Introduction
Over the past several decades there have been significant improvements in the care of patients diagnosed with cancer, particularly in the pediatric age range. There are more than 15.5 million survivors of cancer in the United States, and in pediatrics specifically, cancer is diagnosed in 15,700 patients younger than 20 years each year ( Table 62.1 ). With current 5-year survival for all types at 80%, this yields approximately 450,000 survivors of pediatric cancer. One unintended consequence of the successes of therapy is a growing population of patients at risk for cancer treatment–related cardiotoxicity (CTC), and cardiovascular disease has emerged as a leading cause of both morbidity and mortality in this population. Survivors are five to six times more likely than sibling controls to develop cardiovascular disease of all etiologies, including: symptomatic heart failure from both systolic and diastolic dysfunction; asymptomatic ventricular dysfunction; valvar disease; coronary disease; arrhythmias; autonomic dysfunction; vascular changes; and pericardial disease.
Children (0–14 y) | Adolescents (15–19 y) | Cumulative Anthracycline Dose a | Potential Thoracic Radiation Exposure Scenarios | |
---|---|---|---|---|
LEUKEMIA | ||||
Acute lymphocytic leukemia | 26% | 8% | Low b | Craniospinal photon radiation |
Acute myeloid leukemia | 5% | 4% | High | – |
LYMPHOMA | ||||
Hodgkin lymphoma | 4% | 15% | Low or high b | Site dependent |
Non-Hodgkin lymphoma | 6% | 8% | Low or high b | Site dependent |
Central nervous system c | 21% | 10% | – | Craniospinal photon radiation |
Neuroblastoma | 7% | – | Low b | Site dependent |
Retinoblastoma | 3% | – | – | – |
Wilms tumor | 5% | – | Low b | Select metastatic patients |
Bone tumors d | 4% | 7% | High | Select metastatic patients |
Soft tissue sarcoma | 7% | 7% | High e | Select metastatic patients |
Germ cell tumors | 3% | 12% | – | – |
Carcinoma and melanoma | 4% | 20% | – | Site dependent |
a High (cumulative ≥250 mg/m 2 ) and low dose (<250 mg/m 2 ) applies to doxorubicin or doxorubicin equivalent of other anthracyclines.
b Anthracyclines included only in select high and intermediate risk regimens, not all treatment protocols.
c Includes ependymoma, astrocytoma, and medulloblastoma.
d Includes osteosarcoma and Ewing sarcoma.
e Anthracyclines included only in select high risk regimens, not all treatment protocols.
Development of Cancer Therapy–Related Cardiotoxicity
The first evidence for CTC was reported shortly after the introduction of anthracycline chemotherapy in the late 1960s and 1970s. Early efforts were focused on management of symptomatic heart failure in survivors but quickly shifted to prevention. There has since been recognition that almost all chemotherapeutic agents pose some cardiovascular risk. Clinical experience shows that there are three types of anthracycline-associated cancer treatment–related cardiotoxicities: acute occurs within the first week of treatment as a transient depression of ventricular systolic function and is usually reversible on discontinuation of therapy ( Fig. 62.1 ; and ); early-onset chronic progressive develops less than 1 year after completion of treatment as dilated and/or restrictive cardiomyopathy and can be progressive; and late-onset chronic progressive, which is similar to early-onset progressive but manifests more than 1 year after treatment is completed ( ). This scheme does not incorporate the other possible negative cardiovascular outcomes, nor does it necessarily apply to other drugs or treatment modalities.

Mechanisms of Cancer Treatment–Related Cardiotoxicity
Among the agents found to cause cardiotoxic effects are: anthracyclines, antimetabolites (e.g., 5-fluorouracil, methotrexate), antimicrotubule agents (e.g., paclitaxel), alkylating agents (e.g., cyclophosphamide, busulfan), small molecules (e.g., sorafenib), monoclonal antibodies (e.g., trastuzamab, rituximab), interleukins, and other miscellaneous agents used less frequently. Successful pediatric cancer treatment requires a risk-adapted, multimodal approach. Specific therapy regimen choices are determined by the patient’s specific molecular diagnosis, clinical features, site of disease, and risk determinants (e.g., stage, grade) (see Table 62.1 ). The preponderance of data pertaining to mechanism have been generated in relation to the anthracyclines. A number of widely disparate pathways are involved, including mitochondrial DNA damage, apoptosis from generation of reactive oxygen species, and damage to nuclear DNA through direct interaction of anthracyclines on topoisomerase 2β ( Fig. 62.2 ). Over time the “safe” threshold for anthracycline exposure has steadily decreased, with studies demonstrating structural changes detectable by echocardiography even after single treatments. However, the current generally accepted definition of low- versus high-dose anthracycline exposure is 250 mg/m 2 doxorubicin equivalent.

Radiation therapy also poses increased risk for CTC, accounting for injury to the pericardium, coronary arteries, conduction pathways, and the myocardium—often manifest as diastolic dysfunction. Similar to anthracyclines, there is a dose dependence of injury; however, this seems mostly to affect timing of manifestation, and it has not been established whether there is a lower limit below which injury does not occur. Mechanisms of injury include induction of proinflammatory cascades, endothelial injury, generation of fibrosis, and initiation of oxidative stress.
Patient and Treatment Risk Factors
The majority of information on development of CTC in children comes from studies on adult long-term survivors of pediatric cancers. The Childhood Cancer Survivor Study (CCSS), a cohort of more than 35,000 survivors of childhood cancer and 5000 sibling controls, has been a wealth of information in this regard. Based on the CCSS and other studies, patient- and treatment-related risk factors have been identified ( Box 62.1 ). Patient factors include younger age, female gender, African-American race, and underlying heart disease. Some of the strongest treatment factors are total anthracycline dose, concomitant radiation exposure, and time since therapy. Data from the CCSS report that survivors of childhood cancer are five to six times more likely than controls to develop congestive heart failure, pericardial disease, myocardial infarction, or valvar abnormalities ( Fig. 62.3 ). The incidence of symptomatic heart failure is as high as 12% in patients with multiple risk factors and receiving higher dose anthracyclines. When cardiomyopathy is defined as left ventricular ejection fraction (EF) less than 50%, 7.5% of all survivors are affected with most being asymptomatic.
Patient Related
- ■
Younger age (especially <5 years)
- ■
Female gender
- ■
African-American race
- ■
Trisomy 21
- ■
Cardiovascular risk factors (hypertension, hyperlipidemia, diabetes, obesity)
- ■
Underlying heart disease (congenital heart disease, cardiomyopathy)
- ■
Genetic factors a
a Multiple genotypes identified as risk factors.
Treatment Related
- ■
Total cumulative anthracycline dose b
b Dose cut-off frequently cited as >250 mg/m 2 doxorubicin equivalent.
- ■
Chest radiation c
c Dose cut-off frequently cited as >15–30 Gy chest radiation.
- ■
Time since treatment
- ■
Premodern protocols (before 1975)
- ■
Concomitant therapy with cyclophosphamide, bleomycin, vincristine, amsacrine, mitoxantrone, immunotherapy

Surveillance of Patients Through Therapy and Beyond
Several guidelines exist for monitoring adult patients undergoing cancer therapy, with pathways including robust cardiovascular assessment from diagnosis through survivorship. In pediatric and adolescent patients there are no standardized guidelines for monitoring during therapy, and there is variation between treatment protocols. More regimented surveillance is taken up once therapy is completed, with long-term follow-up directed by the Children’s Oncology Group (COG) ( www.survivorshipguidelines.org ). These guidelines call for: yearly history and physical exam by a physician; laboratory investigations including lipid profile and blood glucose every 2 years; electrocardiogram at entry into long-term follow-up and repeated as necessary; and echocardiogram at intervals of every 1 to 5 years based on a number of factors including age at treatment, total cumulative dose, and whether radiation treatment was concomitant. As yet, there is no inclusion of cardiac serum biomarkers or imaging beyond basic measures of systolic function. If abnormalities arise or a patient has symptoms concerning for cardiovascular disease, referral to a cardiologist is recommended. Cost analysis of the COG guidelines based on the CCSS cohort showed that a certain portion of the cohort could undergo less-frequent screening than currently recommended at a similar health benefit but that the current recommendations do fall within range of value generally accepted as reasonable.
Studies in adult patients have shown that detailed surveillance during and immediately after anthracycline treatment demonstrates subclinical asymptomatic ventricular dysfunction in approximately 10% of patients, with the highest incidence in the first year of treatment. The importance of early identification of cancer treatment–related cardiotoxicity was illustrated in another study of adult patients who had improved response to cardiovascular treatment following early diagnosis and initiation of treatment, with delay in treatment of just 1 to 2 months significantly reducing the proportion of patients responsive to therapy.
Electrocardiography
Most chemotherapy protocols include serial assessment of the ECG, with particular emphasis on the corrected QT interval. Avoidance of QT-prolonging medications and monitoring of electrolytes is essential with certain therapies to reduce likelihood of the development of potentially fatal ventricular arrhythmia such as torsades de pointes, especially in patients at risk for therapy-related diarrhea and emesis.
Echocardiography
Traditionally, studies of CTC in pediatric populations have been based on shortening fraction, and many chemotherapy protocols continue to include this as the sole marker to identify changes of concern. More recently, EF has been incorporated but is not universally used. There is no specified echocardiographic protocol included in COG guidelines, only that an “echocardiogram or comparable imaging should be performed to evaluate cardiac anatomy and function.” Guidelines are available for the multimodality imaging assessment of adult patients throughout cancer therapy, and the cardio-oncology echocardiogram protocol included can be used as a guide for pediatric patients. Included are two-dimensional (2D) and three-dimensional (3D) assessments of function, strain imaging, and measures of right ventricular function. Both 3D EF and parameters of tissue deformation (strain and tissue Doppler) have demonstrated the ability to detect subclinical dysfunction in adult and pediatric studies. Despite this, the majority of current screening efforts in pediatric patients focus on standard echocardiographic measures and only in long-term survivors. One recent study did detail abnormal myocardial strain in a heterogeneous group of 25 pediatric patients immediately after their final treatment but did not include time points during treatment. Unfortunately, as opposed to studies in adults, those in children and adolescents have yet not proven the ability of changes in myocardial strain to predict later changes in symptomatic heart failure or even EF.
Cardiac Magnetic Resonance
Cardiac magnetic resonance (CMR) has increasing utility in detecting cancer treatment–related cardiotoxicity, particularly in patients with limited imaging windows and for assessment of the right ventricle. One study of long-term survivors of pediatric cancers found abnormal left and right ventricular function (EF <55%) in approximately 80% of the cohort at almost 8 years post therapy, including dysfunction that would meet most treatment criteria (left ventricular EF <45%) in nearly 20%. This proportion of dysfunction was similar to another study of adult survivors showing nearly 15% had left ventricular EF less than 50% by CMR, with 75% of this group misclassified as left ventricular EF greater than 50% by 2D echocardiography. In another group of asymptomatic survivors at least 2 years after therapy, increased extracellular volume, suggesting fibrosis, was demonstrated even in the setting of normal left ventricular EF. This increase in extracellular volume correlated with decreased VO 2 , suggesting a much greater potential burden of cancer treatment–related cardiotoxicity than previously realized.
Serum Biomarkers
Serum biomarkers serve as measurable surrogates for disease presence, progression, or severity. Although recommended in the routine assessment of adult patients, cardiac biomarkers have not yet been incorporated to surveillance for pediatric and adolescent patients. Troponins, brain natriuretic peptide (BNP), and N-terminal pro-BNP have been shown abnormally elevated before initiation of therapy, indicating a baseline state of cardiac stress or injury related to illness, with further increases during and after anthracycline treatment. In one study, children diagnosed with acute lymphoblastic leukemia had elevated troponins after anthracycline, but not nonanthracycline, therapy. In another study of children undergoing cancer therapy, plasma BNP was significantly greater when shortening fraction was decreased, and N-terminal pro-BNP values were elevated in patients receiving anthracycline when compared with controls or nonanthracycline chemotherapy. Patients in long-term follow-up may continue to show elevations in these biomarkers as evidence for ongoing or subclinical cardiotoxicity. A search for new serum biomarkers is ongoing, including early data suggesting promise for circulating microRNAs as monitoring tools in pediatric patients. There are also gene polymorphisms described that may identify susceptibility to CTC for individuals with a given genotype. Ultimately, a combination of imaging, serum biomarkers, and genetic profile is likely to yield the most sensitive method to either predict or detect CTC during and after therapy.
Other Modalities
Exercise Testing and Stress Echocardiography
Cardiopulmonary exercise testing (CPET) allows insight to a patient’s cardiac function, cardiovascular symptoms, and aerobic fitness under the stress of exercise. Studies have demonstrated decrease in exercise capacity by CPET, and subclinical myocardial systolic and diastolic dysfunction by exercise echocardiography, when comparing survivors of childhood cancers to healthy controls. Testing with CPET also has potential to identify ischemic disease and dysrhythmias.
Multigated Radionuclide Angiography
Use of multigated radionuclide angiography (MUGA) has been incorporated into adult monitoring, generally for its reproducibility and accuracy at measuring EF when compared with 2D echocadriography. However, with its limited ability to give structural information, poor characterization of the right heart, use of radiation, and the emergence of 3D echocardiography and CMR, MUGA is used less frequently overall and rarely in children.
Ambulatory Rhythm Monitoring
The need for extended rhythm monitoring (Holter monitor, event monitor, loop recorder) is not specifically addressed in follow-up guidelines for pediatric patients. Inclusion is at the discretion of the specialist based on history, exam, or ECG findings.
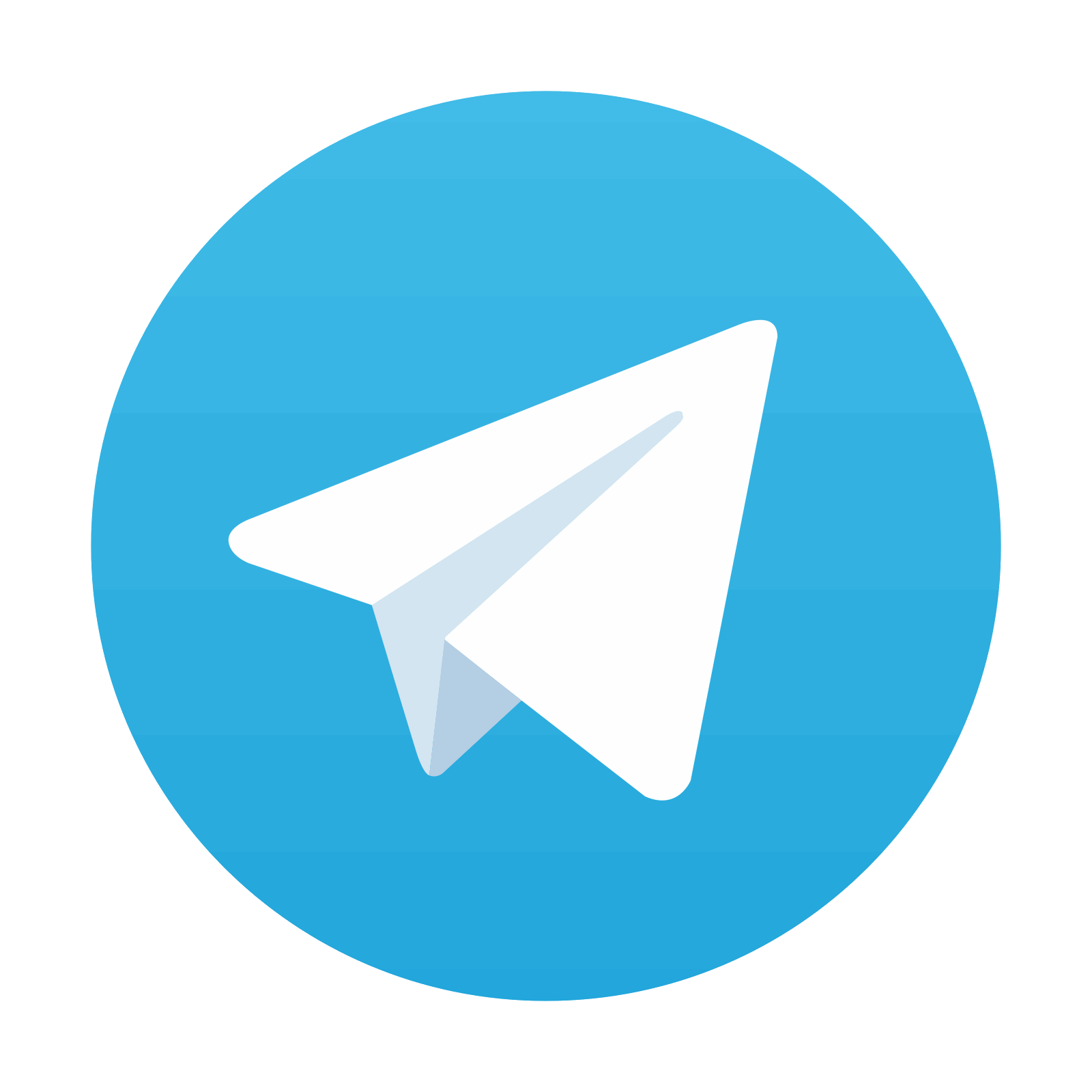
Stay updated, free articles. Join our Telegram channel

Full access? Get Clinical Tree
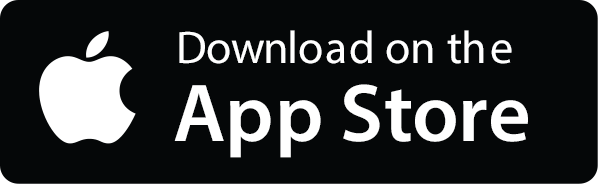
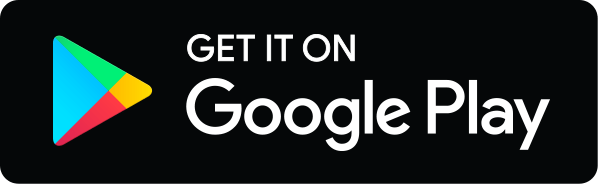