Cardiac magnetic resonance (CMR) imaging has become a powerful diagnostic tool in the assessment of cardiomyopathy. CMR not only provides a comprehensive evaluation of structure and function, but also allows for tissue characterization. CMR is the gold standard for quantification of cardiac volumes and function; it allows 3-dimensional visualization of the heart, as well as detailed flow evaluation with velocity encoded cine imaging. Tissue characterization has become the most exciting aspect of CMR in the field of heart failure (HF). The use of late gadolinium enhancement (LGE) allows for direct visualization of focal ischemic and nonischemic scar. Advances in T1 mapping now enable quantification of diffuse myocardial fibrosis by measuring the extent of interstitial expansion via extracellular volume (ECV) fraction. Further tissue characterization is performed with T2 mapping allowing for visualization of myocardial edema, while T2 star mapping directly quantifies myocardial iron content. The comprehensive nature of CMR not only yields high-quality diagnostic data, but also significant prognostic information, especially in patients with HF. This chapter will highlight the critical role of CMR in the assessment of cardiomyopathies.
A 50-year-old woman with a history of coronary artery disease (CAD) and coronary artery bypass grafting, prior myocardial infarction (MI), and ischemic cardiomyopathy presented with dyspnea and decompensated systolic HF. Her bypass grafts were found to be occluded, including her left internal mammary artery, and she was being evaluated for possible repeat bypass surgery. CMR was ordered to assess myocardial viability.
Assessment of cardiac function with electrocardiogram (ECG)-gated steady-state free precession (SSFP) cine imaging, a key element of the standard CMR cardiomyopathy protocol, demonstrated multiple regional wall motion abnormalities (Figure 23-1) and moderate to severe left ventricular (LV) systolic dysfunction. LGE imaging showed near transmural MI of the basal to mid inferolateral wall and the entire apex (Figure 23-2), consistent with nonviable myocardium. Subendocardial infarction involving 50% of myocardial wall thickness was present in the anteroseptal, anterior, and anterolateral segments (Figure 23-3), suggesting partial viability. Given multiple segments with limited to no viability, high risk of redo sternotomy, and concern for medication noncompliance with dual antiplatelet therapy, the patient was managed with aggressive goal-directed medical therapy.
In acute MI, damaged myocyte membranes allow gadolinium to diffuse into the intracellular space, resulting in increased tissue concentration of gadolinium compared with normal myocardium. Likewise, in chronic infarction, as necrotic tissue is replaced by collagenous scar, the interstitial space expands, which leads to increased gadolinium tissue concentration. Higher tissue concentrations of gadolinium shorten the T1 relaxation time and thus appear bright, or hyperenhanced, whereas viable regions appear black, or nulled.1 In patients with ischemic cardiomyopathy, the transmural extent of infarction predicts improvement in contractile function, with 51% to 75% transmural extent associated with limited viability and 76% to 100% associated with virtually no viability.2,3 The high spatial resolution of CMR allows for a more accurate determination of the transmural extent of viability as opposed to other imaging methods. This distinction is critical because it has been repeatedly shown that revascularization of patients with viable myocardium leads to significant improvement in clinical outcome.3
A 55-year-old man with a history of diabetes mellitus type 2 and hypertension (HTN) presented to the emergency department with 4 days of chest pain associated with nausea. His ECG showed anterior and lateral ST elevations, for which he was emergently taken to the cardiac catheterization laboratory. Angiographic imaging demonstrated 100% occlusion of his mid left anterior descending artery (LAD), at which time he received a bare metal stent with restoration of TIMI-3 flow (Figure 23-4). Subsequent echocardiogram showed moderate, segmental LV dysfunction with an ejection fraction (EF) of 35% to 40% (Figure 23-5) and an apical LV thrombus (Figure 23-6), for which he was placed on warfarin. Six months after his MI, he was enrolled in a research trial and received intracoronary stem cell therapy. He was referred for CMR imaging as part of the research protocol.
Cine SSFP imaging (Figure 23-7) demonstrated persistent anterior and anteroseptal akinesis with moderate LV systolic dysfunction. LGE imaging (Figure 23-8) demonstrated transmural scar of the apical anterior, septal, and inferior walls, and the true apex, along with near transmural extension of the infarct into the distal portion of the mid anteroseptum and inferoseptum, consistent with prior LAD-territory infarction. CMR confirmed a large residual thrombus in the LV apex (Figure 23-8).
In a large cohort of 738 ST elevation myocardial infarction (STEMI) patients, the prevalence of LV thrombus as assessed by CMR was 3.5% and increased to 7.1% for anterior STEMIs. The presence of an LV thrombus was associated with larger infarct size, lesser myocardial salvage, and a lower EF. Moreover, LV thrombus was independently associated with major adverse cardiac events at 1 year.4 CMR is superior to transthoracic echocardiography for the detection of LV thrombus, with reported sensitivities of 88% and 33% to 40%, respectively.5 On LGE imaging, thrombus appears as a low-signal-intensity mass surrounded by high-signal-intensity structures including the ventricular cavity and surrounding myocardium. The absence of contrast enhancement can be used to distinguish thrombus from other masses such as neoplasm, which typically demonstrate contrast uptake due to tumor-associated vascularity. LGE imaging can be further tailored for thrombus assessment by prolonging the inversion time, thus exploiting the avascular tissue properties of the thrombus. At prolonged inversion times, even severely damaged myocardium with microvascular obstruction exhibits some degree of contrast uptake, whereas the thrombus exhibits none and appears black.6 Delayed enhancement CMR is thus highly superior to anatomic cine imaging at identifying the presence of even small thrombi.
A 42-year-old man with no prior medical history presented with 3 months of dyspnea, orthopnea, paroxysmal nocturnal dyspnea (PND), and lower extremity edema. He was initially treated with inhalers for an upper respiratory infection, but his symptoms did not improve. Chest radiography demonstrated cardiomegaly, for which he was referred to cardiology, and subsequent transthoracic echocardiography (TTE) revealed an EF of 5%. Resting nuclear perfusion images demonstrated an extensive area of decreased perfusion within the inferoposterior and inferolateral segments from base to apex but no evidence of ischemia. Cardiac catheterization showed normal coronary arteries. He was referred for CMR to evaluate his cardiomyopathy.
Cine SSFP imaging confirmed severe biventricular dilatation and systolic dysfunction (Figure 23-9). LGE imaging demonstrated striking, linear midmyocardial fibrosis in the septum of nonischemic etiology (Figure 23-10). There was no CMR evidence of prior MI. The ECV fraction was significantly elevated at 35% (normal <29%) signifying diffuse interstitial expansion. With goal-directed medical therapy, his EF by echocardiography improved to 30%. He declined cardioverter defibrillator implantation at that time due to concern that it would interfere with his occupation as an arc welder. Following 6 months of medical therapy, his EF had improved to 48% by multigated acquisition (MUGA) scan (Figure 23-11).
CMR imaging allows the differentiation of ischemic and nonischemic cardiomyopathy based on the location and pattern of LGE; and further delineates nonischemic cardiomyopathies based on morphology, scar, and novel tissue characterization techniques (Figure 23-12).7,8 Midmyocardial fibrosis as noted by LGE has been shown to be an independent and incremental predictor of ventricular arrhythmias, mortality, and morbidity in patients with nonischemic dilated cardiomyopathy.9 This and numerous other studies have found that the presence of LGE was the best predictor of sudden cardiac death in dilated cardiomyopathy patients, and ultimately can aid in selecting ideal candidates for defibrillator implantation.
LGE imaging is an accurate method to measure focal myocardial replacement fibrosis; however, it cannot assess diffuse interstitial fibrosis as image contrast relies on the different signal intensities between fibrotic and normal myocardium.10 In diffuse nonischemic processes these differences may not exist. Novel T1 mapping can now quantify diffuse myocardial fibrosis with measurement of ECV fraction, which has been shown to highly correlate with the histologic extent of collagen.11 An elevated ECV is known to portend worse outcomes in multiple disease states, including the nonischemic patient population and has the ability to detect the ameliorating effects of renin angiotensin aldosterone system inhibition.12 Specifically in patients with dilated cardiomyopathy, ECV measurements reflect myocardial collagen content, and can serve as a noninvasive tool for quantifying myocardial fibrosis to aid in risk stratification and to monitor response to therapy.13
A 59-year-old woman with a history of Laing skeletal myopathy presented for cardiac evaluation. She began experiencing falls in her 30s, which led to genetic testing and demonstration of a myosin heavy chain mutation (MYH7). She was identified as the proband of a large autosomal-dominant family pedigree that was diagnosed with the MYH7 mutation. She experienced an episode of chest pain 8 years prior to her current presentation and reportedly had abnormal cardiac biomarkers at that time, but her workup was otherwise unremarkable. On presentation, she complained of chest pain with emotional stress and with exertion. She was referred for CMR to evaluate for myocardial involvement of her skeletal myopathy.
Cine imaging (Figures 23-13 and 23-14) demonstrated heavily trabeculated left and right ventricles, meeting diagnostic imaging criteria for noncompaction. Biventricular systolic function was normal. She had no fibrosis on LGE imaging and no evidence of LV thrombus at long inversion times. Her chest pain has improved with long-acting nitrate therapy.
Left ventricular noncompaction cardiomyopathy (LVNC) is a rare abnormality of the LV wall resulting from developmental arrest of the normal compaction process of the myocardium leading to the formation of 2 layers of the myocardium: the compacted and the noncompacted layer. The spectrum of myocardial phenotypes is broad and can present as dilated, hypertrophy, mixed, restrictive, or arrhythmogenic cardiomyopathy.14 Multiple genes have been implicated in LVNC, which can be familial or sporadic, isolated or associated with other cardiomyopathies, congenital heart disease, mitochondrial diseases, or complex multiorgan syndromes. The primary phenotype can result in progressive cardiac dysfunction and be associated with dysrhythmias or thromboembolic events.
CMR is uniquely suited for evaluating LVNC as it can easily identify the noncompacted layer predominantly located in the apical and lateral segments. A noncompacted to compacted layer ratio of >2.3 in diastole yields a high diagnostic accuracy for distinguishing pathologic LVNC.15 Given the diverse phenotypic presentation in LVNC, numerous LGE patterns have been described including subendocardial, trabecular, midmyocardial, and transmural.16 The presence of myocardial fibrosis itself has been shown to relate to clinical disease severity and LV systolic dysfunction. In addition, CMR can better visualize right ventricular (RV) involvement as well as identify apical thrombi.
A 62-year-old woman with ER/PR+ metastatic breast cancer presented 16 years after her diagnosis with supraventricular tachycardia. Subsequent echocardiogram showed new biventricular systolic dysfunction with an LVEF of 25% to 30%. Her oncologic history was notable for full treatment with cyclophosphamide, doxorubicin, and 5-fluorouracil with subsequent tamoxifen therapy after her initial diagnosis. Her disease progressed 10 years after her diagnosis, and she was subsequently treated with letrozole followed by docetaxel and later fulvestrant with additional thoracic radiation. She developed leptomeningeal involvement, for which she received capecitabine and intrathecal cytarabine, and had recently been started on exemestane and everolimus at the time of her cardiac presentation. She was referred for CMR to evaluate her cardiomyopathy.
SSFP cine imaging demonstrated LV dilatation and severe LV systolic dysfunction (Figure 23-15). LGE imaging revealed the presence of midmyocardial fibrosis within the septum, consistent with an underlying nonischemic myopathic process (Figure 23-16). She had no evidence of myocardial inflammation, fatty infiltration, or iron overload. Her native T1 time was elevated at 1082 ms. Her cardiomyopathy was presumed to be secondary to her intensive and prolonged chemotherapy regimens. She was placed on goal-directed medical therapy with an improvement in her EF to 39%. She developed angioedema with lisinopril and did not tolerate long-acting nitrate therapy due to headaches; thus, she remains on beta blocker and hydralazine therapies and has demonstrated sustained improvement in LV systolic function.
Autopsy data have shown that myocardial fibrosis is a key feature of patients with late anthracycline-induced cardiomyopathy,17 which CMR imaging is best suited to detect. CMR imaging also adds diagnostic value for its accurate assessment of LVEF, proving to be a more precise tool than echocardiography for screening this at-risk population.18
However, early detection of chemotherapy-induced cardiotoxicity is also critically important. Current surveillance strategies rely on changes in LV systolic function to identify cardiotoxicity, a feature that is now thought to be a late finding.19 Newer diagnostic techniques are emerging that may allow for earlier detection of cardiotoxicity secondary to chemotherapy. Research has shown that patients treated with anthracyclines have higher native T1 times and ECVs as calculated from T1 mapping than controls, even in the absence of LV dysfunction.20,21 In a cohort of cancer patients, changes in T1 signal intensity correlated to small but significant decreases in LVEF just 3 months after the receipt of potentially cardiotoxic chemotherapy, suggesting that T1-weighted signal intensity may serve as an early marker of injury.20 Further research in this area is ongoing. However, the ability of CMR to accurately assess LV systolic function with the added benefit of myocardial tissue characterization may lead to more accurate and more timely diagnosis of this form of cardiomyopathy.
A 58-year-old woman with atrial fibrillation, HTN, stroke, and gastroparesis was previously diagnosed with a nonischemic cardiomyopathy after an unremarkable workup, including negative fat pad, bone marrow, and gastric biopsies. Her mother died from a nonischemic cardiomyopathy at age 50 years. She presented acutely 4 years later with decompensated systolic HF, and a CMR examination was performed.
Breath-held SSFP cine imaging was obtained and demonstrated severe concentric left ventricular hypertrophy (LVH), right ventricular hypertrophy (RVH), biatrial enlargement with wall thickening, a moderate sized pericardial effusion, and bilateral pleural effusions (Figure 23-17). Biventricular systolic function was moderately to severely reduced. LGE imaging demonstrated diffuse hyperenhancement of the myocardium and atrial walls with the inability to null the myocardium (Figures 23-18 and 23-19). Calculated ECV fraction by T1 mapping (Figure 23-20) was 82% (normal <29%).22 Together these findings were diagnostic for classic cardiac amyloidosis. Subsequent right heart catheterization showed elevated filling pressures and endomyocardial biopsy demonstrated positive Congo red staining, and immunohistochemical staining confirmed transthyretin-related amyloid (ATTR) deposition within the heart. No amino acid sequence abnormalities in the transthyretin protein were detected, making wild-type ATTR amyloidosis the most likely etiology. Of note, urine and serum protein electrophoreses were normal.
ATTR amyloidosis is a fatal disorder characterized primarily by progressive neuropathy and cardiomyopathy. Mutant (with autosomal-dominant inheritance) and wild-type (with predominant cardiac involvement) forms exist.23 The prognosis of wild-type ATTR is better than other forms of amyloid cardiomyopathy, with median survival of 60 months from presentation with HF symptoms versus only 5.4 months in patients with cardiomyopathy from light chain (AL) amyloidosis.24 In a large series of more than 100 patients with wild-type ATTR, median survival from onset of symptoms was 6.07 years.25 Our patient survived 4 years after her initial presentation with HF.
CMR is uniquely suited for the diagnosis of cardiac amyloidosis. In this disease, insoluble fibrillar amyloid protein deposits within the myocardial interstitium. CMR T1 mapping allows for quantification of extracellular interstitial matrix expansion via the measurement of ECV fraction.26,27 ECV is uniformly and uniquely elevated in cardiac amyloidosis28 and provides significant prognostic value.29 Figure 23-27 demonstrates the striking difference between precontrast and postcontrast T1 maps in a patient with cardiac amyloid (panel A and B) and a healthy individual (panel C and D). Additionally, amyloid infiltrative disease is associated with distinct patterns of LGE. Gadolinium-based contrast agents accumulate in extracellular spaces without crossing intact myocardial cell membranes and result in T1 shortening. In cardiac amyloid, there is increased gadolinium contrast in areas of amyloid deposition, resulting in substantial lowering of myocardial T1 and prominent hyperenhancement on LGE imaging. LGE patterns in amyloidosis may be patchy, transmural, or subendocardial.30 Recent literature suggests that the LGE pattern may be able to distinguish between various subtypes of cardiac amyloidosis.31
A 51-year-old man with a known history of hereditary hemochromatosis (HH) was admitted with altered mental status due to severe diabetic ketoacidosis. Genetic testing 10 years earlier confirmed the presence of a C282Y mutation in the HH gene, but he had previously refused treatment. Abdominal imaging on presentation showed iron deposition within the liver, pancreas, and adrenal glands. His hospital course was complicated by paroxysmal atrial fibrillation and nonsustained ventricular tachycardia. He was referred for CMR to assess for cardiac involvement.
T2-star (T2*) imaging demonstrated severe cardiac and hepatic iron overload (Figure 23-21). Both myocardial and hepatic T2* values were <1.8 ms (shortest echo time) (normal >20 ms),32 which precluded precise calculation of tissue iron content. LV systolic function was mildly reduced on cine imaging with no evident fibrosis on LGE imaging. The patient was subsequently started on goal-directed medical therapy and underwent phlebotomy for iron overload. His disease was felt to be too advanced to realize any significant benefit from chelation therapy.
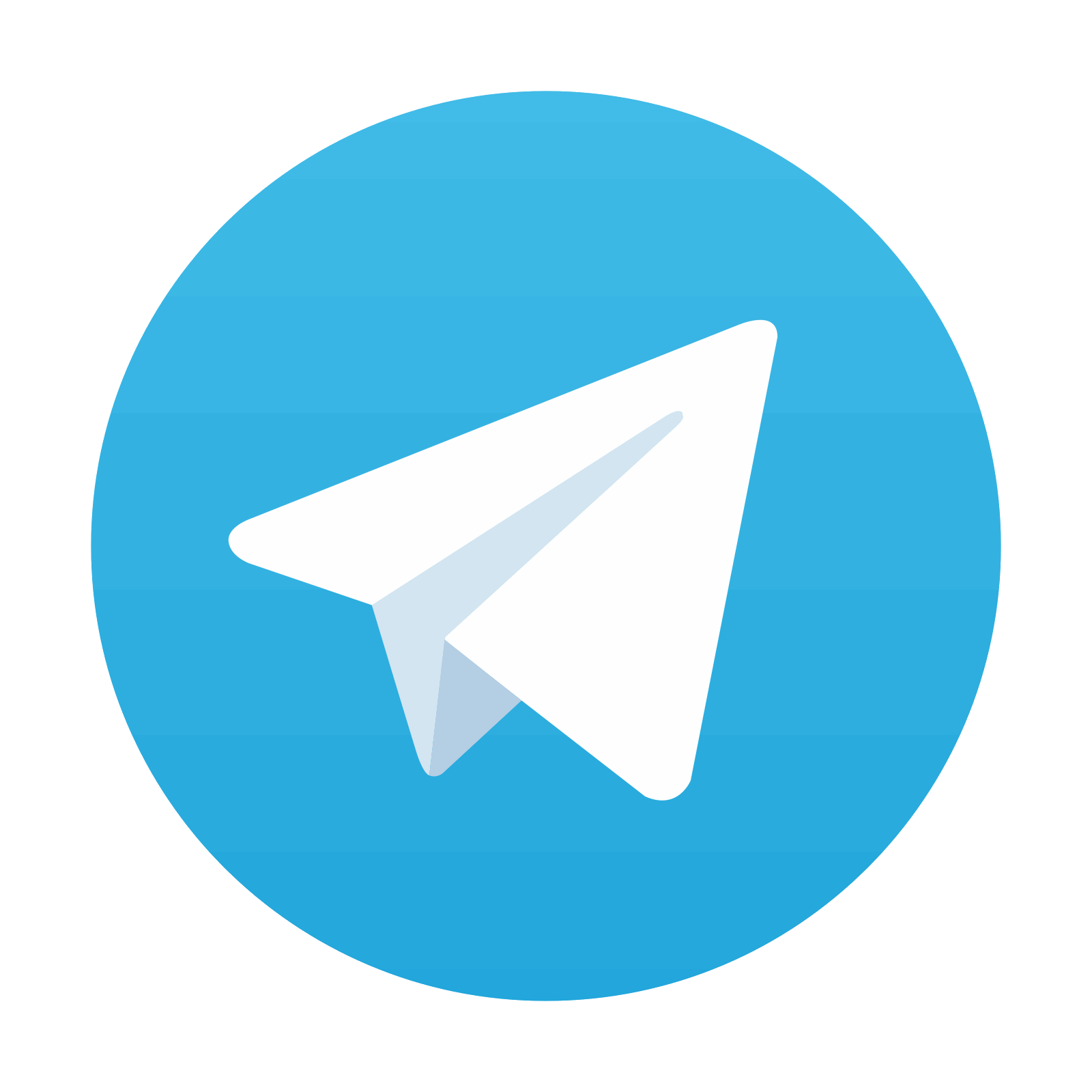
Stay updated, free articles. Join our Telegram channel

Full access? Get Clinical Tree
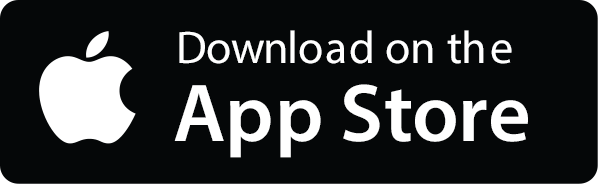
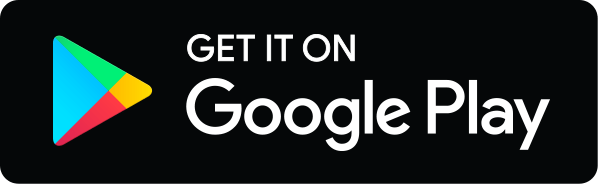