Longitudinal relaxation corresponds to the recovery of the magnetization along z direction and is defined by an exponential time constant, T1. Water molecules have long T1 values and appear dark on T1-weighted images whereas fat has a short T1 value and will appear bright.
Transverse relaxation refers to how quickly the spins exchange energy in the xy direction. This loss of transverse magnetization follows an oscillating pattern referred to as the free-induction decay and follows a time constant: T2. Local inhomogeneities in the magnetic field further accelerate this process and lead to a faster decay called T2*. Typically, water molecules have long T2 values and appear bright on T2-weighted images that can be useful in detecting active inflammation, for example.
The main magnet coils generate a strong constant, yet inhomogeneous, magnetic field (B0). Typically, the field strength of cardiac MRI scanners ranges from 1.5 to 3 Tesla.
When turned on, the transmitter coils that are built in the main structure of the magnet can emit an RF (weaker magnetic field) that will excite protons.
Three gradient coils built in the main structure of the magnet along the x, y, and z directions can be turned on and off. Gradients create small variations in magnetic field along their axis and are useful for slice selection and localizing the magnetic resonance signal in space.
Receiving coils are typically placed on the surface of the patient’s body to maximize signal coming from the heart and are turned on during signal readout.
seen in infarction, infiltration, replacement fibrosis, and also inflammation/edema.
TABLE 37.1 Clinical Indications for Cardiac Magnetic Resonance (CMR) | ||||||||||
---|---|---|---|---|---|---|---|---|---|---|
|
with myocardial injury, but with nonobstructive coronary arteries (MI with no obstructive arteries [MINOCA]). By providing multicomponent assessment of myocardial structure and physiology, CMR can effectively capture various noncoronary abnormalities and guide differential diagnosis.
![]() FIGURE 37.2 Short-axis phase-sensitive inversion recovery image obtained 15 min after gadolinium administration in a patient with a recent anteroseptal MI. Within the 100% transmural LGE in the anteroseptum, an area of MVO is clearly seen. LGE, late gadolinium enhancement; MI, myocardial infarction; MVO, microvascular obstruction. (Reprinted by permission from Springer: Kramer CM, Salerno M. Acute Myocardial Infarction and Postinfarction Remodeling. In: Kwong R, Jerosch-Herold M, Heydari B, eds. Cardiovascular Magnetic Resonance Imaging. Contemporary Cardiology. New York, NY: Springer; 2019: 161-174. Copyright © 2019 Springer Science+Business Media, LLC.) |
![]() FIGURE 37.3 A 62-year-old male is referred to pharmacologic stress CMR using regadenoson to assess for coronary artery disease in the context of newly discovered hypokinesis of the inferolateral wall on echocardiogram. The study revealed a moderate size first-pass perfusion defect involving the basal inferior septum, basal inferior, mid inferior, and apical inferior segments. These areas were partially matched by subendocardial to transmural late gadolinium enhancement raising concerns for prior myocardial infarction in the distribution of the posterior descending coronary artery with significant peri-infarction ischemia. CMR, Cardiac magnetic resonance imaging; LGE, late gadolinium enhancement. |
There is excellent correlation of stress CMR assessment of ischemia against invasive measurement of fractional flow reserve, showcasing its high accuracy in determining the physiologic significance of coronary stenosis, and safely guiding management in IHD.13 Compared to cardiac single photon emission computed tomography (SPECT) imaging, stress CMR has several technical advantages: (1) it is not limited by attenuation artifacts; (2) it is free from ionizing radiation; (3) it has three- to fourfold higher spatial resolution; (4) it takes 35 to 45 minutes to complete (compared to >2 hours for dual-isotope SPECT); and (5) it performs better than SPECT in detecting single or multivessel coronary disease.14,15,16
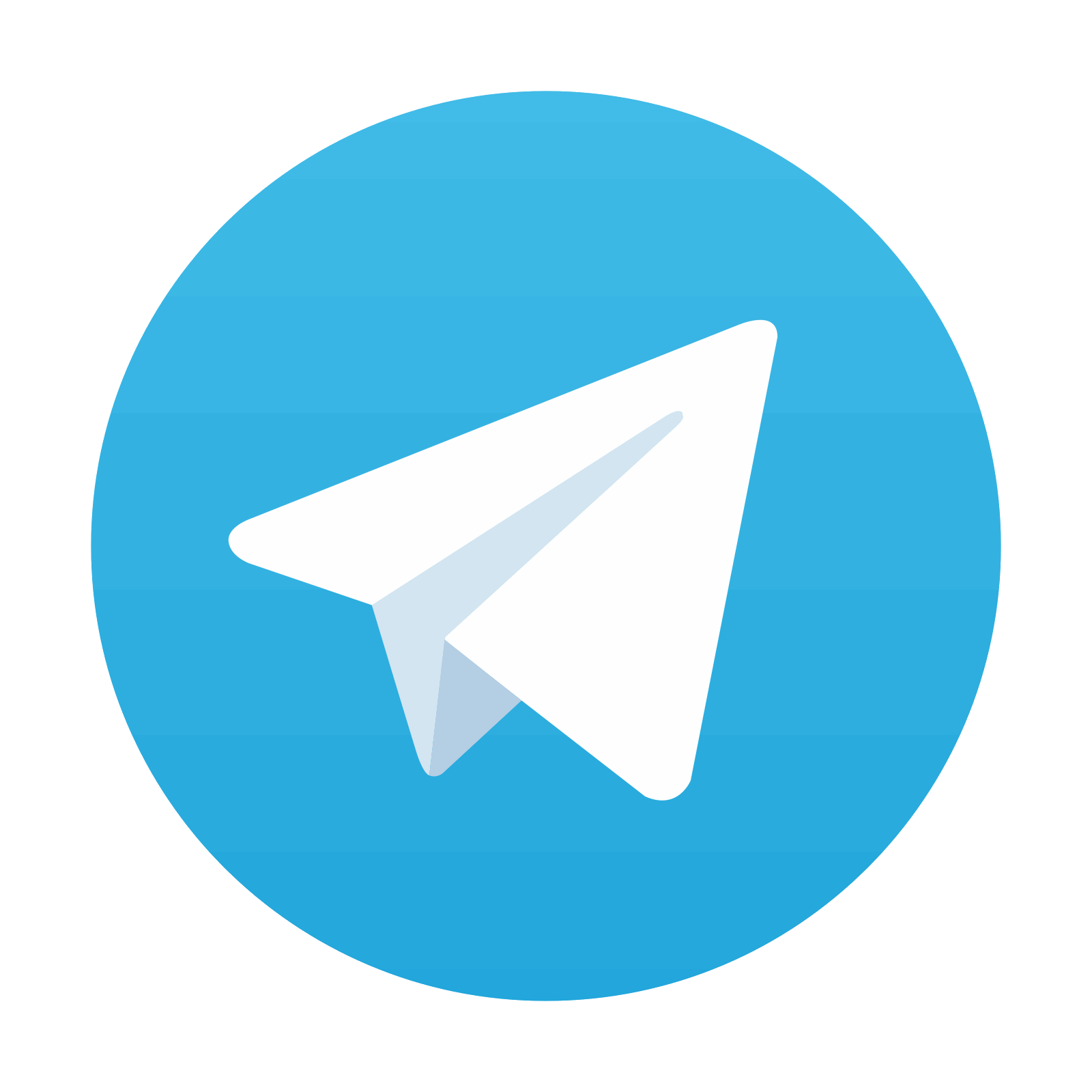
Stay updated, free articles. Join our Telegram channel

Full access? Get Clinical Tree
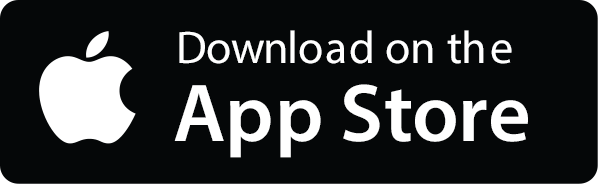
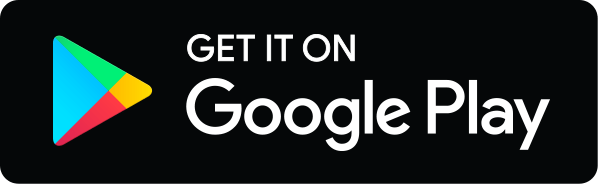
