1. Anthracyclines — Doxorubicin, Daunorubicin, Epirubicin, Idarubicin, Mitoxantrone
2. Monoclonal antibodies against HER2 — Trastuzumab
3. Alkylating agents — Cyclophosphamide, Ifosfamide
4. Antimicrotubule agents — Paclitaxel
5. Angiogenesis inhibitors — Bevacizumab
6. Tyrosine kinase inhibitors — Sunitinib, sorefinib, imatinib, lapatinib
7. Pyrimidine analogues — 5-Flourouracil, Capecitabine
Chemotherapy-induced cardiomyopathy has been broadly classified into two types- Type I (non-reversible) and Type II (reversible) [3]. However, this distinction is not always mutually exclusive- type II dysfunction may not always be reversible and type I may reverse with cessation of the offending agent and heart failure (HF) therapy. Also, both types may co-exist, especially in patients receiving more than one cardiotoxic drug. Differences between type I and II cardiomyopathy are described in Table 13.2.
Table 13.2
Differences between Type I and Type II chemotherapy induced cardiomyopathy
Type I | Type II |
---|---|
Myocardial cell death | Myocardial cell dysfunction |
Reversible | Non-reversible |
Related to cumulative dose | Not related to cumulative dose |
Agent cannot be reintroduced without risk | Agent can be reintroduced with minimal risk |
Biopsy shows myocardial damage | Minimal changes on biopsy |
Drugs like anthracyclines, alkylating agents, microtubule inhibitors | Drugs like trastuzumab, angiogenesis inhibitors, pyrimidine analogues, tyrosine kinase inhibitors |
Definition
The definition of chemotherapy-induced cardiomyopathy has not been precisely defined. Various trials have employed different definitions of this entity. The Cardiac Review and Evaluation Committee (CRCE) has established the following criterion to diagnose chemotherapy induced cardiac dysfunction [4]:
- 1.
Decrease in left ventricular ejection fraction (LVEF), which is either global or worse in the septum
- 2.
Symptoms of congestive heart failure
- 3.
Signs of congestive heart failure, including but not limited to, S3 gallop, tachycardia or both
- 4.
A symptomatic decline in LVEF of at least 5 % or asymptomatic decline in LVEF of at least 10 % to an absolute LVEF of <55 %
Anthracyclines
Anthracyclines still remain the cornerstone of many modern chemotherapeutic regimens, with doxorubicin being the most widely used agent. These agents intercalate between specific bases in the DNA and inhibit DNA and RNA synthesis. Cardiotoxicity with these agents was recognized very early and found to be dose-dependent [5]. Initial studies, performed in 1970s, recommended a maximum total doxorubicin dose 550 mg/m2 [6]. However, these studies were limited by lack of evaluation for asymptomatic LV dysfunction. Recent studies report incidence of LV dysfunction to be as high as 50 % with long-term follow up [7]. Swain et al. [8] reported incidence of cardiomyopathy at 5 %, 16 % and 26 % respectively, with cumulative doses of 400, 500 and 550 mg/m2. Hence, currently the cumulative dose is usually limited to 400–450 mg/m2. However, there is no safe dose and cardiomyopathy has been reported with doses as low as 250 mg/m2, especially in children [9].
Three different manifestations of anthracycline-mediated cardiotoxicity have been described [10]:
- 1.
Acute — Acute cardiotoxicity is rare, occurring in <1 % patients and manifests within hours to weeks as arrhythmias, acute heart failure, myocardial ischemia, or pericarditis/myocarditis-like syndrome. Most patients recover completely from this acute cardiotoxicity. However, long terms effects are not known.
- 2.
Early-onset chronic — This manifestation occurs within the 1st year of treatment and incidence is reported at 1.6–2.1 %. It typically presents as dilated cardiomyopathy and may persist, or even progress, after discontinuation of the offending agent.
- 3.
Late-onset chronic — This is the most common form and is seen in about 1.6–5 % patients. It typically occurs years after chemotherapy and presents as progressive dilated cardiomyopathy, heart failure, or arrhythmias.
Various risk factors have been described for the development of anthracycline-mediated cardiomyopathy (Table 13.3) [11]. The most important risk factor is the total cumulative dose. Other major risk factors include extremes of age, pre-existing cardiovascular disease, concomitant use of other cardiotoxic drugs and chest irradiation.
Table 13.3
Risk factors for anthracycline-mediated cardiomyopathy
1. Cumulative anthracycline dose |
2. Mode of administration (rate of infusion, type of agent, individual dose) |
3. Age — Children <15 years and elderly >70 years |
4. Chest irradiation |
5. Pre-existing cardiovascular disease |
6. Hypertension |
7. Use of other cardiotoxic drugs (e.g., trastuzumab, cyclophosphamide, paclitaxel) |
8. Female sex |
9. Trisomy 21 |
10. HFE gene mutation |
Anthracyclines produce progressive and irreversible type I cardiomyopathy. Cardiac biopsy typically shows patchy areas of interstitial fibrosis, vacuolation and rarely frank necrosis [12]. The pathophysiology of anthracycline-medicated cardiomyopathy is not clearly understood and multiple mechanisms have been hypothesized [13]. The most commonly accepted hypothesis is generation of excessive reactive oxygen species (ROS) and free radical induced myocyte damage. Various mechanisms have been hypothesized for excess free radical production, including mitochondrial dysfunction, increased endothelial nitric oxide synthase production, iron dependent redox cycling, and NAD(P)H dependent mechanisms [13]. However, recent literature suggests that the ROS hypothesis may not fully explain the anthracycline-induced cardiotoxicity [13]. Other hypothesized mechanisms include inhibition of topoisomerase II, DNA cross-linking, decreased ATP production, direct damage to the mitochondria and cell membranes, and increased apoptosis.
Several attempts have been made to reduce doxorubicin cardiotoxicity:
- 1.
Structural modification of anthracyclines —
Many studies have focused on the development of chemotherapeutic agents, with reduced cardiotoxicty, while preserving antitumor efficacy. Unfortunately, thus far, the development of such an agent has remained unsuccessful. Epirubicin drew initial interest due to its reduced cardiotoxicity when compared mg-for-mg with doxorubicin. However, subsequent studies demonstrated epirubicin as a less potent chemotherapeutic agent compared to doxorubicin with similar cardiotoxicity at functionally equivalent doses of epirubicin [14].
- 2.
Different vehicle —
A liposomal-encapsulated doxorubicin remains restricted to the circulating blood and does not cross capillary junctions into normal organs. However, it easily penetrates into the tumor due to increased capillary permeability. It has been shown to reduce cardiotoxicity while still retaining its anti-neoplastic effects [15]. However, more data is still required and increased cost limits its use.
- 3.
Different protocols —
Continuous infusion reduces the risk of cardiotoxicity by decreasing the peak level of the drug [16]. However, it is associated with increased cost, need for an indwelling catheter and increased inconvenience to the patient. There is also a concern for reduction in antineoplastic effects.
Trastuzumab
Trastuzumab is a monoclonal antibody active against human epidermal growth factor (HER2) receptor, which is overexpressed in 25 % of breast cancers. However, trastuzumab significantly increases the risk of cardiomyopathy. A meta-analysis of randomized clinical trials with use of trastuzumab as an adjuvant chemotherapeutic agent showed a 1.6 % absolute increase in incidence of symptomatic heart failure and 7.2 % increase in LV systolic dysfunction [17]. Another trial in patients with metastatic breast cancer showed a 19 % increased risk of cardiomyopathy when trastuzumab was used in combination with anthracycline and cyclophosphamide, and a 12 % increased risk when added to paclitaxel [18]. Analysis of the SEER-Medicare database showed a 32.1 % incidence of cardiomyopathy in patients receiving trastuzumab and a 41.9 % incidence in patients receiving anthracycline plus trastuzumab, which is much higher than previously reported trials [19]. Most protocols recommend use of trastuzumab sequentially with anthracyclines. However, even sequential use is associated with an increased risk of cardiomyopathy, though much less than concomitant use [20] (See Table 13.4).
Table 13.4
Risk factors for development of trastuzumab-induced cardiomyopathy
1. Age> 50 years |
2. Hypertension |
3. Concomitant use of anthracyclines or paclitaxel |
4. Pre-existing cardiovascular disease |
5. Smoking |
Trastuzumab produces type II cardiomyopathy, which is not dose dependent and potentially reversible. There is no visible myocyte damage on histology and changes are visible only on electron microscopy [21]. Some authors have re-challenged patients with trastuzumab without recurrence of cardiomyopathy in most patients [4]. However, many authors have questioned its reversible nature and reported a 20–40 % incidence of persistent LV dysfunction [22]. MRI studies have shown evidence of delayed gadolinium enhancement despite recovery of cardiac function, suggesting persistent myocardial damage [23]. Long-term studies are needed to better define the natural history of trastuzumab-induced cardiomyopathy.
The mechanism of trastuzumab-induced cardiomyopathy is not well understood, but inhibition of ErbB2 is thought to be the main mechanism. ErbB2 is a critical component of multiple anti-apoptotic pathways and is necessary for myocyte survival and repair. Trastuzumab binds to the ErbB2 on cardiac myocytes and blocks the cardioprotective ErbB2-ErbB4 signaling pathway [24]. Removal of trastuzumab leads of resumption of these pathways and recovery of cardiac damage. The synergistic cardiotoxic effects of anthracycline and trastuzumab can be explained by myocyte damage due to anthracyclines and blockage of repair mechanisms by trastuzumab [25].
A few approaches to reduce trastuzumab-induced cardiotoxicity are under investigation:
- 1.
Second generation monoclonal antibodies —
Lapatinib, a HER1 and HER2 receptor inhibitor, appears to be associated with much lower risk of cardiomyopathy than trastuzumab [26]. Other second-generation anti-HER2 therapies are being developed and three agents – neratinib, trastuzumab-DM1 and pertuzumab, are currently under investigation.
- 2.
Anthracycline-sparing protocols —
The BCIRG 006 trial demonstrated that the anthracycline-sparing chemotherapy protocols with trastuzumab alone were associated with significantly decreased risk of cardiomyopathy while preserving antitumor efficacy [27]. However, data regarding anti-neoplastic efficacy without anthracyclines is still conflicting [28].
- 3.
Shorter treatment duration —
Preliminary data shows that shorter regimens of trastuzumab are associated with a lower incidence of cardiomyopathy [29]. However, larger long-term studies are required to assess cardiotoxicity and anti-tumor efficacy.
Alkylating Agents
Cyclophosphamide is activated in the liver and its active metabolite crosslinks DNA, disrupting cell division. It has been associated with increased risk of cardiomyopathy, especially in combination with an anthracycline or cisplatin [30]. Other risk factors include advanced age and mediastinal irradiation. The mechanism of cardiotoxicity is not very well established and appears to be related to the strength of the individual dose, rather than cumulative dose [30]. Ifosamide has also been rarely associated with cardiomyopathy [31].
Antimicrotubule Agents
Paclitaxel, alone, has not been implicated as a cause of cardiomyopathy. However, it reduces the elimination of doxorubicin and increases its toxicity [32]. Paclitaxel should be avoided immediately before doxorubicin administration and within the next hour. The most common cardiovascular side-effect of paclitaxel is transient asymptomatic bradycardia [33].
Flouropyramidines
5-Flourouracil (5-FU) and its oral prodrug capecitabine have been associated with cardiotoxicity. The most common cardiac side effect is myocardial ischemia, likely related to coronary vasospasm [34]. Cardiomyopathy from these agents is rare and limited to a few case reports. It appears to have a type II pattern, with recovery of cardiac function in most cases after cessation of the agent [35].
Angiogenesis Inhibitors
Bevacizumab is a recombinant monoclonal antibody against the vascular endothelial growth factor (VEGF) receptor. It has primarily been associated with an increased risk of thrombotic events. The risk of cardiomyopathy is very low and follows the reversible type II pattern [36]. The most probable hypothesis is the loss of protective effects of VEGF against endothelial dysfunction caused by excess oxidative stress.
Tyrosine Kinase Inhibitors
The most common cardiovascular effect of tyrosine kinase inhibitors is hypertension. Sunitinib has been associated with a 6–8 % incidence of HF and up to a 19 % incidence of cardiomyopathy, especially in patients with pre-existing coronary artery disease and cardiac risk factors, like hypertension [37]. Imatinib, an inhibitor of the Bcr-Abl protein, is used in the treatment of chronic myelogenous leukemia and has been associated with HF as well [38]. It is hypothesized to induce cardiotoxicity by the activation of the endoplasmic reticulum stress response pathways. Electron microscopy of cardiac biopsies demonstrates membrane whorls, pleomorphic mitochondria, effaced cristae, glycogen accumulation, lipid droplets, and vacuoles [38]. However, data is limited to animal studies and a few small case series.
Diagnosis
Traditionally, the detection of cardiotoxicity has relied on detection of reduction in LVEF. Multiple-gated acquisition (MUGA) scan and transthoracic echocardiography are the two most commonly used modalities. Baseline LVEF should always be obtained before initiation of chemotherapy. The values obtained from different modalities are not interchangeable and, hence, the same modality should continually be used throughout the chemotherapy protocol for an assessment and comparison of LVEF.
MUGA Scan
Historically, MUGA scan has been the modality of choice to assess LVEF prior recent advances in echocardiography. MUGA scan has the advantage of lower inter-observer variability and generation of an exact LVEF number [39]. However, it exposes the patients to ionizing radiation (approximately 7.8 mSv/exam) [40] and does not provide any information regarding valvular disorders, pericardial diseases, and diastolic parameters. Currently, most centers use echocardiography as the primary modality for LVEF assessment. MUGA scan continues to be used at many centers due to the oncologists’ familiarity with the test and interpretation of its results. MUGA scan use should be limited in patients with poor acoustic windows or with pre-existing cardiomyopathy prior to the initiation of chemotherapy.
Transthoracic Echocardiography
Transthoracic echocardiography is the most commonly used modality for assessment of LVEF in patients receiving chemotherapy. Echocardiography is readily accessible, portable, inexpensive, and does not expose patients to ionizing radiation. It also provides additional information regarding valvular disorders, diastolic dysfunction, and pericardial disorders. Its disadvantages include larger inter-observer variability, dependence on complex geometric models and lack of good acoustic windows in many patients. Inter-observer variability and need for assumptions regarding ventricular geometry can be reduced by using 3D transthoracic echocardiography [41]. A small study with real time 3D transthoracic echocardiogram showed good correlation with MRI and MUGA scans in patients receiving chemotherapy [23]. Poor acoustic windows can be improved by the use of contrast agents, which better delineate the endocardial borders and reduce intra- and inter-observer variability [42]. Ideally, 3D echocardiography or contrast-enhanced echocardiography should be used as the modality of choice in screening patients for chemotherapy-induced cardiomyopathy.
Multiple indices of diastolic function have been evaluated in patients undergoing cardiotoxic chemotherapy [43]. Diastolic dysfunction usually precedes systolic dysfunction and early detection can potentially reduce the future risk of cardiomyopathy. However, none of the diastolic parameters have been found to have any significant association with development of future cardiac dysfunction [44].
Another modality used for early detection of cardiac dysfunction is the assessment of cardiac reserve by exercise or pharmacological stress echocardiography. Small studies have shown that reduction in cardiac reserve can be seen as early as after first cycle of chemotherapy and it can predict future cardiovascular events [45]. However, data regarding use of stress echocardiography are very limited.
The newest development in echocardiography is speckled and strain imaging. Multiple small trials have shown that these modalities can detect systolic dysfunction earlier than standard parameters and predict long term development of cardiomyopathy [46, 47]. Larger trials are still required before these modalities become standard of care. The major concern with these highly sensitive techniques is that many patients may never develop clinically significant cardiac dysfunction and life-saving therapies may be withheld unnecessarily.
Endomyocardial Biopsy
An endomyocardial biopsy can show typical features of cell damage from agents with type I cardiotoxicity. It was the gold standard to diagnose chemotherapy-induced cardiotoxicity. Billingham et al. [12] performed the pivotal trials assessing the histopathological changes following anthracycline exposure and developed a four point scoring system to characterize the extent of myocardial damage. However, it is an invasive procedure with significant risks and is not routinely used these days, especially with recent advances in other modalities of non-invasive imaging.
Cardiac MRI
Cardiac MRI (CMR) is currently the gold standard for assessment of LVEF [48]. CMR can also identify regions of non-transmural cardiac injury by delayed gadolinium enhancement. Anthracycline-induced cardiomyopathy characteristically demonstrates a delayed enhancement in the anterolateral wall and trastuzumab-induced cardiomyopathy shows a subepicardial delayed enhancement in the lateral wall [23]. CMR also provides detailed structural information, including right-sided chambers. However, CMR is not widely available and is expensive. CMR cannot be used in patients with metal devices or implants and gadolinium use is contraindicated in patients with reduced renal function.
Cardiac Biomarkers
Reduction in LVEF is a late development in the cascade of development of cardiomyopathy and early identification is critical. Several cardiac biomarkers have been studied to identify early cardiac damage. However, utmost caution must be exercised as a false positive result can withhold lifesaving therapy. A good biomarker must be easy to measure, accurate, reproducible, and most importantly, should have high specificity to limit likelihood of false positive results. Biomarkers should be used as an adjunct to the previously described modalities of cardiac assessment [49].
Troponin I is the most studied biomarker as a predictor of development of cardiomyopathy. Elevation of troponin I levels can predict cardiac damage earlier than currently used modalities [50]. One study involving 703 patients receiving anthracyclines showed a 30 % incidence of troponin I elevation and 9 % patients had persistent elevation even at 1 month [51]. Biomarker measurements were done before starting chemotherapy and immediately after. The testing was repeated at 12, 24, 36 and 72 h and again at 1 month. Cardiovascular endpoints were seen in 1 %, 37 % and 84 % patients, respectively, in troponin I negative, transient positive and persistent troponin I positive patients at 1 month. The positive and negative predictive value of troponin I was 84 % and 99 %, respectively. However, this has not been validated in repeated larger trials. A consensus regarding optimal timing of troponin I measurement has also not been reached.
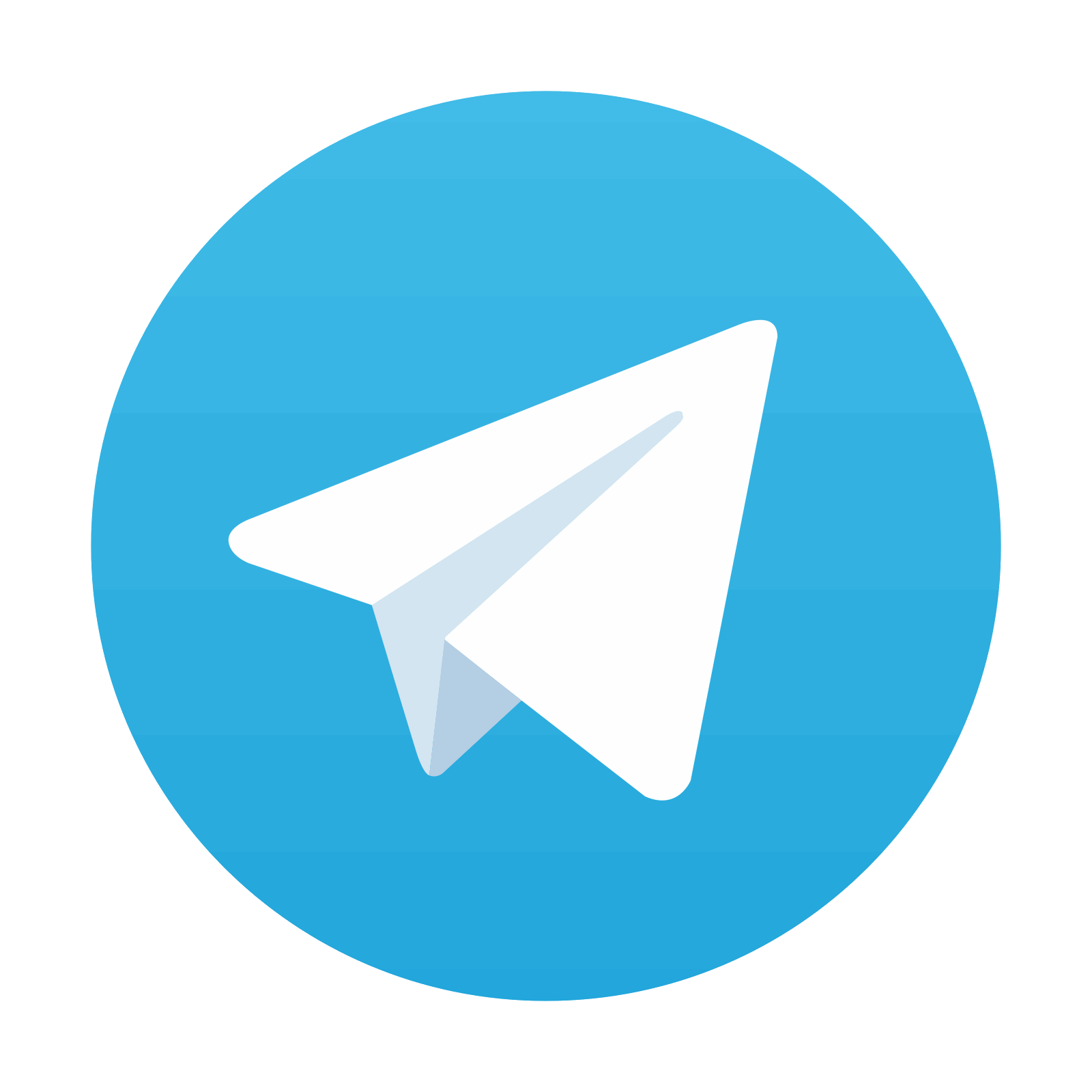
Stay updated, free articles. Join our Telegram channel

Full access? Get Clinical Tree
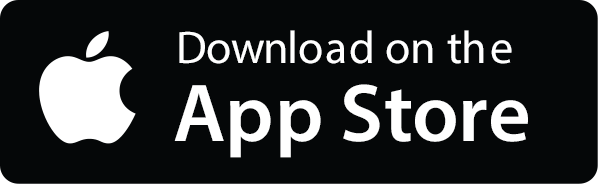
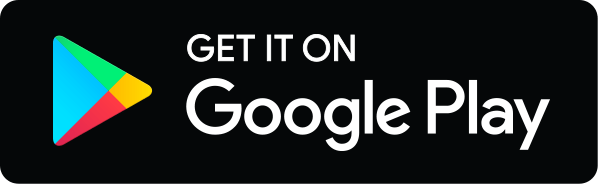