.
ETIOLOGY
Typically, cardiogenic shock occurs in the setting of massive damage of myocardium due to large AMI, but other possibilities must be always kept in mind. In the Should We Emergently Revascularize Occluded Coronaries for Cardiogenic Shock (SHOCK) registry (14), predominant
left ventricular failure was detected in 78.5% of patients. However, acute severe mitral regurgitation occurred in 6.9%, ventricular septal defect was seen in 3.9%, tamponade/rupture in 1.4%, and right ventricular shock in 2.8%. Determination of the specific etiology of shock has obvious importance for selecting an optimal treatment strategy, especially when AMI occurs on top of previous valvular disease or congenital cardiac defect (for example, atrial septal defect).
left ventricular failure was detected in 78.5% of patients. However, acute severe mitral regurgitation occurred in 6.9%, ventricular septal defect was seen in 3.9%, tamponade/rupture in 1.4%, and right ventricular shock in 2.8%. Determination of the specific etiology of shock has obvious importance for selecting an optimal treatment strategy, especially when AMI occurs on top of previous valvular disease or congenital cardiac defect (for example, atrial septal defect).
INCIDENCE
Cardiogenic shock can occur as a complication of unstable angina, non-ST-elevation AMI, and ST-elevation AMI (15). Due to differences in the definition of cardiogenic shock and the criteria for inclusion of patients, the reported incidence of cardiogenic shock complicating acute coronary syndromes (ACS) vary between different series. The data regarding the incidence of cardiogenic shock are derived from large population-based analyses as well as from subset analyses of randomized clinical trials that studied effects of different treatment modalities in ACS.
Non-ST-Elevation Acute Coronary Syndrome and Cardiogenic Shock
In the setting of non-ST-elevation ACS, relatively limited data exist on the incidence of cardiogenic shock. This has important implications, because the incidence of non-ST-elevation ACS appears to be increasing significantly (16). In the Global Use of Strategies to Open Occluded Coronary Arteries (GUSTO IIb) trial (3), one of the largest clinical trials undertaken, cardiogenic shock was a predefined subset to be analyzed. Among the 8,001 patients with non-ST-elevation ACS, cardiogenic shock occurred in 2.6% of cases. This was about half of the shock incidence in the ST-segment-elevation ACS subgroup in the same trial (odds ratio [OR] 0.50, 95% confidence interval [CI] 0.413, 0.612, p <0.001). The subset of patients with cardiogenic shock was also analyzed in the Platelet Glycoprotein IIb/IIIa in Unstable Angina: Receptor Suppression Using Integrilin Therapy (PURSUIT) trial of patients with non-ST-elevation ACS (7). Of the 9,449 patients enrolled, 2.5% (237) developed shock after enrollment. In another large survey of ACS, the Euro Heart Survey of ACS, the incidence of cardiogenic shock among patients admitted with non-ST-elevation ACS was 2.4% (of whom 1.7% developed it during hospitalization) (17).
ST-Segment-Elevation Acute Coronary Syndrome and Cardiogenic Shock
The most common cause and studied etiology of cardiogenic shock is acute ST-segment-elevation ACS. In the prethrombolysis era, Leor et al. reported that, among patients with AMI without signs of heart failure upon presentation, cardiogenic shock developed in hospital in 2.6% of 3,465 patients (18).
In three large, international thrombolytic therapy trials for acute ST-elevation AMI, the incidence of cardiogenic shock ranged from 4.2% to 7.2% (6,9,19). However, the reported incidence of cardiogenic shock among ST-segment-elevation AMI patients receiving thrombolytic therapy seems to be biased, because the patients with cardiogenic shock are often not enrolled in multicenter randomized trials.
Goldberg et al. evaluated the trends in the incidence of cardiogenic shock complicating ST-segment-elevation AMI in a single community from 1975 to 1997 (13). The overall annual incidence during this period was 7.1%, and ranged from 4.5% to 8.6%. In an observational study, the second National Registry of Myocardial Infarction (NRMI-2), which analyzed data of 426,253 patients with AMI hospitalized in the United States between 1994 and 1997, the mean incidence rate of cardiogenic shock was 6.2% (20). In the Euro Heart Survey of ACS, the incidence of shock was 8.5% in the ST-segment-elevation AMI group (21).
Overall, cardiogenic shock occurs in all types of ACS and is more than twice as frequent in ST-segment-elevation ACS as in non-ST-elevation ACS. No significant change has occurred in the incidence of cardiogenic shock complicating AMI over the past two decades (13).
Right ventricular infarction is the distinct entity in the cardiogenic shock spectrum. Most of these patients were not included in randomized trials. No exact data exists on the frequency of cardiogenic shock in the presence of right ventricular infarction. In the SHOCK Registry, the incidence of right ventricular infarction causing cardiogenic shock was 2.8% (14).
TIME TO DEVELOPMENT OF CARDIOGENIC SHOCK IN ACUTE CORONARY SYNDROME
An apparent discrepancy exists, probably due to selection bias, among data from randomized trials and population-based analyses in regard to the time of shock development. In randomized trials, approximately 90% of patients with shock developed it after enrollment, and only approximately 10% had shock on arrival. However, in a population-based study of unselected patients enrolled with AMI, 56% of shock patients had shock upon arrival (22).
In the prethrombolysis era, Leor et al. (18) reported that shock developed at a mean of 4 ± 4 days after admission (median 2 days, range 3 hours to 16 days) in patients who were admitted with Killip class I status. Hands et al. (23) reported that shock occurred at a mean of 3.4 ± 0.8 days (with half the patients developing shock within the first 24 hours) in patients who did not have shock upon admission.
In large thrombolytic trials, the median time to the occurrence of shock among patients with persistent ST-segment elevation who developed in-hospital shock was 10 or 11 hours (6,8,15), with most experiencing shock within the first 48 hours after enrollment. Shock occurred later after symptom onset in non-ST-elevation patients than in those with ST-segment elevation. In GUSTO IIb, patients with non-ST-segment elevation developed shock a median of 76 hours after enrollment, in contrast to ST-segment-elevation patients, in whom the median time was 9.6 hours. In the multicenter SHOCK registry (24), the time from AMI onset to shock onset was also different: 8.9 hours for non-ST-segment elevation versus 5.9 hours for ST-segment-elevation infarction. In the PURSUIT database of non-ST-elevation ACS, shock most commonly developed more than 48 hours after enrollment (median 94.0 hours) (7).
In summary, in population-based studies, more than half of patients with cardiogenic shock have shock upon arrival. Thrombolysis shortens time to shock development. Patients with ST-segment-elevation ACS develop cardiogenic shock earlier than the patients with non-ST-elevation ACS.
PREDICTORS OF SHOCK
With the use of readily available clinical data, several algorithms can help in predicting the probability of shock development in ACS patients.
The algorithm for patients with persistent ST-segment-elevation AMI was devised in the GUSTO-I trial. It was subsequently validated in the GUSTO-III trial, with a high concordance index of 0.79 (3). Among patients with ST-segment elevation, older age was the variable most strongly associated with shock. Other predictors derived from the physical examination, such as systolic blood pressure, heart rate, and Killip class, were also strong predictors of subsequent development of shock. These parameters provided more than 85% of the information needed to predict shock in this model.
Algorithms also were developed and tested for non-ST-elevation ACS. In the PURSUIT study (7), the most important factors associated with development of shock were older age and ST depression of >0.5 mm. Using these and other simple factors (systolic blood pressure, pulse, height, presence of pulmonary rales), a scoring algorithm was developed (7). The concordance index of the original logistic model was 0.71; of the validated model, it was 0.67. Hasdai et al. then tested the validity of this model from the PURSUIT database in the GUSTO-IIb patients and found a concordance index of 0.68, again indicating reliability of the model in predicting shock.
Despite the differences in the pathophysiology and clinical presentation of cardiogenic shock between patients with persistent ST-elevation AMI and patients with other ACS, the predictors for the development of shock are quite similar. Patients with AMI are more likely to develop shock than patients with unstable angina. Patients with significant ST-segment depression in the initial electrocardiogram are more prone to the development of shock. Among patients with ST-segment-elevation AMI, the extent of ST-segment elevation, the presence of reciprocal ST-segment depression, and the contour of the terminal portion of the QRS complex all can be important in predicting the development of shock.
All these algorithms were developed to complement, but not supplant, sound clinical judgment.
OUTCOME
The outcome of cardiogenic shock complicating ACS seems to have improved during a 23-year period, with the greatest improvement in mortality occurring during the 1990s (13). From 1975 through 1990, the in-hospital mortality from cardiogenic shock decreased from 70% to 61% between 1993 and 1995 and further to 59% in 1997. In the Global Registry of Acute Coronary Events (GRACE) registry that enrolled patients between 1999 and 2001, the in-hospital mortality rate for cardiogenic shock patients was 59% (25). In the Euro Heart Survey of ACS, the in-hospital mortality rate for cardiogenic shock was 52% (21).
In thrombolytic trials, the mortality rate from cardiogenic shock remained high even in those patients treated by thrombolysis: In GISSI-1, the 21-day mortality among those with shock at presentation was 69.9% in those randomized to streptokinase (n = 146) and 70.1% in those randomized to placebo (n = 134) (26). In GUSTO-I, the in-hospital mortality rate for cardiogenic shock was 55% (6). In GUSTO-III, the mortality rate remained high—63% in the reteplase group and 65% in the alteplase group (19).
Once cardiogenic shock has occurred, the rate of mortality is not different irrespective of the status of ST-segment deviation. In the GUSTO-IIb trial, 72.5% of non-ST-segment-elevation patients with shock died versus 63.0% of ST-segment-elevation patients. In the SHOCK registry, the mortality was also high and similar: 62.5% for non-ST-segment elevation and 60.4% for ST-segment elevation (p = 0.65). In the PURSUIT trial, 65.8% of shock patients died within 30 days. In a subset analysis among shock patients, those who presented with AMI had a higher incidence of mortality at 30 days (77.2% compared with 52.7%, p = 0.001).
Some geographical differences may exist in cardiogenic shock outcome, as evidenced by the analysis of GUSTO-I trial (4). Adjusted 30-day mortality was significantly lower among patients treated in the United States than among those treated elsewhere (50% versus 66%, p < 0.001). The same trend was observed in GUSTO-III population: 53% 30-day mortality in USA-enrolled patients versus 66% in other countries. However, when the adjustment was made
for greater use of intra-aortic balloon pump counterpulsation and revascularization procedures, no significant difference was observed in mortality between the USA and non-USA cohort (19).
for greater use of intra-aortic balloon pump counterpulsation and revascularization procedures, no significant difference was observed in mortality between the USA and non-USA cohort (19).
In the SHOCK trial registry examining cardiogenic shock caused by right ventricular infarction, mortality was unexpectedly high in patients with predominant RV shock and similar to patients with predominantly left ventricular failure shock—despite the younger age, lower rate of anterior MI, and higher prevalence of single-vessel coronary disease of right ventricular compared with left ventricular shock patients, and their similar benefit from revascularization (27).
MANAGEMENT OF CARDIOGENIC SHOCK PATIENTS
Cardiogenic shock patients need thorough hemodynamic monitoring due to the extreme complexity of this condition. In addition to invasive systemic blood pressure monitoring, many authorities advocate the use of right heart catheterization and/or echocardiography for guiding therapeutic measures.
Right Heart Catheterization
Based on apparent difficulties in noninvasive assessment of left ventricular filling pressures, clinicians often consider the direct measurement of pulmonary artery pressure using a pulmonary artery (Swan-Ganz) catheter. In the early 1990s, a randomized trial of right heart catheterization use in critically ill patients, which included some cardiogenic shock patients, was terminated early because the enrolling physicians felt that randomization was unethical (28).
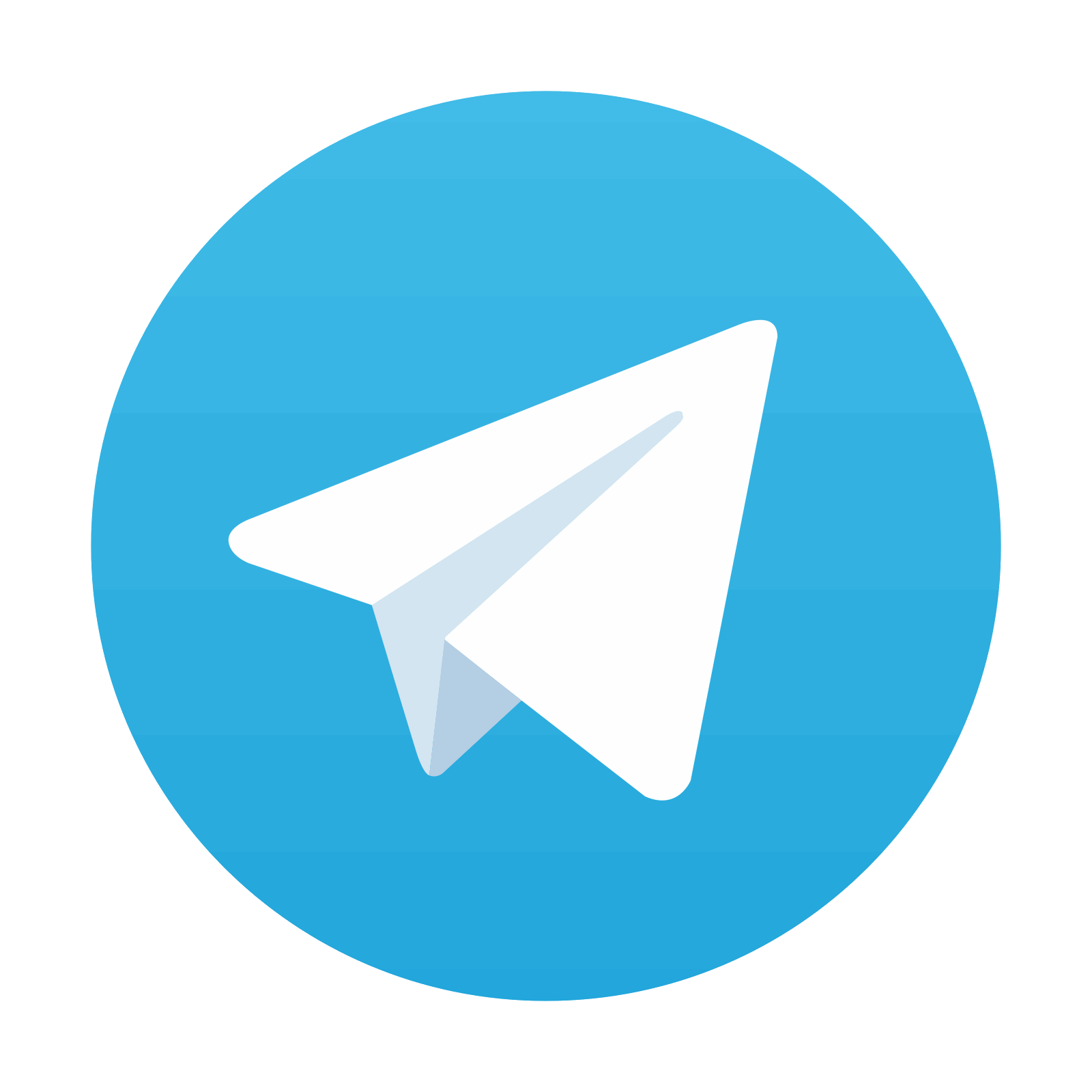
Stay updated, free articles. Join our Telegram channel

Full access? Get Clinical Tree
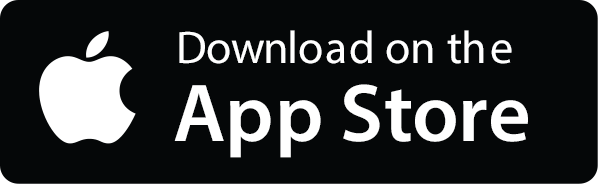
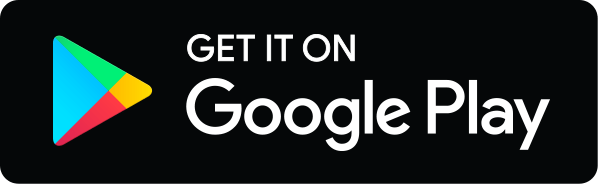