Outline
Epidemiology of Heart Failure in Patients with Cancer, 665
Specific Cancer Therapies, Their Mechanisms of Cardiotoxicity, and Implications for Clinical Practice, 666
Anthracyclines, 666
Radiation Therapy, 666
Cyclophosphamide, 667
Targeted Therapies, 667
ErbB2 Targeted Therapies: Trastuzumab, Lapatinib, and Pertuzumab, 667
Angiogenesis Inhibitors, 668
Bcr-Abl Inhibitors, 669
Proteasome Inhibitors, 669
Immune Checkpoint Inhibitors, 670
Emerging Cancer Therapies, 670
Risk Assessment, Screening, and Monitoring, 670
Prevention of Heart Failure During Cancer Treatment, 671
Modification of Chemotherapeutic Regimens, 671
Dexrazoxane, 671
Beta-Blockers, 671
Renin-Angiotensin-Aldosterone Axis Inhibition, 672
Statins, 672
Managing Heart Failure During Cancer Treatment, 672
Advanced Therapies in the Cancer Patient with Heart Failure, 672
Heart failure (HF) as a consequence of cancer treatment is a well-known clinical challenge with a history as old as the earliest days of cytotoxic chemotherapies and radiation-based treatment. During the past several decades of advancement in cancer treatment with overall improvements in cancer survival, cardiovascular (CV) toxicities, specifically HF, have become increasingly recognized as a consequence of treatment with a diverse set of agents. Fortunately, a substantial amount of cardiotoxic effects may be preventable with careful control of known CV risk factors and the use of selected cardio-protective therapy. When left ventricular (LV) dysfunction does occur, prompt identification and treatment can lead to recovery, while delayed treatment is associated with much more limited benefit.
For patients who are still actively receiving chemotherapy or targeted therapy, decisions to hold or stop chemotherapy due to concern for cardiotoxicity are complex, with limited evidence for guidance but with obvious life and death implications. A close collaboration between the cardiology and oncology teams is therefore mandatory to optimize overall care. Differentiating between asymptomatic or mild LV dysfunction and more severe cardiomyopathy, as well as whether the HF is likely related to the chemotherapy, have major repercussions with regard to future treatment. For more in-depth coverage of this topic, recent consensus statements and recommendations by experts in this field are important resources.
Epidemiology of Heart Failure in Patients with Cancer
The population of patients who may have CV diseases, including HF, as well as cancer, increases each year. This is driven by several factors, most notably a direct result of the success of cancer therapies leading to greater survivorship, the age-associated risk of heart disease and malignancy in an aging population, the overlap in risk factors between heart disease and malignancy, and ultimately effects of targeted cancer therapy. Cancer survivors are at substantial increased risk compared to persons without malignancy for developing ischemic heart disease and HF throughout their lives. Health behaviors, such as tobacco use and alcohol abuse, as well as obesity and associated metabolic disorders, increase the risk of developing both HF and certain cancers. In addition, CV disease and HF are known consequence of certain cancer therapies. The improvements in outcome for pediatric cancer patients have been tremendous and have resulted in a growing population of adults at risk for HF. A striking example of unintended consequence of cancer treatment is the apparent rise in incidence of heart transplant patients with a history of anthracycline cardiomyopathy. Additionally, although radiation therapy has improved over the decades, chest radiation remains an important cause of significant heart disease, including complex vascular and valvular disease, that may result in HF. Newer emerging cancer therapies, which offer a great deal of hope for patients who have previously incurable malignancies, such as metastatic breast cancer and multiple myeloma, unfortunately do have direct and indirect deleterious effects on CV tissues in susceptible patients. The intertwining impact of cancer therapies in a myriad of CV tissues needs to be understood by both oncologists and cardiologists. In one study of Medicare patients over the age of 66 diagnosed with breast cancer, CV death was more prevalent than death due to the cancer itself.
A growing number of agents are known to cause cardiac dysfunction and HF ( Table 46.1 ). Thus, a patient being treated with potential cardiotoxic therapy should be considered a Stage A HF patient per American College of Cardiology (ACC)/American Heart Association (AHA) guidelines. The risk of HF varies by chemotherapeutic regimen and individual risk factors, and there may be considerable time delay between exposure and clinical presentation. For some older chemotherapeutics, a great deal is known about CV safety and toxicity, while for newer therapies the risks may be largely unidentified. Moreover, many life-saving cancer therapies gain approval for treatment of cancer with relatively limited data regarding toxicity. Additionally, there may be an unpredictable lag time that occurs after the administration of chemotherapy before any development of the signs and symptoms of HF. As a result, if HF or other CV disease is detected in a patient with cancer during or after treatment with a novel therapy, some consideration should be given that the cancer treatment may be promoting CV disease.
Class of Therapy | Generic Names | Timing | Risk Factors for HF |
---|---|---|---|
Anthracyclines | doxorubicin daunorubicin epirubicin idarubicin mitoxantrone | Concurrent and delayed | Cumulative dose Concurrent radiation therapy, cyclophosphamide, or ErbB2 targeted therapies Age (young and old) Hypertension Known heart disease |
Alkylating agent | cyclophosphamide | Early | Dose, age, concurrent anthracyclines |
HER2(ErbB2)-targeted therapies | trastuzumab pertuzumab lapatinib | Concurrent | Hypertension, age, low pre-treatment LVEF |
VEGF/angiogenesis targeted therapies | sunitinib sorafenib pazopanib bevacizumab | Concurrent | Hypertension |
Bcr-Abl inhibitors | imatinib ponatinib | Concurrent | |
Proteasome inhibitors | bortezomib carfilzomib | Concurrent | Prior anthracyclines |
Mediastinal/chest radiation | Delayed | Cumulative dose Concurrent anthracyclines Age (young) Hypertension | |
Check point inhibitors | ipilimumab nivolumab pembrolizumab | Concurrent | Combination therapy |
There are also situations where HF may be a direct consequence of malignancy, rather than therapy. For instance, infiltrative cardiomyopathy secondary to primary amyloid light-chain (AL) amyloidosis occurs in the setting of multiple myeloma and plasma cell dyscrasia. The unique challenges this condition presents to the HF clinician are covered in detail elsewhere ( see Chapter 22 ).
Specific Cancer Therapies, Their Mechanisms of Cardiotoxicity, and Implications for Clinical Practice
Anthracyclines
Anthracyclines are a mainstay of cancer treatment and a well-recognized cause of cardiomyopathy and HF. Anthracyclines’ main antitumor effect is through the inhibition of topoisomerase II (topII), an enzyme involved in uncoiling DNA. By inhibiting the enzyme, anthracyclines induce DNA strand breaks and apoptosis. In addition to the topIIα (topIIα) form of the enzyme that is predominant in cancer cells, there is also a topIIβ (topIIβ) form expressed in cardiac myocytes. Anthracyclines inhibit both, and the inhibition of the latter is plausibly culpable for a significant amount of the compound’s cardiotoxicity. Mice lacking topIIβ are resistant to the cardiotoxicity of anthracyclines. Additional anthracycline toxic effects also have been linked to the generation of free radicals with the subsequent generation of reactive oxidant species that produce oxidative damage to lipids, proteins, and nucleic acids ( Fig. 46.1 ).

It is well established that an individual’s risk of HF secondary to anthracyclines is directly related to the cumulative drug exposure, which is consistent with the concept that every anthracycline dose potentially causes some degree of irreversible cardiac damage. A combined analysis of three doxorubicin Phase III studies found a 7% incidence of cardiac events (LV dysfunction or heart failure) even at a lower dose of 150 mg/m 2 . A dose threshold ≥250 mg/m 2 of doxorubicin (≥600 mg/m 2 of epirubicin) has been identified, mainly from childhood cancer survivor studies, as being a threshold where the rate of HF increases substantially. Other known risk factors for anthracycline HF include age (both young and elderly), hypertension, preexisting cardiac conditions, concurrent mediastinal radiation, and/or other therapies with cardiac effects including cyclophosphamide, paclitaxel, and trastuzumab (see later).
Despite the likely immediate loss of myocardium during anthracycline treatment, patients with cancer frequently remain asymptomatic or only minimally symptomatic for months to years. Contractile reserve and compensatory mechanisms on the cellular and subcellular level presumably maintain cardiac function. One of the survival factors implicated in the modulation of anthracycline cardiotoxicity is the neuregulin/ErbB signaling system, explaining the increased toxicity when anthracyclines are used concomitantly with ErbB2-targeted therapeutics.
Genetic analysis of cohorts by genome-wide associations studies have identified a number of genetic loci that speak to the importance of cellular uptake mechanisms and carbonyl redox cycling in the cardiotoxicity of anthracyclines. Loci associated with genes encoding protein components of cellular transport systems including the ATP-cassette transporters in the multidrug resistance family are associated with the risk of cardiotoxicity. Animal work supports the notion that these transporters regulate the uptake of anthracyclines. This purported mechanism has important implications for patients undergoing treatment with anthracyclines that are typically underappreciated by most practitioners. Commonly prescribed medications, including antihypertensives diltiazem and verapamil, are competitively transported by these same proteins, and concomitant treatment with these or other similarly transported pharmaceuticals, such as cyclosporine or digoxin, will raise the cytoplasmic anthracycline concentration. Other antihypertensives such as beta-blockers (BBs), and angiotensin converting enzyme inhibitors (ACEIs) are not handled by this transporter and therefore will not alter intracellular anthracycline concentrations. Thus, all patients should have a careful review of their medication list with a focus on identifying substrates for the p-glycoprotein/MDR/ATP-cassette transporters, and consideration for a change in medication class if appropriate.
Radiation Therapy
Radiation therapy remains an important component of therapy for specific cancers. A history of mediastinal radiation may result in HF by a variety of mechanisms, and should be factored into the evaluation of any patient presenting with CV-based symptoms. The effects of mediastinal radiation on the heart are related to radiation dose, and can manifest anywhere between a few years after exposure to over two decades later. A dose ≥30 Gy has been identified as a marker for significantly increased risk, being associated with a 2.8- to 4.7-fold risk of HF as a first event compared to no mediastinal radiation treatment (RT) after adjustment for anthracycline dose and other risk factors.
Radiation can affect every part of the heart that is in the path of treatment, causing diffuse atherosclerosis, valvular disease, conduction disorders, pericardial disease, and myocardial disease with both diastolic and systolic dysfunction. Chest radiation is also associated with pulmonary hypertension, and a 3% incidence of pacemaker or defibrillator malfunction in patients with preexisting devices. The only known strategies to prevent cardiac disease during RT are to limit CV exposure, and to control CV risk factors optimally with the hopes of reducing the risk of subsequent CV injury. It is recommended that cardiologists are involved in patients with devices undergoing radiotherapy to help with appropriate planning and monitoring.
Cyclophosphamide
Acute cardiac injury in the form of myocarditis and pericarditis is a known complication of cyclophosphamide, particularly when used at high doses during induction therapy for some bone marrow transplant regimens. It is estimated that HF may occur in up to 7% to 28% of patients receiving high dose cyclophosphamide, but the exact incidence has not been established. Pathologic examination shows disruption of endothelial cells, hemorrhagic myocarditis, and disrupted myocyte ultrastructure. Fulminant HF refractory to treatment has been reported in certain cases in which high dose cyclophosphamide was used. Risk factors identified for the development of cardiomyopathy include age, dose, and concurrent treatment with anthracyclines. There are no current practice guidelines for monitoring cardiac function after cyclophosphamide induction therapy, nor any accepted practices for prevention. Careful monitoring by physical exam for signs of fluid retention, with maintenance of high suspicion for cardiac contribution to nonspecific symptoms of fatigue and malaise in the early weeks/months after induction therapy with cyclophosphamide is clinically prudent.
Targeted Therapies
Recent advances in cancer therapy have occurred through the discovery of specific signaling cascades contributing to cancer progression and the development of novel agents that target these processes. Several of these therapies have been associated with the development of HF and cardiomyopathy, and are highlighted below.
ErbB2 Targeted Therapies: Trastuzumab, Lapatinib, and Pertuzumab
Trastuzumab (Herceptin) and pertuzumab (Perjeta), are recombinant humanized monoclonal antibodies against the human epidermal growth factor receptor (EGFR) 2 (HER2, a.k.a. ErbB2) protein, an oncogene that when overexpressed in tumor cells promotes cell proliferation through constitutive activation of growth signaling pathways. Lapatinib (Tykerb/Tyverb) is a small molecule dual tyrosine kinase inhibitor (TKI) of the EGFR and the ErbB2 receptor, that blocks receptor activation of intracellular signaling. These therapies are used for the treatment of ErbB2-overexpressing tumors, including breast and gastric cancers. Cardiac side effects of ErbB2-targeted therapeutics were first detected in the pivotal trastuzumab breast cancer trials, where trastuzumab combined with anthracyclines showed greater cardiotoxicity compared to anthracycline alone. The contemporary use of trastuzumab has expanded to include adjuvant therapy before and after surgery, potentially for longer than 1 year and for extended periods (more than 4 years). Risk factors for trastuzumab-associated cardiac dysfunction include older age, hypertension, diabetes mellitus, atrial fibrillation or flutter, adjuvant chemotherapy, renal failure, coronary artery disease, and weekly (more often) trastuzumab transfusion. In contrast to delayed anthracycline cardiomyopathy, ErbB2-targeted therapy-related cardiac dysfunction generally occurs during treatment. There is a reasonable likelihood of reversibility of LV dysfunction, especially when treated with typical HF therapy. The cardiotoxic potential of lapatinib appears to be less than trastuzumab, perhaps due to the shorter half-life of this small molecule Pertuzumab, on the other hand, has a similar rate of observed cardiotoxicity to that of trastuzumab alone, but does not appear to have additive toxicity in large clinical trials. While this may be due to differences in the biological properties of these ErbB2-targeted therapies, there has also been much greater scrutiny of cardiac function in patients being considered for treatment with ErbB2-targeted therapies since the initial trials.
The cardiac side effects of anti-ErbB2 therapeutics might be explained by studies examining the biology of the neuregulin, an EGF-family ligand and ErbB receptor in the CV system ( Fig. 46.2 ). Neuregulin, ErbB2, and ErbB4 receptor tyrosine kinases play an important role in cell–cell crosstalk and mediate cellular responses to stress in the heart. ErbB2 is expressed in the adult cardiomyocytes and disruption of its expression through genetic engineering in mice leads to progressive dilated cardiomyopathy. Myocardial stress induced by ischemia activates neuregulin/ErbB signaling in the heart, and suppressing signaling prevents recovery from ischemic injury.

Angiogenesis Inhibitors
Cancer treatments that target the signaling pathways for vascular endothelial growth factor (VEGF), and ultimately are intended to be angio-genic, are an important class of compounds that have been associated with development of HF. VEGF regulates angiogenesis, endothelial cell survival, vasodilatation, and cardiac contractile function. The VEGF-targeted angiogenesis inhibitors, like ErbB2 targeted therapy, are either antibody-based, which bind and neutralize VEGF (e.g., bevacizumab), or small molecular inhibitors of VEGF tyrosine kinase receptors (TKIs; e.g., sunitinib, sorafenib). These are now used in the treatment of a variety of cancers.
A general CV class effect of these compounds is the augmentation of blood pressure. Hypertension related to VEGF signaling pathway inhibition appears to occur through several mechanisms, including increased vascular stiffness, which may be mediated by increased activity of the renin–angiotensin system. A preeclampsia-like syndrome with proteinuria and thrombotic microangiopathy has been reported in a subset of patients. VEGF activates vascular eNOS via the PI-3kinase/PKB/Akt pathway, and this is one mechanism for VEGF-mediated vasorelaxation. Mice lacking VEGF demonstrate glomerular pathology, with loss of podocytes and disruption of the basement membrane. VEGF-targeted therapies are also associated with a 3-fold increase in risk for arterial thromboembolic events (stroke, transient ischemic attacks, myocardial infarction, angina, and other arterial events), which appears to be an additional consequence of disrupting endothelial homeostasis.
LV dysfunction and HF occurs in patients treated with bevacizumab (monoclonal antibody to the VEGF receptor), although HF appears to be more common in those treated with VEGF-targeted TKIs. VEGF signaling mediates the adaptation of the heart to pressure overload. Suppressing VEGF signaling in the setting of pressure overload leads to more rapid deleterious cardiac remodeling and HF in mice.
Retrospective analyses of sunitinib-associated cardiac dysfunction report that up to 10% of patients receiving sunitinib experience some degree of HF, although larger meta-analysis have reported an incidence of 4%. Sunitinib induces mitochondrial injury and cardiomyocyte apoptosis in both mice and cultured rat cardiomyocytes. Sunitinib inhibits several tyrosine kinases including AMP-activated kinase (AMPK) and platelet-derived growth factor receptors (PDGFR), which may explain the higher rate of clinical cardiac toxicity ( Fig. 46.3 ). Mice lacking PDGF receptor expression respond to pressure overload induced by surgical aortic constriction with accelerated cardiac remodeling associated with impaired vascular growth and function. Mice lacking AMPKa2 similarly are normal in the unstressed condition, but experience accelerated remodeling and LV dysfunction after pressure overload. It is interesting that in each of these animals the phenotype of cardiac dysfunction is not manifested unless the animals are subjected to pressure overload. This would suggest that the hypertensive effect of VEGF-targeted TKIs may be requisite for the induction of contractile dysfunction.

It is yet unclear whether cardiac dysfunction induced by angiogenesis inhibitors is reversible. In early reports, patients with HF and LV dysfunction generally responded to temporary suspension of sunitinib and medical management. However, the preclinical data in animals and cells supports a proapoptotic effect of sunitinib that would be associated with some degree of irreversible cardiac damage. Persistent cardiac dysfunction has been noted in some persons treated with sunitinib. Predicting and preventing HF in these patients remains an important challenge for the future.
Bcr-Abl Inhibitors
Inhibitors of “Philadelphia Chromosome,” Bcr-Abl, have become the first-line therapy for the ∼90% of patients with chronic myelogenous leukemia, as well as other tumors with this oncogene. Imatinib (Gleevec) was the first Bcr-Abl inhibitor developed. Imatinib-associated LV systolic dysfunction has been reported, and may occur due to the inhibition of Abl in cardiac myocytes where it regulates endoplasmic reticulum stress responses. Second- and third-generation Bcr-Abl inhibitors have been developed, which have distinct properties—most importantly the ability to overcome imatinib resistance via a number of strategies. Certain newer Bcr-Abl inhibitors have been linked to higher rates of peripheral arterial occlusive disease (nilotinib). Most recently, a unique Bcr-Abl inhibitor that is able to block a specific mutant form of Bcr-Abl, ponatinib, has been linked to high rates of thromboemboli, arterial occlusion, and HF.
Proteasome Inhibitors
The ubiquitin–proteasome system (UP-S) is a cellular protein degradation system that is vital to normal cellular function, and in some tumors critical for proliferation. Preclinical studies have shown that proteasome disruption inhibits proliferation, induces apoptosis, reverses chemoresistance, and enhances chemotherapy and radiation efficacy in some tumors. The proteasome pathway is also critical for the maintenance of cardiac structure and function, and altered proteasome function is associated with cardiac pathophysiology.
Bortezomib (Velcade) was the first proteasome inhibitor to be developed successfully as a cancer therapy. Bortezomib blocks proliferation and induces apoptosis of plasma cells, and is therefore used for the initial treatment of patients with multiple myeloma. It also enhances sensitivity to conventional chemotherapy by inhibiting the proteasomal degradation of signaling molecules that regulate cell survival. The side effects reported include asthenia, peripheral neuropathy, gastrointestinal symptoms, and anorexia. There are a few reports of cardiac failure occurring in patients during treatment with bortezomib, suggesting that under some circumstances inactivation of the cardiac proteasome can result in adverse effects on the heart.
Because of the success of generally well-tolerated bortezomib, newer proteasome inhibitors have been developed. Carfilzomib, an irreversible proteasome inhibitor, is approved as second-line therapy for relapsed multiple myeloma. Although this therapy appears to be more active in resistant myeloma cases than previous treatments, there may be a greater likelihood of cardiac events including HF, sudden cardiac death, and acute coronary syndrome. It does seem apparent that there is a vascular component to the observed cardiotoxicity associated with carfilzomib, especially in combination with immune modulators (“imids”). The cardiac safety of carfilzomib and other proteasome inhibitors will become clearer as greater experience accumulates with these therapies.
Immune Checkpoint Inhibitors
Checkpoint proteins serve as “off switches” for the immune system. Tumor cells that bind to the checkpoint proteins are able to deactivate the associated T-cell and avoid the immune response. Checkpoint inhibitors block this interaction, maintaining the T cells in their activated form as they seek out and attack the tumor cells. This new class of chemotherapy has revolutionized the treatment of metastatic melanoma and is now approved for non–small cell lung cancer and renal cell cancer as well. The combination of ipilimumab, an antibody to anticytotoxic T-lymphocyte-associated antigen 4 (CTLA-4), and nivolumab, an antibody to programmed cell death protein 1 (PD-1), has achieved a substantial increase in 3-year survival in the treatment of melanoma. Since checkpoint inhibitors inherently activate the immune system, immune-related adverse events are prevalent. Cardiac toxicity itself has been considered rare, but a growing number of case reports of fulminant myocarditis or serious conduction system issues, at times fatal, have now been reported with combination therapy.
Emerging Cancer Therapies
There are many oncogenic proteins that regulate tumor growth and are targets of ongoing efforts to develop new therapies for cancer. To the extent that these targets may have essential functions in maintaining CV homeostasis, it is easily anticipated that substantial adverse CV effects (including HF) can potentially occur. An obvious challenge for development of drugs targeting these pathways will be the selection of timing and delivery strategies that will limit CV adverse events. Mechanistically, it is apparent that normal myocardial tissue can be protected from brief interruptions in critical pathways regulating metabolism as long as fatty acid, oxygen, and glucose are in ready supply, allowing for adaptive changes in substrate utilization. However, longer interruptions of these essential cellular machinery cascades, and/or alterations during times of metabolic stress (e.g., ischemia, hemodynamic stress, or chronic increases in rate-pressure product) may increase the likelihood of CV dysfunction. Similarly, cancer therapies targeting proteins involved in regulating cell survival may have no deleterious effects in the absence of stressors promoting cell death.
One revolutionary new therapy that has recently been approved by the US Food and Drug Administration (FDA) for two types of leukemia and lymphoma is a type of immunotherapy referred to as chimeric antigen receptor (CAR) T-cell therapy. The therapy works by collecting a patient’s own T cells and engineering them to express a specific CAR that enables them to hone in on cancer cells. The modified T cells are then grown in the lab in significant quantities to be infused back into the patient. It remains to be seen to what degree this more targeted immune therapy may still lead to off-target immune-mediated toxicity.
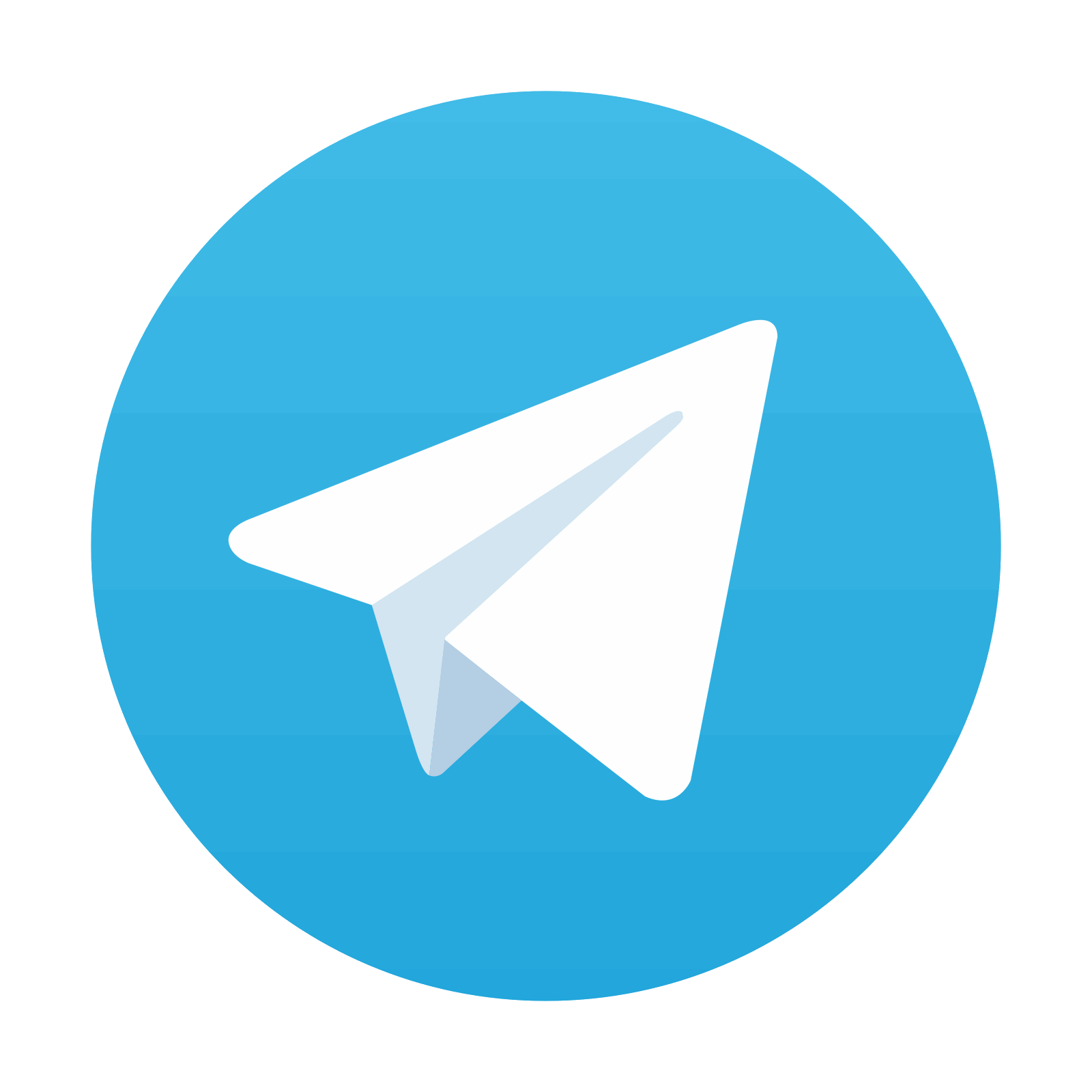
Stay updated, free articles. Join our Telegram channel

Full access? Get Clinical Tree
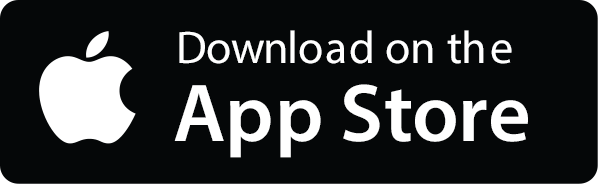
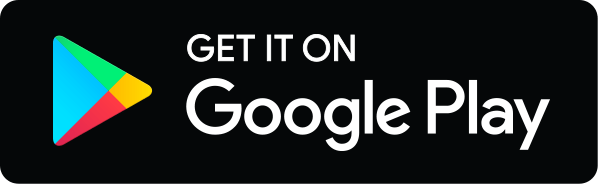