Approach
Availabilitya
Costb
Immune barrierc
Infectious barrierd
Global availabilitye
Allotransplant
*
**
***
****
***
Assist device
***
**
**
**
**
Artificial heart
**
**
**
***
**
Engineeringf
*
****
*
*
*
Organogenesisg
*
****
*
*
*
Xenotransplant
****
*
****
*
****
These barriers, with the exception of infection, would seem to be minimized if nonhuman primates were used as sources of hearts. However, despite this logic, nonhuman primates are no longer considered for such use because the number of hearts of suitable size would be too small to address the shortage of human hearts and because nonhuman primates might convey and their organs propagate novel infectious agents. Instead, swine are considered the most suitable species to use as a source of organ xenografts. Swine are favored because (1) hearts of suitable size can be obtained in large numbers, (2) they can be bred and genetically engineered to lower biological barriers, (3) breeding (and cloning) can generate genetically uniform transplants with definable properties, and (4) use of swine organs could be planned and might engender lower costs than alternatives.
While swine might harbor some microorganisms capable of transfer to humans, these organisms are better known and “less threatening” than those found in nonhuman primates. And in contrast to the use of human hearts, the use of swine as a source of hearts enables exclusion of known pathogens by breeding in a controlled environment, testing, and, if necessary, pretreating either the recipient or the animal source.
Xenogeneic Cell and Tissue Transplants . Cellular transplants of various types are being explored for treatment of cardiac disease [26–29], although the benefits and mechanisms underlying these benefits remain subjects of debate [26, 27, 30]. One question is whether cell transplants, and particularly stem cell transplants, regenerate myocardium directly or establish a microenvironment that facilitates regeneration. This question has practical implications because allogeneic cells, even without immunosuppression, have exhibited benefit, implying that the benefit reflects a transient impact on the microenvironment [26, 31].
If cell transplants need only deliver temporary impact, and if immunity to the transplant is not detrimental, then xenogeneic sources of cell transplants might merit consideration. As discussed below, xenogeneic cell and tissue transplants are usually not susceptible to the most explosive types of rejection (hyperacute and acute vascular rejection) seen in organ transplants (◘ Fig. 34.1). Besides the ready availability and potentially uniform properties of xenogeneic cells, the cells could be obtained from animals genetically engineered to increase production of beneficial substances. Unlike allogeneic cells, the potential for oncogenicity is probably nil.


Fig. 34.1
Susceptibility of organ xenografts and cell and tissue xenografts to humoral and cellular immunity. Organ xenografts have a vascular system full of animal origin (black); therefore, the blood vessels are readily attacked by antibodies of the recipient (red). Bound antibodies activate complement of the recipient because heterologous complement regulatory proteins (CRPs) fail to control the complement cascade. Cell and tissue xenografts have blood vessels formed by ingrowth of the recipient’s blood vessels. These recipient blood vessels are not targeted by recipient antibodies. Complement of the recipient is effectively controlled by the recipient’s CRP. Both organ xenografts and cell and tissue xenografts are susceptible cell-mediated immunity because the recipient’s lymphocytes and monocytes actively migrate between adjacent endothelial cells
Tissue Engineering Using Xenogeneic Organ as Scaffold. Beyond the established uses of decellularized xenogeneic valves, decellularized xenogeneic organs have been envisioned as scaffolds for seeding with cells and engineering whole organs [32–34]. Besides availability, xenogeneic tissue and organs might be preferred for this purpose because the properties of the biological source can be controlled, potentially made uniform, and genetically engineered. Acellular organ scaffolds, like acellular valve tissue, would probably evoke less immunity and suffer less injury than living tissues. (Acellular tissue is not a true xenograft.) However, the extent of engineering and costs needed to generate a full-size, fully functioning “hybrid heart” for clinical use might still pose daunting hurdles to clinical application.
Reverse Xenotransplants . In what might be called a reverse xenotransplant, animals might also be used to generate human tissue or organs (i.e., organogenesis) from stem cells or primordia (◘ Fig. 34.2). As one example, stem cells from an individual needing treatment might be engrafted into fetal animals to coax differentiation of an embryonic organ. At a suitable stage of development, the organ rudiment would be engrafted in a heterotopic site and development completed in the individual to be treated [5, 11, 35]. The developed tissues could be used to repair or replace the diseased organ.


Fig. 34.2
Organogenesis by “reverse xenotransplantation.” Limitations to using stem cells for generating whole organs may be addressed by reverse xenotransplantation. A patient’s cells are harvested and used to generate pluripotent stem cells. The cells are introduced into a fetal animal where they can be induced and coaxed to generate an organ primordium. The human organ primordium is harvested and cells are introduced into the patient where an organ can form, mature, and become vascularized. Each of these steps has been taken successfully in one or another experimental system, but whether it can succeed as a strategy and at what cost are unknown
Although the overall model is theoretical, each of the steps in this approach has been carried out successfully in one or another system. Besides complexity, the major limits to this approach include the need to assure that the new organ is vascularized by the human recipient rather than the temporary host animal (to avoid vascular rejection), the time required for the organ to develop and mature, and the extraordinary costs for each of the steps.
Immunological Barriers to Xenotransplantation
Natural Immunity. The initial barrier to xenotransplantation is called “natural” because it engages effector components of the immune system that are fully active at the time of transplantation and are not evoked by exposure to a foreign antigen. These effector systems include “natural antibodies,” natural killer (NK) cells, phagocytes, and complement.
Individuals of the same species inherit many distinct gene variants and, therefore, produce many allotypic molecules. Despite these differences, only natural immunity poses a significant barrier to organ allotransplantation. Individuals of different species differ at every genetic locus and have detectable, functionally significant differences in many macromolecules. Yet the natural immune barrier to organ xenotransplantation appears to reflect differences at only a few genetic loci, mentioned below. For this reason, genetic engineering of animals can potentially lower or eliminate the innate immune barrier to xenotransplantation, a possibility that has aroused much interest.
Xenoreactive natural antibodies that recognize cells of other species can be found in the blood of all immune-competent individuals [36]. These antibodies have the same properties as the natural antibodies that recognize blood group saccharides [37].
Xenoreactive natural antibodies in humans, apes, and old-world monkeys predominantly recognize a disaccharide—galactose-α-1,3-galactose (Galα1-3Gal)—that terminates complex carbohydrate substitutions on glycoproteins and glycolipids of nonhuman primates, such as pigs, and also new-world monkeys [38–42]. While other natural antibody-antigen combinations exist, Galα1-3Gal and anti-Galα1-3Gal constitute the main initial barrier to cardiac xenotransplantation, since removal of the antibodies against this sugar prevents hyperacute rejection of cardiac xenografts [43]. Exposure to Galα1-3Gal and inflammation attendant to transplantation causes increased production of the antibodies and isotype switching [44]; however, “affinity maturation” of these antibodies probably reflects selection of existing clones more than somatic hypermutation. Genetic engineering of swine solved the problem of natural anti-Galα1-3Gal antibodies by eliminating the enzyme α1,3-galactosyltransferase, which catalyzes production of Galα1-3Gal (45). Organs from the animals that cannot produce Galα1-3Gal (sometimes inaptly called “αGal-KO” animals) do not undergo hyperacute rejection after transplantation into nonhuman primates [46, 47].
The immune barrier posed by natural antibodies depends absolutely on the activation of complement, as the complement system presents the most daunting natural immune barrier to cardiac xenotransplantation . Complement recognizes foreign and/or damaged cells and causes the cells to be further injured, killed, engulfed, and/or used to stimulate inflammation and immunity.
Human complement recognizes swine cells via three potential mechanisms. First, the binding of xenoreactive natural antibodies (or elicited antibodies) to swine cells engages C1q, C1r, and C1s, triggering the classical complement pathway by cleaving C4 and C2. Fragments of C4 attach to cell membranes and associate with fragments of C2, generating an enzyme complex that cleaves C3, continuing and amplifying the activation of complement. Recruitment of the classical complement pathway to swine xenografts in primates depends on binding of xenoreactive natural antibodies [48]. Thus, the pathway does not proceed for the most part in “αGal-KO” organs transplanted into swine.
Second, complement is also activated on foreign cells via the “alternative pathway .” This pathway begins with spontaneous hydrolysis of C3, leading sequentially to formation of C3b, attachment to cells, and formation of an enzyme complex with factor B and factor P, which further activates the complement cascade. Unlike the classical complement pathway, which remains inactive unless triggered, the alternative complement pathway is continuously activated, but full activation is blocked by inhibitors such as decay-accelerating factor (DAF). DAF is expressed on cell surfaces in the graft and factor H, which circulates in the blood. Decay-accelerating factor is so named because it promotes the decay, i.e., dissociation of the classical pathway and alternative pathway enzyme complexes. It dissociates homologous complexes much more efficiently than heterologous complexes. Factor H also dissociates C3b-factor B complexes and promotes cleavage of C3b by factor I. It does so much more effectively on homologous than on heterologous polyanionic surfaces. Factor H functions poorly or not at all on microbial, some xenogeneic, and damaged cell surfaces and on surfaces where the polyanions are blocked (e.g., by bound antibody) or shed. If factor H fails to control C3b-factor B complexes on xenogeneic cell surfaces, the alternative pathway will be activated without restraint, whether or not antibodies are bound [49, 50].
In most swine to human xenografts , factor H effectively regulates C3b-factor B complexes. Therefore, complement activation requires activation of the classical by antibodies (or by the lectin pathway as described below). However, the activity of factor H probably varies enough, with differences in cell surface chemistry, charge, and damage, that increased contribution of the alternative pathway cannot be dismissed without evidence.
Third, complement might be activated on xenogeneic cells through the lectin pathway. Association of mannose-binding lectin (MBL) and/or ficolins with damaged or dying cells (or to microorganisms) triggers the lectin pathway. MBL and ficolins recruit one or more MBL-associated serine proteases (MASPs), especially MASP-1 and MASP-2, to form a complex that cleaves C4 and C2, leading to activation of the rest of the complement cascade as described above. IgM bound to Galα1-3Gal or other targets can recruit MBL and MASP-2, leading to complement activation independent of C1q [51–53]. Given the breadth of potential targets for MBL and ficolins, it seems likely that regulation of the pathway by polyanions and antiproteases determines whether or not that pathway contributes to tissue injury in xenografts.
Natural killer cells and macrophages can also interact with and injure xenogeneic cells, causing researchers recently to question whether they contribute to the pathogenesis of xenograft rejection [54–59]. NK cells can be stimulated when UL16-binding protein or related proteins of swine interact with natural killer group 2, member D (NKG2D), and/or when swine major histocompatibility complex (MHC) class I fails to interact with human killer inhibitory receptors [60–62]. Interactions of human macrophages with swine cells can be promoted by complement fragments and bound IgG and suppressed by failed interaction of swine CD47 with signal-regulatory protein-α (SIRP-α) [63].
NK cells and macrophages are both important in forming an innate immune barrier to cell and tissue transplants. But whether they initiate injury to organ grafts, and to what extent, is not clear. Thus, NK cells and macrophages rapidly (within minutes) injure or kill xenogeneic cells in culture. However, xenogeneic cells are found in xenogeneic heart transplants after periods of weeks or months and accompany other features of graft rejection [64].
Elicited/Adaptive Immunity . With few exceptions, transplants of foreign cell tissues or organs elicit “adaptive” immune responses in the recipient [65]. Unlike innate immunity, adaptive immunity requires activation and proliferation of B and/or T lymphocytes. The first step in activation of B cells and T cells involves the recognition of a foreign antigen (intact antigen in the case of B cells, antigen fragments associated with recipient MHC, or intact foreign MHC itself in the case of T cells) by antigen receptors. Because the repertoires of lymphocyte antigen receptors and, hence, of distinct lymphocyte clones is so vast (approximately 109), each T cell and B cell clone consists of as few as ten cells. Therefore, activation of B cells and T cells must be followed immediately by proliferation to expand by orders of magnitude the number of effector cells.
The bringing together of the few naive lymphocytes bearing receptors for a foreign antigen with that antigen, and expansion of these cells, usually requires 2–6 weeks—sooner if the antigen recruits an unusually large fraction of cells, such as alloreactive T cells. Therefore, the impact of adaptive immunity usually follows that of innate immunity. Nevertheless, antibodies and helper T cells use components of innate immunity, such as complement and macrophages, to exert effector functions.
Several factors might hasten and expand the adaptive immune response to xenografts. Practically every protein in xenografts can elicit an immune response. Some proteins, such as MHC proteins, are highly immunogenic and elicit immunity in practically all individuals. Other proteins are less immunogenic and elicit immunity in some individuals but not in others.
Besides whatever early damage it inflicts on grafts, the powerful innate immune response to xenografts accelerates adaptive immunity. For example, complement-induced tissue injury liberates antigen and activates antigen-presenting cells, increasing antigen processing, association with MHC, and migration to regional lymphoid organs. Heightened inflammation also speeds the migration of T cells to lymphoid organs, accelerating the relatively rare interactions with MHC-antigen complexes.
Activation of complement enables and amplifies B cell responses to an antigen. Even B cell responses thought to be “T cell independent” are hastened because of the availability of polymeric (i.e., carbohydrate) antigen. While the “direct” T cell response to xenogeneic cells might be impeded by incompatibility between CD4 and CD8 of T cells and xenogeneic MHC [66], this incompatibility has little impact on the dimensions of the cellular immune response to xenografts in vivo.
The Impact of Immunity on Xenografts
The intensity of immune responses does not necessarily determine the intensity of tissue injury observed in xenografts (or allografts). The dissociation between immunity and impact pathology reflects two factors—one related to the source of blood vessels feeding the graft and the other to the intrinsic susceptibility of the graft to injury.
Origin of Blood Vessels in the Graft. The impact of immunity on xenografts depends to a considerable extent on the origin of blood vessels in the graft (◘ Figs. 34.1 and 34.3) [11, 67]. Organs such as the heart are transplanted by primary anastomosis of the recipient’s blood vessels with the large blood vessels of the graft (◘ Fig. 34.1). Because of this anastomosis, the endothelial lining of the graft consists entirely of donor cells. With reperfusion, xenoreactive antibodies of the recipient circulating at the time of transplantation and those produced later can bind directly to graft blood vessels, activating complement and recruiting inflammatory cells (◘ Fig. 34.3).


Fig. 34.3
Impact of immunity on cardiac xenografts . Cardiac xenografts are susceptible to hyperacute rejection over hours, acute vascular rejection and cellular rejection over days to weeks, and chronic rejection over months after transplantation. Hyperacute and acute vascular rejection can be countered by accommodation, the acquired resistance of the graft to immune and inflammatory injury. Rejection of every type might be countered by tolerance. In contrast to organ xenografts, cell and tissue xenografts are mainly susceptible to cellular rejection and to injury caused by natural killer cells and macrophages independent of immunity [not shown]
Hyperacute Rejection . The binding of xenoreactive antibodies to blood vessel lining cells and the rapid activation of complement (or direct activation of complement independent of antibodies) can cause hyperacute rejection. As in allografts, hyperacute rejection of xenografts is characterized by platelet thrombi, focal hemorrhage, infarction, and attachment of neutrophils to vessel walls. The pathogenesis of hyperacute rejection of cardiac xenografts depends absolutely on activation of the full complement cascade [68] and probably on the rate that full activation proceeds, since a low level of expression of human DAF, which slows but does not necessarily prevent complement activation in a swine organ, averts the condition [21]. Consistent with that concept, ABO-incompatible organ transplants, in which complement regulators such as DAF, of the donor are compatible with the recipient, do not usually undergo hyperacute rejection. The dramatic difference between the fate of cardiac xenografts and ABO-incompatible allografts exemplifies the importance of susceptibility of a graft to injury.
Acute Vascular Rejection . When hyperacute rejection does not occur or is prevented, a xenogeneic organ becomes subject to acute vascular or antibody-mediated rejection (sometimes called delayed xenograft rejection). Characterized by focal ischemia, swelling of endothelium, and fibrin thrombi, with or without fixation of antibodies and complement to vessel walls, acute vascular rejection typically begins days or weeks after transplantation, but, as seen in allografts, it can occur at later times.
In its earliest manifestation, acute vascular rejection is caused by natural antibodies directed against Galα1-3Gal [69, 70]. Later, acute vascular rejection is caused by antibodies elicited de novo after transplantation [71], although some of the targets might be the same as those recognized by natural antibodies [72]. Acute vascular rejection is associated with endothelial cell activation caused by small amounts of complement that escape control by complement inhibitory agents or expression of human complement regulatory proteins [73–76].
Endothelial cell activation and acute vascular rejection may also be caused by interactions of inflammatory cells or platelets with endothelium [77, 78]. However, activation of complement in small amounts, owing to incompatibilities of complement regulatory proteins or binding of small amounts of anti-swine antibodies, would probably activate the inflammatory cells and the endothelium, facilitating interactions.
Acute vascular rejection is the main biological barrier to clinical application of cardiac xenotransplantation.
Cellular Rejection . Xenografts, like allografts, are subject to cellular rejection and, presumably, to chronic rejection or coronary vasculopathy. While cellular rejection might be more aggressive, for reasons discussed above, its control by available immunosuppressive drugs makes it unlikely to cause loss of xenografts. An important question, however, is whether the recipient of a cardiac xenograft would be broadly sensitized to human leukocyte antigen (HLA). Broad sensitization to HLA and associated ineligibility for receiving most allografts might be considered a serious hindrance to using a xenograft as a “bridge” to allotransplantation. Investigation of a limited number of human subjects and nonhuman primates exposed to swine tissues and organs has not revealed consistent evidence of such “cross sensitization”; however, the risk has not been excluded.
Chronic Rejection . Whether chronic rejection would impair function and limit survival of cardiac xenografts has been a matter of conjecture because experimental cardiac xenografts succumbed to acute vascular rejection before chronic coronary vasculopathy could develop. Still, chronic rejection of heterotopic cardiac xenografts has been described [64], but whether this condition is more likely to arise, as suspected, or not is unknown.
Cell and Tissue Xenografts . Cell and tissue grafts derive their vascular supply from the ingrowth of the recipient’s blood vessels. The full capillary network of cellular grafts is of recipient origin. The capillary network of tissue grafts is partly or fully of recipient origin. Hence, xenoreactive antibodies and complement do not bind directly to blood vessels of cell and tissue grafts and penetrate the vessels slowly, if at all (◘ Fig. 34.1). Small amounts of heterologous complement produced in the graft are presumably controlled by the complement regulatory proteins of the graft. The limited availability of antibodies and complement of the recipient thus prevents antibody-mediated rejection of cell and tissue grafts [23, 67, 79]. On the other hand, cell and tissue xenografts are fully susceptible to cellular rejection because the recipient’s activated T cells and phagocytes migrate actively through blood vessel walls.
Accommodation . Binding of xenoreactive antibodies and fixation of complement in transplanted organs sometimes induce resistance to injury by these or other components of the immune system. Acquired resistance to immune and inflammatory injury has been called accommodation [20, 80, 81]. What exactly induces accommodation in xeno- and allotransplantation is not completely known. But investigation of cells in culture indicates that exposure to subtoxic levels of xenoreactive antibodies and complement induces changes that enable the cells to resist subsequent exposure to higher, toxic levels [82–84].
The resistance described in cultured cells probably occurs in accommodated organs, as well [73, 82, 85, 86]. However, systemic and regional factors such as availability of hormones and vasoactive substances also determine the well-being of organs [87–89]. In any case, accommodation is another example of how the susceptibility of a transplant to injury determines the impact of immunity on a graft.
Overcoming Immune Barriers to Xenotransplantation
Genetic Engineering , Cloning, and Breeding. Xenotransplantation makes it possible to use several approaches to “designing” and optimizing the source organs and tissues used for transplantation into humans. These approaches include adding genes, i.e., transgenesis; cloning, which enables targeting as well as adding of genes; and breeding to optimize existing sets of genes and to generate more uniform lines of animals [20, 90–92].
Genes encoding human complement regulatory proteins have been introduced in swine to overcome the incompatibility of swine complement regulatory proteins with human complement [21, 93]. Expression of even low levels of human decay-accelerating factor (CD55) or CD46 in transgenic pigs prevents hyperacute rejection when the organs of these pigs are transplanted into nonhuman primates (as a model for humans). Similar approaches are being used to express regulators of coagulation because coagulation is often seen in rejecting xenografts. However, the benefit has yet to be proven.
Several approaches to reproductive cloning have been used in conjunction with gene targeting in stem cells to generate swine with nonfunctional or absent α1,3-galactosyltransferase genes. These swine do not produce Galα1-3Gal [45, 94], the main antigen recognized by human xenoreactive natural antibodies. As might be expected, recipients of organs from these “Gal-KO” animals do not undergo hyperacute rejection. Treatment of recipients with maximum amounts of immunosuppressive drugs has enabled survival of heterotopic cardiac xenografts in nonhuman primates for 6 months and with other genetic manipulations for more than a year [24, 46].
While these results were achieved in nonfunctioning (heterotopic) model systems, it is entirely possible that equal or better results could be obtained in clinical trials because the transgenes introduced in swine were partially incompatible with nonhuman primates and because the level of monitoring and medical care available for experimental systems cannot approach those used in clinical practice.
Immune Therapeutics . All or nearly all immunosuppressive drugs and biologics used in allotransplantation have been tested in xenotransplantation models. Immunosuppressive drugs appear to effectively prevent early cellular rejection of most swine to primate cardiac xenografts. However, the drugs do not appear to avert T cell-dependent B cell responses. Nor do they necessarily do so in allotransplantation [95]. Their ability to effectively suppress cellular rejection over periods of months and years has not been explored. While the drugs and biologics routinely used in clinical practice do not reliably prolong survival of cardiac xenografts, these drugs and biologics were developed and optimized for use in humans, so they will likely prove more effective in human subjects than in nonhuman primates.
Induction of Tolerance . The failure of existing immune therapeutics to control the immune response to xenotransplantation has led some to conclude that clinical application of xenotransplantation might depend on induction of tolerance to swine antigens [96]. Induction of tolerance to antigens in xenografts would seem to pose a substantially greater hurdle than induction of tolerance to antigens in allografts because all xenogeneic proteins and some carbohydrates are potential targets of immunity and because innate immunity aggravates responses to these antigens .
On the other hand, tolerance to a xenograft might be induced more readily or at least with no greater difficulty than tolerance to an allograft for several reasons. First, if cloned or highly inbred swine are used as sources of xenografts, key antigens, once identified, might be suppressed, modified, or masked by genetic engineering or pretreatment of swine.
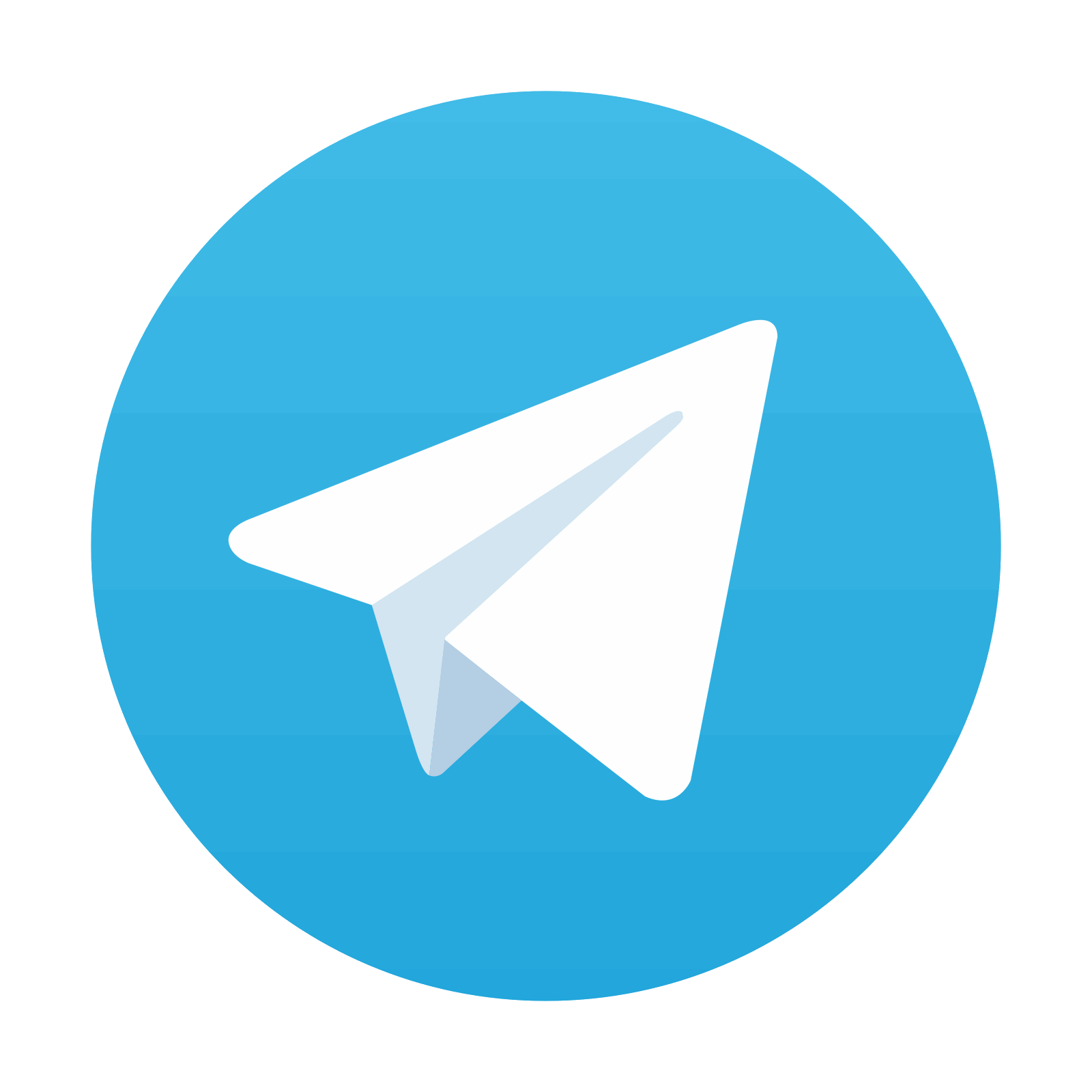
Stay updated, free articles. Join our Telegram channel

Full access? Get Clinical Tree
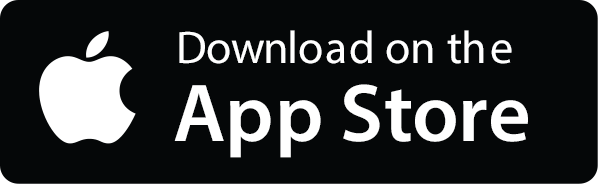
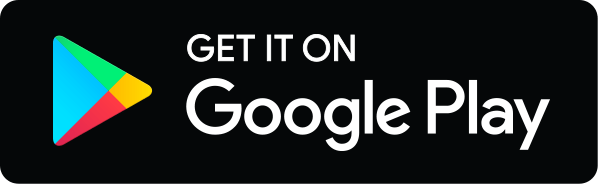