Key points
- •
PET imaging is ideal for the quantitative assessment of myocardial blood flow, metabolism, viability, and innervation.
- •
PET imaging can be done with or without on-site cyclotrons, but the tracers are different.
- •
The radiation exposure from PET studies is generally less than that of SPECT studies.
- •
Attenuation correction is routinely used for PET imaging, unlike SPECT imaging, where it is optional and not widely used.
- •
Misregistration artifacts have become more prevalent with the advent of hybrid cameras because of the difference in temporal resolution between the PET and the CT image. Artifactual count losses may develop if lung tissue on the CT image overlays LV wall activity on the PET image and there is not proper alignment of the images prior to reconstruction.
- •
Overcorrection of the inferior wall is another artifact that results from the difference in scanning times between PET and CT images. This artifact is typically seen in patients with hypoinflated lungs on CT imaging.
- •
Thirteen percent of rubidium decays are associated with a prompt-gamma that correlates in time with the annihilation photons. Prompt-gammas may represent a significant problem when imaging in the 3D mode. Artifactual defects may develop in the PET image if there is no adequate correction for these unwanted events in the reconstruction algorithm.
- •
N-13-ammonia has favorable physical characteristics over rubidium. However, it has not entered the mainstream of nuclear cardiology because it requires an on-site cyclotron for production.
- •
F-18 flurpiridaz is a new tracer developed in an attempt to allow exercise stress testing in myocardial perfusion PET and to facilitate the quantification of myocardial blood flow. The diagnostic performance of F-18 flurpiridaz myocardial perfusion PET has not yet been determined.
- •
The image quality is improved with glucose-loading protocols relative to fasting protocols in FDG myocardial viability imaging. Glucose-loading protocols stimulate the release of endogenous insulin, decreasing plasma levels of free fatty acids and optimizing the myocardial transport and utilization of FDG. As a result, it decreases the blood pool activity and improves image contrast.
Background
There has been an exponential growth in cardiac PET imaging in the past decade. The growing interest in this technology is a result of the increased availability of PET cameras, changes in reimbursement, and the scientific evidence documenting its clinical efficacy in the evaluation of patients with CAD. In this chapter, we will illustrate common artifacts in cardiac PET imaging, briefly review the physical characteristics and kinetics of myocardial perfusion PET tracers, and discuss the differences between fasting and glucose-loading protocols in FDG viability imaging.
Case 20-1
PET/CT Artifact: LV–Lung Mismatch ( Figure 20-1 )
A 53-year-old woman presented to the ED with new-onset atypical angina. Her risk factors included hyperlipidemia, hypertension, and family history of CAD. She was first ruled out for MI by ECG and serial troponin I. Subsequently she was referred for rubidium myocardial perfusion PET. The physical findings were remarkable for obesity (weight 230 lb, BMI 35 kg/m 2 ) and hypertension. She underwent vasodilator stress testing with regadenoson. The ECG showed sinus rhythm at baseline. Stress ECG showed an isolated PVC and no ischemic changes. The images were obtained using a PET/CT camera operating in the 3D mode.




Comments
Hybrid PET/CT cameras are rapidly replacing stand-alone PET cameras in cardiac imaging. While on stand-alone cameras, rotating sources (germanium or cesium) are used to obtain the attenuation image, on hybrid cameras, the CT image is used for PET attenuation correction. This reduces the attenuation scan time from minutes (3 to 10 minutes) to seconds (5 to 20 seconds). The PET image is averaged over a few minutes (about 5 minutes for rubidium). Because the CT scan time is short, sometimes the image does not match the LV–lung interface of the PET image. This increases the emission-transmission misalignment rate in cardiac PET. If the images are not properly aligned prior to reconstruction, perfusion defects may develop. The artificially low attenuation value of the LV wall on the CT image (lung tissue instead of soft tissue) decreases the expected photon attenuation and scatter from that region. When the images are reconstructed, the count density of the misregistered LV wall is artifactually decreased.
The original reconstructed PET images show a large reversible defect of moderate severity in the anterolateral wall. The stress perfusion defect is secondary to misalignment of the PET and CT images in the LV–lung interface. There is lung tissue on CT images overlaying LV activity in the anterolateral wall on PET images. Registration of the stress images was subsequently performed using an emission-driven method. This method is proprietary and not commercially available. It modifies the heart outline of the CT image based on the PET data. The program expects to find soft tissue attenuation on the CT image if the corresponding voxel on the PET image contains LV wall activity. If there is an inconsistency and lung tissue on the CT image overlays LV wall activity on the PET image, the attenuation value of the voxel is modified to match that of soft tissue. Following registration and reconstruction of the images, the PET images are normal.
Different CT protocols have been proposed in an attempt to minimize the emission-transmission misregistration rate on PET/CT imaging. At our institution, the “solution” to this problem has been to coach the patients to shallow breathe during the acquisition and to average the CT data over a longer time by slowing the rotational speed of the gantry and increasing the pitch. Despite these efforts, the CT attenuation scan time on multislice CT scanners is still typically under 20 seconds. We have tested other CT protocols including ECG-gated (with breath-hold), ungated (with and without breath-hold), “slow,” “fast,” and cine CT. The misregistration rate was highest with ECG-gated breath-hold CT (about 60%) and lowest with cine CT and “slow” ungated CT (about 20% each). Regardless of the CT protocol used for attenuation correction, it is important that the reader remembers to review the quality control images. The fused images should be available at the time of interpretation to ensure proper registration was performed prior to image reconstruction.
Case 20-2
PET/CT Artifact: LV–Diaphragm Mismatch ( Figure 20-2 )
A 42-year-old man was referred for rubidium myocardial perfusion PET because of worsening shortness of breath on exertion. He had type 2 diabetes and hyperlipidemia. Calcium scoring CT performed 1 year prior showed a total Agatston score of 102. He underwent vasodilator stress testing with regadenoson. There were no ST changes on ECG. The images were obtained using a PET/CT camera operating in 3D mode.


Comments
The PET images in this patient show relatively increased uptake in the inferior wall, which is slightly more pronounced on the stress images. Fused stress PET/CT attenuation images reveal no LV–lung mismatch. However, there is LV–diaphragm mismatch. The lungs on the CT image are hypoinflated relative to the averaged lung volumes of the PET image. The elevation of the diaphragm on the CT image increases the expected photon attenuation from tissues in the same axial plane of the inferior LV wall because lung attenuation is replaced by soft tissue attenuation from the subdiaphragmatic organs. As a result, the reconstructed PET images show an artifactually increased count density in the inferior LV wall.
The LV–diaphragm mismatch is less prevalent than the LV–lung mismatch; it occurs in about 5% of cases at our institution. When severe, the “hot spot” in the inferior wall can produce downscaling in the remainder of the LV and artifactual perfusion defects may develop in the LAD and LCX distributions. These artifactual defects are sometimes “reversible,” particularly when the patient hyperventilates during the stress PET acquisition.
Can we prevent “reversible” artifacts by performing a CT scan post-stress for stress PET attenuation correction? At our institution, we use the same rest CT image for rest and stress PET attenuation correction. We have not been able to demonstrate an improvement in registration rate of the stress images by adding a CT attenuation scan post-stress. This is because we use regadenoson for pharmacologic stress testing. By the time the stress PET acquisition is finished and a second CT attenuation scan is obtained, hyperventilation and tachycardia have usually subsided (the same applies for adenosine). As a result, the CT image poststress is essentially another rest CT attenuation scan that does not improve the stress PET image quality.
Case 20-3
Rubidium 3D PET Artifact: Prompt-Gammas ( Figure 20-3 )
A 63-year-old woman was referred for rubidium myocardial perfusion PET because of one episode of unexplained syncope. Her risk factors for CAD were hypertension and hyperlipidemia. She underwent vasodilator stress testing with adenosine. Baseline ECG showed sinus rhythm, no Q waves, and no ST-T abnormalities. Stress ECG was unremarkable. The images were obtained using a PET/CT camera operating in 3D mode.




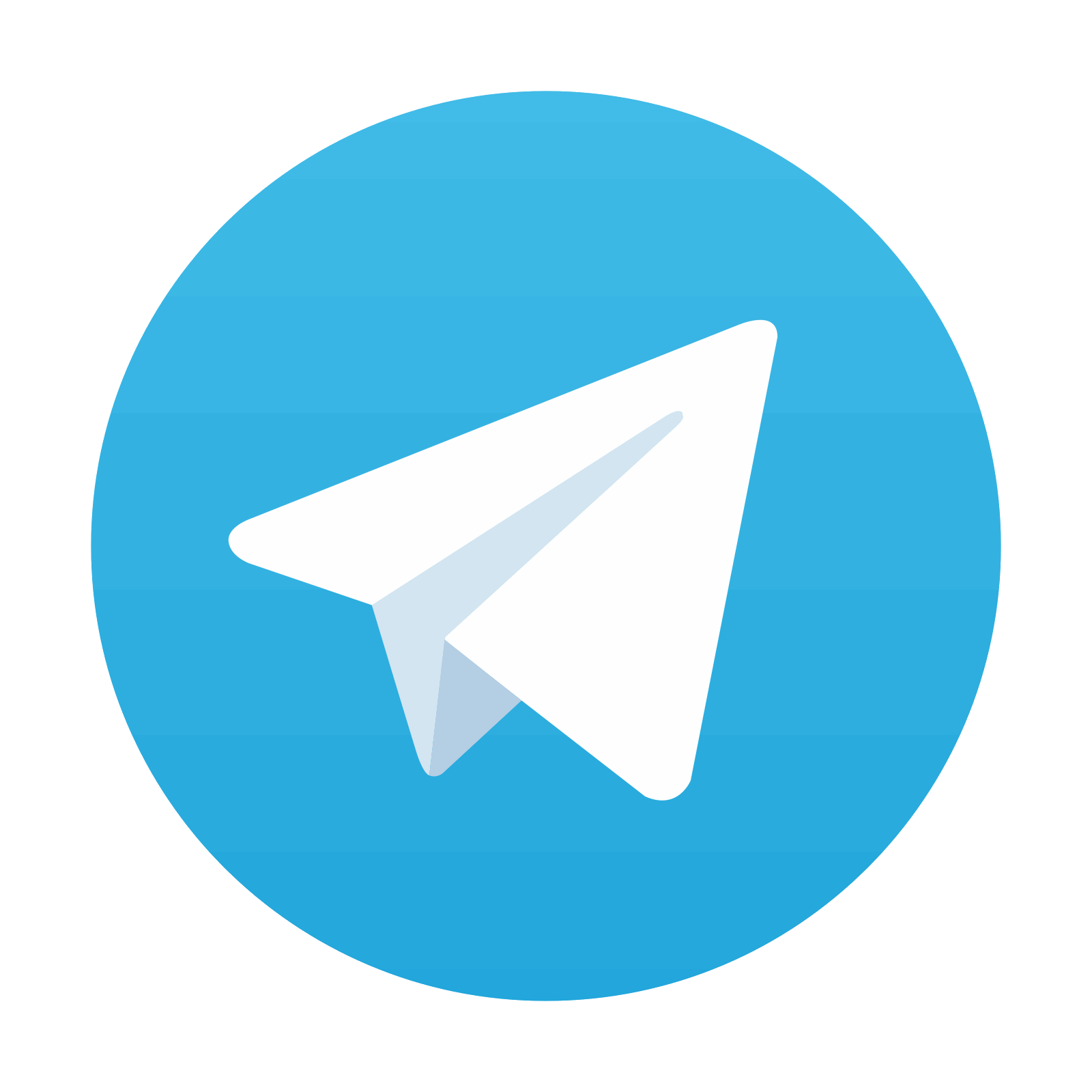
Stay updated, free articles. Join our Telegram channel

Full access? Get Clinical Tree
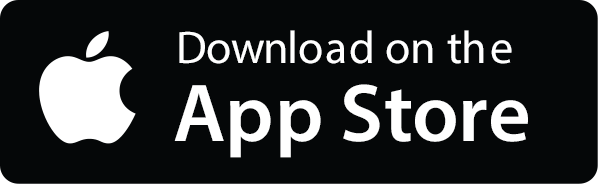
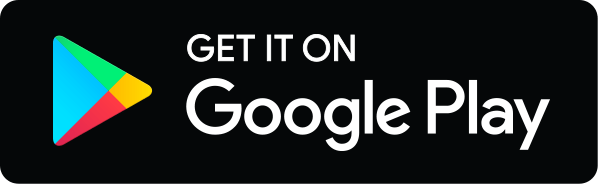
